Environmental effects of ozone depletion and its interactions with climate change: progress report, 2015
Received
6th January 2016
, Accepted 6th January 2016
First published on 28th January 2016
Abstract
The Environmental Effects Assessment Panel (EEAP) is one of three Panels that regularly informs the Parties (countries) to the Montreal Protocol on the effects of ozone depletion and the consequences of climate change interactions with respect to human health, animals, plants, biogeochemistry, air quality, and materials. The Panels provide a detailed assessment report every four years. The most recent 2014 Quadrennial Assessment by the EEAP was published as a special issue of seven papers in 2015 (Photochem. Photobiol. Sci., 2015, 14, 1–184). The next Quadrennial Assessment will be published in 2018/2019. In the interim, the EEAP generally produces an annual update or progress report of the relevant scientific findings. The present progress report for 2015 assesses some of the highlights and new insights with regard to the interactive nature of the effects of UV radiation, atmospheric processes, and climate change.
We would like to dedicate this progress report to the memory of Dr David J. Erickson III (20 May, 1960–16 November, 2015), member of the Environmental Effects Assessment Panel since 1994 and contributor to the section on biogeochemical cycles. He was a scientist, colleague, and friend to all the members of the Panel. His expertise and knowledge of issues related to climate change and the effects of UV radiation on chemical processes in the environment will be sorely missed. We will remember Dave for his colourful, larger than life, and humorous character – full of spirit and courage.
1 Ozone and changes in biologically active UV radiation reaching the Earth's surface
1.1 By 2013, the implementation of the Montreal Protocol had already achieved significant benefits for the ozone layer and, consequently, for surface UV-B radiation
Model calculations have shown that, without the Montreal Protocol, a deep Arctic “ozone hole”, with total ozone values of below 120 DU, would have occurred in 2011 given the meteorological conditions in that year (Fig. 1). The decline of stratospheric ozone over the Northern Hemisphere mid-latitudes would have continued, more than doubling to about 15% by 2013 relative to the onset of ozone-depletion.
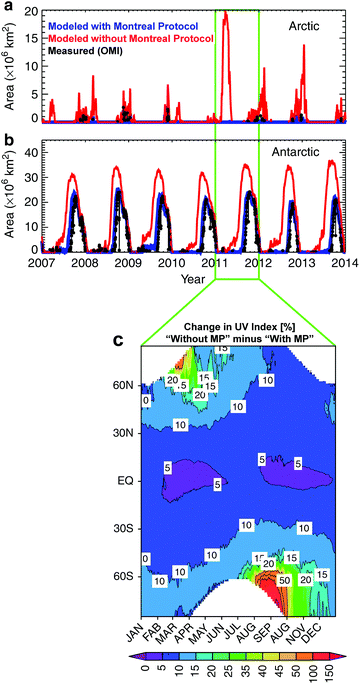 |
| Fig. 1 (a) Without the Montreal Protocol (MP), there would have been a deep ozone ‘hole’ (with calculated ozone of less than 120 Dobson Units (DU)) in the Arctic in 2011, of comparable area to that in Antarctica in recent years. These Arctic ozone depletions would have recurred in future years. The area of the Antarctic ozone hole would also have continued to increase. The area of the ozone hole is defined as the area with a column ozone of 220 DU. (b) The observed area of the Antarctic ozone hole from 2007 to 2014 estimated from Ozone Monitoring Instrument (OMI) data (black symbols), and calculated from model runs including the MP (blue) and without the MP (red). (For the Arctic, no ozone hole has been observed during this period). (c) The percentage difference in the daily zonal mean ultraviolet index at local noon between model runs without and including the MP. (Adapted from Chipperfield, et al. Nat. Commun., 2015, 6). | |
In addition, the Antarctic ozone hole would have been 40% larger in 2013 relative to what was observed, with an enhanced loss of ozone also at sub-polar latitudes of the Southern Hemisphere.1 These large reductions in ozone would have resulted in springtime increases in UV-B radiation at the Earth's surface of about 10% at mid-latitudes and over 20% at high latitudes of both hemispheres. For the implications of these changes for human health, see section 2 below.
1.2 Measurements at several sites over the last decade have shown decreases in surface UV-B radiation that are consistent with observed increases in total ozone
However, at some sites, changes in aerosols, clouds and, at high latitudes, sea ice, were the main drivers of changes in UV-B radiation. Between 1990 and 2011, changes in UV-B irradiance ranging between +0.4 and −8% per decade were found at four northern high-latitude locations (59°–71°N) in agreement with opposite trends in total ozone.2 In contrast, UV irradiance at 325 nm, aerosol optical depth and cloud fraction did not have significant long-term trends. No significant trends in UV irradiance were found for the three southern high-latitude locations considered in this study.
Particularly in urban areas, the effects of aerosols can mask changes in UV-B radiation that arise from changes in ozone. Despite increasing total ozone over the 1991 to 2013 period, erythemally weighted irradiance at Uccle, Belgium, had increased by 7% per decade due to a combination of decreasing amounts of aerosol and cloud, which more than counteracted the effect of ozone.3 Statistical analysis showed that trends in UV-B irradiance and total ozone changed in the late 1990s, consistent with the recovery of stratospheric ozone starting at about the same time.
UV radiation for the period 1950–2011 was reconstructed from a variety of proxy data for nine locations in Spain. The erythemal irradiance increased between 1950 and 2011 by about 13%, of which half was due to decreases in ozone. Between 1985 and 2011, an increase of about 6% was calculated, mostly due to decreasing amounts of aerosols and clouds.4 The day-to-day variability of the noon-time clear-sky UV Index at Thessaloniki, Greece, was found to be influenced more by aerosols than total ozone, even for extreme total ozone events.5
Reflectivity data from several satellites between 1979 and 2012 suggest that decreases in sea-ice and small increases in the amount of clouds over the region of the Southern Ocean around the Antarctic Peninsula have resulted in decreases in UV-B radiation at the surface.6,7
1.3 New measurements have confirmed high levels of UV radiation at low-latitude and high-altitude locations
Extremely large values of the UV Index (peaking at >20) have been measured at four sites of the Tibetan Plateau. These large values are due to the high elevation (3600–4500 m) and low latitude (29–31°N), resulting in small solar zenith angles, and low total ozone.8 In Kampala, Uganda (0.3°N, elevation 1200 m), UV Index values of more than 17 were measured on several occasions between 2005 and 2015,9 while in La Paz, Bolivia (6.5°S, elevation 3420 m), up to 70% of the measurements in 2008–2012 exceeded UV Index values of 10.10
1.4 New studies have improved understanding of the effects of clouds on UV radiation and the physical mechanisms of the processes involved
The weak wavelength-dependence of the cloud optical depth predicted through theoretical calculations was confirmed with measurements. Erythemal and total solar irradiance data recorded at Valencia, Spain, indicate that the difference in the cloud optical depth derived from these measurements is smaller than 2%.11 The effective albedo caused by clouds below a mountain summit was quantified using measured and modeled UV irradiance data from the Izaña observatory (28°N, 2400 m above sea level).12 The largest observed effective albedo value in the UV was 0.58 (i.e., 58% of the incident radiation is reflected upwards). More typical values range between 0.2 and 0.5 and are about 10 times larger than the local surface albedo (0.02–0.05). Measurements combined with radiative transfer modeling confirmed the theoretical predictions that clouds composed of small water droplets attenuate UV radiation more efficiently than clouds composed of large droplets, for the same liquid water content.13 The magnitude of this effect depends on the solar zenith angle and liquid water content.
1.5 Surface UV radiation may have been affected by changes in the amount and optical properties of anthropogenic aerosols, which have been observed at multiple sites worldwide since the year 2000
However, at most sites, no UV radiation data are available to assess these effects quantitatively. Aerosol data from the AERONET network revealed decreases in the amount and absorption efficiency of aerosols (quantified using the single scattering albedo) at most stations since 2000.14–17 These changes were caused by decreases in air-pollution and should have led to increases in surface UV radiation. In contrast, aerosol amounts at many locations in China remain high (the aerosol optical depth at 440 nm ranged between 0.3 and 1.0 and have exceeded 5 during extreme events in some locations) with no significant trend since 2002.18 Measurements at six stations in the USA in 1995–2010 indicated no trend in direct irradiance but a positive trend in diffuse irradiance. This was attributed to an increase in the size of aerosols, possibly due to increased aircraft traffic.19
1.6 The increasing frequency and extent of wildfires due to climate change become important sources of aerosols with significant effects on surface UV radiation
Wildfires across the globe emit black carbon (BC) and organic carbon (OC) smoke particles that can persist in the atmosphere for days to weeks. The inclusion of emissions from fires in climate models increased the predicted global mean annual aerosol optical depth at visible wavelengths by 10%.20 Modeling studies21,22 projected that climate change will increase summertime concentrations of OC aerosol over the western USA by 40–70% and concentrations of BC aerosol by 20–27% in 2050, relative to present levels. Most of this increase (75% for OC and 95% for BC) is caused by larger emissions from wildfires with the remainder caused by changes in meteorological conditions. Such increases in carbonaceous aerosols would lead to significant reductions in UV radiation at the Earth's surface. A recent study has shown that wildfires in Russia in 2010 caused reductions of up to 50% in the daytime averaged photolysis rates of NO2 and O3 (which is driven by UV radiation) along the transport path of the aerosol plume.23
1.7 Over the Arctic and northern high latitudes, surface UV-B radiation is projected to decrease during the 21st century due to the recovery of total ozone, increases in cloud cover, and reduction of surface reflectivity caused by the shrinking of sea-ice and snow cover
Relative to the present, the greatest reductions in surface UV-B radiation (280–315 nm) over land are projected for April under all skies, locally reaching about 30% for the noon UV Index and about 50% for the noon effective dose for the production of vitamin D.24,25 For mid latitudes, the UV Index is projected to show a decrease from 1975 to 2100 of about 5% in the Northern Hemisphere and of about 10% in the Southern Hemisphere, with about equal contributions from projected increases in stratospheric ozone, and cloud cover.26
1.8 In the Arctic region, the processes that led to the sporadic large losses of stratospheric ozone observed in recent years are still unclear
A recent study27 suggests that these losses have not been caused by increasing amounts of greenhouse gases. This was concluded from the analysis of long-term changes in the volume of polar stratospheric clouds derived from measurements in 1979–2011 and model simulations for the 21st century. However, loss of Arctic sea ice may lead to changes in the circulation patterns over the polar region, which could ultimately lead to substantial reductions of polar ozone in March and April, delaying the ozone recovery by up to 12 years.28
1.9 Depletion of Antarctic ozone has influenced the climate in the Southern Hemisphere but there is still no consensus that the ozone hole has caused the recently-observed increasing extent of Antarctic sea ice
Ozone depletion in the late 20th century has likely driven the observed changes in circulation, temperature and salinity of the Southern Ocean.29 Long-term records of data show that changes in circulation due to the ozone hole are contributing to a decrease in summer temperatures over south-east and south-central Australia, and inland areas of the southern tip of Africa.30 In the decades since the appearance of the ozone hole, anomalously high and low total ozone in the spring have been significantly correlated with hotter and colder than normal summers, respectively, over large regions of the Southern Hemisphere, and in particular over Australia.
Increases in the extent of sea ice leads to increased UV-B radiation above the surface, but to decreased radiation penetrating the water under the ice. Potential influences of the Antarctic ozone depletion on sea ice are complex and not well understood. Two processes with different time scales have been proposed:31 in the short-term (a few years to a few decades depending on the model), changes in the ocean circulation cool the sea surface around Antarctica leading to seasonal expansion of the sea ice, but also to upwelling of warm waters in the region of the seasonal sea ice. This leads to an annual expansion and the retreat of sea ice. In the long-term (multiple decades), the effect resulting from upwelling of warm water will dominate, slowly leading to warming of the ocean and ultimately to an overall reduction of sea ice. Based on the results of an improved model it has been argued29 that the cooling due to ozone depletion is weak, does not occur in the month of maximum sea ice extent, and is overwhelmed by the warming due to increases in greenhouse gases.
1.10 El Niño may indirectly influence UV-B radiation
Measurements and model simulations for China suggest that decreases in total ozone during El Niño events lead to increases in clear-sky erythemal irradiance.32 Increases due to El Niño events of up to 6% have been calculated for the middle and lower reaches of the Yangtze River in winter and up to 10% for the northwestern Tibetan Plateau during spring. Changes in cloud patterns associated with these events could also be important for the modulation of UV radiation.
1.11 High quality measurements are necessary to detect and quantify changes in surface UV radiation caused by ozone depletion and, eventually, by ozone recovery
Array spectrographs are increasingly being used for monitoring spectral UV irradiance; however, their accuracy is limited by their inherent instrumental characteristics. A recent study confirmed that measurement errors caused by stray light limit the useful spectral range of these instruments to wavelengths longer than about 305 nm, in particular for small solar elevations.33 Bearing in mind these limitations and the increased uncertainty of data in the UV-B range due to stray light, the usefulness of these instruments is mainly in monitoring the spectrum of radiation in situations when the radiation field changes rapidly (e.g., in partly cloudy conditions or under tree canopies).
1.12 Instruments on satellites can provide reliable estimates of surface UV doses under low-aerosol and clear-sky conditions, but these estimates may be affected by large systematic biases over polluted areas, overcast skies, or snow-covered surfaces
UV Index data from the Ozone Monitoring Instrument (OMI) were validated with ground-based measurements with a NILU-UV multifilter radiometer near New York.34 For clear-sky conditions, the data have good agreement (within 2%), while the OMI bias increases greatly with the optical depth of clouds, reaching −24% on average for overcast conditions.
OMI UV data were also compared with ground-based measurements at 13 stations located in the Arctic and Scandinavia from 60° to 83°N.35 When the surface albedo is known, OMI data typically exceed ground-based measurements by 0–11%. Otherwise biases are much larger; ranging between +55%, when the albedo assumed by OMI is too high, to −59% when it is too low. These large negative biases are observed when reflections from snow and ice, which ultimately increase downwelling UV irradiance, are misinterpreted as reflections from clouds.
By combining satellite data on ozone from GOME-2 and clouds from AVHRR/3, an improved algorithm was developed to estimate surface UV radiation.36 New global datasets of erythemal dose were constructed from OMI measurements for large-scale ecological studies. These data are provided at a 0.25° spatial resolution (about 25 km at the equator).6
1.13 Personal UV dosimeters are useful to estimate exposure to UV radiation and to quantify related effects, such as the status of vitamin D and the damage to skin from UV radiation
While electronic UV dosimeters are more expensive and can be more difficult to use than traditional dosimeters (e.g., spore-37 or polysulfone-dosimeters38) they have several advantages: firstly, electronic dosimeters can be tuned to more closely match the biological weighting of interest than is the case for polysulfone dosimeters, for which differences between their response function and action spectrum of interest are large.39 Secondly, even if there are differences between the instrument response and the target weighting, the latter can still be derived from the measurements using radiative transfer models that include ozone and the solar zenith angle (SZA) as inputs. This is generally not possible for the older dosimeters because of their long integration periods that include a wide range of SZA.40 Thirdly, the time series of data from the electronic dosimeters can assist in quality assurance and in verifying compliance of the users to the measurement protocols. For example, if the dosimeter is not actually worn, but left stationary, that will be obvious from the data record. Finally, because of their more linear response, calibration accuracy will generally be better in the reusable electronic devices, compared with polysulfone dosimeters, which have variable non-linear responses, e.g.ref. 39.
Further research has confirmed that UV irradiance on a horizontal surface (e.g., the UV Index) is not a good indicator of personal exposure. To more realistically model personal exposure, information on the directional distribution of radiation (radiance) would be preferable instead of the more commonly available irradiance data. However, such measurements are not widely available.41
UV dosimeters have recently been used to measure the exposure to UV radiation of members of an Antarctic expedition,42 high school students in Switzerland,43 seafarers working on the decks of vessels,44 skiers,45 tennis players,40 hikers,40 and runners.40,46 Amongst others, it was found that the Swiss students were exposed to 1.7% of the ambient UV irradiance during school days, while 85% of the cumulative UV dose was obtained on weekends and holidays. Skiers in Italy received 65% of the ambient dose on average, as measured by polysulphone detectors. The median daily UV exposure of hikers and tennis players typically exceeded 5 standard erythemal doses (SED), according to one study, but maximum exposures can be much higher.
2 Human health: the effects of solar UV radiation and interactions with climate change
2.1 Risky behaviour regarding sun exposure remains very common among fair-skinned populations, putting them at increased risk of skin cancer and other adverse effects of sun exposure
In the 2010 US National Health Interview Survey, 37% of adults reported sunburn on at least one occasion in the previous year.47 Sunburn was particularly common in young adults (18–29 years, 52%), and in individuals with fair skin (44%). Amongst those reporting sunburn in the previous year, 12% had 4 or more sunburns. In a nationally representative sample of adolescents in the USA, self-reported use of sunscreen declined from 68% in 2001 to 56% in 2011.48 In France, 78% of respondents in a telephone interview reported deliberate sun exposure and 38% did not use sufficient sun protection.49 In one study, the eyes were the least likely part of the body to be protected from sun exposure.50 East Asian immigrants to western countries typically have comparatively low sun exposure, but this increases as they become integrated into the culture of the host country.51 This change in behaviour is beneficial for vitamin D status, but may be associated with an increased risk of skin cancers. In a cross-sectional study of 386 university students in north China, over 90% were aware that exposure to UV radiation caused skin cancer and sunburn, while only 28% were aware of the effects on the risk of developing cataracts and 3% on risk of pterygium (a growth on the surface of the eye, usually on the side closest to the nose, that may cover the cornea and impair vision).50
2.2 The incidence of cutaneous melanoma continues to increase globally, but, in many countries, mortality may have peaked. Exposure to solar UV radiation is the most important cause
A personal or family history of cutaneous melanoma (CM) does not reduce risky sun behaviour. Ongoing exposure of CMs to UV radiation may promote metastasis. The incidence of CM is highest in fair-skinned populations and has risen considerably in recent decades. For example, the age-standardised incidence rate of CM per 100
000 persons in the UK was 17.4 for 2009–2011, an increase of 57% in men and 39% in women, compared to 2000–2002.52 The age-standardised incidence rate increased from 26.7 in 1982 to 48.8 in 2010 in Australia53 and doubled from 1982 to 2011 in the USA.54 A further doubling in incidence by 2030 is predicted for the USA.54 The incidence of CM increases with increasing age.52,54 The incidence rates in children and young adults have decreased over the last ten years in countries such as Australia, which have had several decades of strong public health programs promoting sun protection, but rates continue to increase in other countries.54–59 High-dose sun exposure at any time during life increases the risk of CM, but exposure occurring in childhood years, and associated with the development of nevi, may be particularly important.60
Age-standardised mortality rates due to CM have stabilised in some countries, probably due to a combination of earlier detection, improvements in treatment and reductions in risky behaviour regarding the sun in younger cohorts who have grown up with strong sun protection programs. For example, in the USA the mortality rate was 2.7 per 100
000 persons in 2011, the same as in 1982.54 In Australia, mortality has been stable at around 6.0 per 100
000 from 2004 to 2012.53 In fair-skinned individuals globally, time trends in annual mortality rates for CM from 1955 to 2010 were closely related to the year of birth (age-cohort) with the change in overall rates reflecting a downturn in mortality in younger age groups.61 The peak years for CM mortality were for those born in 1936–1940 in Oceania, 1937–43 in North America, 1945–53 in the UK and Ireland and 1957 in Central Europe.
One study showed that, following a diagnosis of CM, patients in Denmark still expose themselves to high levels of solar UV radiation without using personal protection, particularly when on holiday.62 Similarly, children of people who have survived CM had higher levels of sun exposure and sunburn than average-risk populations in a study from California.63 Contrary to a previous report,64 higher levels of sun exposure prior to diagnosis of CM were not associated with a reduction in subsequent mortality65 when examined with a rigorous study design focused on this specific question. Furthermore, in a mouse model, repetitive exposure of primary CMs to UV radiation promoted a spread to blood vessels and metastasis to the lung.66
2.3 The incidence of non-melanoma skin cancers continues to increase, primarily in fair-skinned populations
Changes in patterns of sun exposure over time are resulting in a more rapid increase in the incidence of basal cell carcinoma (BCC) compared to that of squamous cell carcinoma (SCC). In the USA there was a 14% increase in the age-adjusted rate of procedures (a proxy for incidence) for non-melanoma skin cancers (NMSCs) between 2006 and 2012.67 Extrapolating to the US population, the estimated total number of NMSCs was almost 5.5 million in 2012, with approximately 3.3 million people treated. Patterns of sun exposure have changed over time, with increased intermittent recreational exposure, most frequently occurring in locations with high ambient levels of UV radiation. As a consequence, the incidence of BCC is increasing at a faster rate than that of SCC in younger people.68,69 Sun protection programs that have been shown to be effective in lowering the incidence of NMSC should have a particular focus on leisure-time sun exposure in young people.
A study in South Africa showed that the mean age-standardised annual incidences (per 100
000 people, figures are female, male incidence) of BCC, SCC and CM in 2000–2004 were highest in groups classified in the study as white (BCC: 113, 198; SCC: 32, 70; CM: 17, 21), followed by the coloured (BCC: 27, 59; SCC: 15, 26; CM: 4, 6), Asian (BCC: 5, 8; SCC: 3, 4; CM: 1, 1) and then black (BCC: 2, 3; SCC: 2, 3; CM: 1, 1) population groups.70 SCC and BCC were most commonly found on the head.
A recent review of studies quantifying the direct costs of NMSC to health care systems found that these costs were highest for the USA (nearly 600 million € annually) followed by Australia (around 350 million € annually), although after adjustment for population, costs were greatest for Australia, followed by New Zealand, Sweden and Denmark.71 Skin cancer prevention initiatives were shown to be highly cost-effective and possibly cost-saving.
2.4 Both UV-A and UV-B radiation can lead to the formation of skin cancer
While UV-B irradiation is well known to cause DNA damage, UV-A (315–400 nm) irradiation also causes cellular damage through the generation of reactive oxygen species, as well as causing immunosuppression. There is increasing evidence to suggest that UV-A irradiation can also cause the production of thymine dimers in the DNA of basal keratinocytes and influence gene expression.72 UV-A radiation may be implicated in the development of both CM and NMSC through its role in the inhibition of nucleotide excision repair.73
2.5 Several eye diseases, including cancers of the eyelid and the surface of the eye, cortical cataract and pterygium, are strongly related to exposure to UV radiation
The evidence is less clear for other diseases of the eye, such as nuclear and posterior subcapsular cataract, ocular melanoma and age-related macular degeneration. Exposure to UV radiation causes cancers of the eyelids, principally BCC.74 The causative role of exposure to UV radiation in ocular squamous neoplasia may involve a combination of DNA damage, local and systemic immunosuppression, and the reactivation of latent viruses.75 Specific genetic and epigenetic changes in genes involved in oxidative stress may increase susceptibility to UV radiation-induced development of cortical cataracts.76,77 In China, there was an increase in disability-adjusted life years (DALYs) for cataract of 92 per 100
000 population for every 1000 J m−2 increase in ambient erythemal UV radiation estimated from satellite data.78 For the population aged ≥75 years this figure increased to 1342 DALYs per 100
000 people.
The risk of exfoliation syndrome (an age-related disease that is the most common identifiable cause of glaucoma) increased with greater time outdoors and a history of work over water or snow, and reduced with the use of sunglasses in a clinic-based case-control study in the USA and Israel.79 Blue light, rather than UV radiation, may play a previously-unacknowledged role in the pathogenesis of uveal melanoma,80 as well as age-related macular degeneration.81
2.6 Less time outdoors appears to be a major risk factor for the development of myopia, but whether this is an effect of UV radiation is not clear
In a birth cohort study, myopia (defined as a mean refractive error of both eyes ≤−0.5 diopters) in young Australian adults was significantly less common in participants with objective evidence of greater sun damage to the eye (measured as the area of conjunctival autofluorescence),82 and was significantly more common in individuals with vitamin D deficiency.83 These findings support the hypothesis that outdoor light exposure may be protective against myopia, with the relevant wavelengths, or the role of UV radiation, not yet determined.
2.7 Exposure to solar UV radiation can alter the immune response to a variety of microorganisms in animal studies, and recent reports support a similar role in humans
The risk of recurrence of infection with herpes simplex virus in the eye was increased three-fold in people in North Carolina who had the highest outdoor exposure (8 hours or more per week outdoors when the UV Index was ≥4) compared with those having the least outdoor exposure (7 hours or fewer outdoors when the UV Index was <4).84 In studies in Korea85 and Taiwan,86 the occurrence of shingles, caused by the reactivation of herpes zoster virus, was more common in the sunny months than in the winter, possibly due to the immunosuppression induced by exposure to higher levels of solar UV radiation.
In a systematic review of 24 randomised trials, the effectiveness of the BCG vaccine against tuberculosis (TB) decreased with closer proximity to the Equator and increasing levels of UV radiation, possibly due to UV-induced suppression of the immune response to the vaccination.87 Furthermore, in a study over 28 years in Birmingham, UK, there was strong evidence for seasonality of TB, with notifications being 24% higher in the summer than in the winter.88 The lesions of dermal leishmaniasis (a parasitic infection spread by sandflies) that can arise after an apparent cure from visceral leishmaniasis, appear on sun-exposed areas of the body.89 Exposure to solar UV radiation is thought to play a key role, acting by suppressing immune responses in the skin at the exposed body sites.
A study in the USA found a significant positive correlation between the average annual levels of UV radiation for each State and the incidence of oral and pharyngeal cancer in people with fair skin, but not in those with deeply pigmented skin.90 There was also a positive correlation between levels of UV radiation and the incidence of cervical cancer in white women. Human papilloma virus (HPV) is commonly found in each of these cancers; the variation in incidence in relation to levels of UV radiation suggests that exposure to solar UV radiation may induce the production of soluble mediators that promote HPV-associated tumorigenesis at non-exposed body sites.
2.8 Despite much research into a wide range of disease outcomes, the role of vitamin D in human health, beyond its importance for calcium homeostasis and the maintenance of bone, has not been confirmed91
Recent reviews92,93 show that vitamin D deficiency (a concentration of 25-hydroxyvitamin D [25(OH)D] in serum or plasma of less than 50 nmol L−1) is common worldwide, although the use of different assays for which accuracy is not assured makes it difficult to fully assess the magnitude of the problem. One study showed a high prevalence of vitamin D deficiency in young children in Iran that was the result of high levels of air pollution, leading to low levels of exposure to UV radiation.94 New work is providing greater guidance on how much sun exposure is required for the avoidance of vitamin D deficiency, including in people with different skin types.95 In Australia, a program of sun exposure led to increased 25(OH)D levels in older people in residential care but was only effective when started in spring or autumn, and some individuals did not achieve vitamin D sufficiency.96
Many observational studies show that low serum 25(OH)D concentrations are associated with increased risk, progression, severity and mortality for many disorders, including cancers,97 autoimmune diseases such as lupus,98,99 inflammatory bowel disease100 and multiple sclerosis,101 poor cognitive function,102 attention deficit hyperactivity disorder103,104 and myopia.83 Other studies show no effect of vitamin D. Intervention studies using supplementation with vitamin D show no benefit for most health outcomes tested.91 The major determinant of serum 25(OH)D concentration in many populations is recent sun exposure or time outdoors.105 This means that serum 25(OH)D, as well as being the usual measure of vitamin D status, is a marker for recent sun exposure and time outdoors. Intervention studies using supplementation with vitamin D test a specific effect of vitamin D. However, associations with 25(OH)D may be due to the link with other factors related to recent sun exposure and time outdoors such as physical activity or exposure to UV radiation, rather than vitamin D status per se. The lack of a beneficial effect of supplementation with vitamin D suggests that there is, in fact, no causal association between higher vitamin D status and better health, but that other factors associated with the level of 25(OH)D may be important for health. Alternatively, supplementation studies may have had null results due to study designs where the supplementation dose was too low, for too short a time, in people without vitamin D deficiency at the beginning of the study, or the sample size of the study was too small. In a few supplement studies there has been evidence of improvement in disease symptoms, for example for lupus,98,99 and autism106 but the designs used in the studies do not allow firm conclusions to be drawn. The role of vitamin D in health and disease, the optimal level and the size of any effect, remain unclear.
Sun exposure and/or vitamin D during pregnancy may be particularly important for the health of offspring. Vitamin D status in pregnancy was inversely related to the risk of attention deficit hyperactivity disorder in the offspring107 in one study, but was not in another, and low 25(OH)D level in umbilical cord blood has been correlated with an increased risk of transient wheezing and atopic dermatitis in early childhood.108 Higher maternal vitamin D status was associated with an increased risk of depression in the offspring in another report.109
A few studies show that both low and high levels of 25(OH)D are associated with increased risk of an adverse health outcome, such as all-cause mortality,110 or an adverse effect of higher 25(OH)D levels for prostate cancer,111 and hypertension112 suggesting that optimal levels are not necessarily high levels.
2.9 Other potential benefits and risks of exposure to UV radiation are being revealed. Higher sun exposure is associated with a reduced risk of some cancers and autoimmune diseases, but whether this is a causal association is not clear
In Finland, fishermen, but not their wives, had higher incidence of lip cancer but lower incidence of colon cancer than the general Finnish population.113 Whether these cancers are linked to the higher exposure to UV radiation, or the presumed higher consumption of fish, was not explored, although the discrepancy in risk between the men and their wives might lend weight to a UV-related explanation rather than a dietary cause. In the USA, the incidence of several sub-types of non-Hodgkin lymphoma was significantly lower in the highest compared to the lowest quintile of ambient UV radiation (average daily cloud-adjusted noon-time UV irradiance at 305 nm (UV-B) over 1982–92) for the residential location, with some effects being more pronounced in fair- compared to dark-skinned populations.114 Similarly, incidence rates for multiple myeloma, a cancer of the plasma cells which often spreads to the bone marrow, were greater at higher latitudes and there was an independent inverse association between UV-B irradiance and the incidence of multiple myeloma.115 In contrast, another study showed that higher residential erythemal UV radiation was associated with an increased risk of B-cell acute lymphoblastic leukemia in children aged less than five years; there was a threshold effect, with risk increasing at levels of ambient UV radiation above 100 J cm−2.116
Several studies report an association between higher levels of sun exposure or ambient UV radiation and a reduction in inflammatory bowel disease: the risk of Crohn's disease,117 and hospitalisation and need for bowel surgery for both ulcerative colitis and Crohn's disease.118 The well-described seasonal variation in disease relapses in people with multiple sclerosis shows latitudinal variation, with a shorter gap between relapses in people living at higher latitude.119 There is a strong latitudinal gradient of increasing rates of hospital admission for anaphylaxis (a severe allergic reaction) in children in Chile; that is, with increasing latitude above 34°S and lower levels of solar UV radiation.120
An inverse association between mortality from all causes and levels of UV radiation or personal sun exposure have been described for dialysis patients,121 female participants of a large cohort study in Sweden at the 20-year follow-up,122 and in premature babies (23–28 weeks gestation) in the first 28 days of life.123
Many commonly used drugs can be phototoxic or cause photosensitivity. Photosensitivity reactions are reported with angiotensin II receptor blockers, used to treat hypertension.124 In addition, long-term use of these drugs is associated with an increased risk of CM.125 Long-term use of diuretics may increase the risk of SCC and BCC.125 Additional advice on sun protection should be provided when these drugs are prescribed.
Higher levels of UV radiation have been associated with lower levels of serum folate,126 including in women of childbearing age.127 Whether this effect is sufficient to alter the risk of disorders such as neural tube defects in humans is not clear. The combination of high dietary folate intake and high levels of exposure to UV radiation may potentiate DNA damage128 and the risk of BCC.129
2.10 Infrequent use and poor understanding of labels and application of sunscreens are common, resulting in people overestimating their sun protection. There is mixed evidence on the long-term benefits of the use of sunscreens for the prevention of skin cancers
Sunscreens are not used commonly in some countries.130–132 Even where their use is common, sunscreens are often applied at a thickness that is one-fifth to one-half of that required to achieve the rated sun protection factor (SPF).133,134 Poor application may continue even after training,135 but new approaches to training for application may improve effective protection.136 The use of higher SPF sunscreens (e.g. SPF 50+) may compensate to some extent for the application thickness being less than optimal. New sunscreens provide better protection within the UV-A waveband, but, for a given SPF, increasing protection within the UV-A is accompanied by reduced protection within the UV-B waveband.137
A randomised controlled trial in an older Australian cohort showed that regular daily use of sunscreen reduced the risk of actinic keratosis (a scaly growth on the skin that may be a pre-malignant stage of SCC), SCC, and CM.138 In contrast, increasing use of sunscreens from 1997–2007 in a large cohort of Norwegian women was associated with an increased risk of sunburn,139 and a meta-analysis of observational data from Europe, America and Oceania showed no significant increase in the risk or benefit of sunscreen use for CM.140
A wide range of oral/nutritional photo-protective agents is being investigated.141,142 There is considerable interest in the development of natural biocompatible sunscreens,143 including plant extracts144–146 and compounds ingested by marine animals (e.g. mycosporine-like amino acids) that probably function as sunscreens in tissues exposed to UV radiation.147–150 Recent work also notes adverse health effects such as allergic and photo-allergic contact dermatitis from the use of synthetic sunscreens,151,152 and that sunscreens may be endocrine disrupters153 and have adverse effects on marine ecosystems (see section 4.5 below).
2.11 Sunglasses and contact lenses provide excellent protection from UV radiation, although in some countries labelling of sun protection factors may be inaccurate
A proposed new UV protection label for eyewear, the eye-sun protection factor (E-SPF), integrates the transmission of UV radiation through the lens and the reflection of UV radiation from the back of the lens (caused by coatings on both sides of the lens to reduce glare).154 Validation of its biological relevance is now required.155 A high proportion of sunglasses purchased from unauthorized dealers in developing countries do not comply with international standards for UV protection.156 Testing of four commercially available soft contact lenses showed that they blocked 89–99% of UV-B radiation,157 Use of UV-blocking contact lenses and spectacles was associated with reduced UV-induced damage as measured by autofluorescence in the conjunctiva near the nose but not in that on the outer side of the eye.158
2.12 Research on the interactions between UV radiation and the effects of climate change on human health remains sparse
UV radiation may alter the physiology of some mosquitoes leading to an increased tolerance to some chemical insecticides and this effect may be accentuated by environmental pollution.159 The importance of these interactions for future control of vectors of diseases is not clear.
In a study of people with white skin in the USA, the incidence of BCC increased in relation to increasing levels of lifetime residential ambient UV radiation and was also higher in the fourth (compared to the first) quintile of ambient temperature.160 It is difficult to predict from this ecological study whether there might be interactions between exposure to UV radiation and warmer temperatures due to climate change that could affect the risk of skin cancer. In another study, temperature was an important determinant of time spent outdoors in cooler, but not hotter, climates.161 Thus, at locations where climate change leads to ambient temperatures in a “comfortable” range, people are likely to spend more time outdoors and have higher exposure to UV radiation. In contrast, if climate change leads to temperatures outside this range, people are more likely to spend more time indoors, and receive less UV radiation (also see a further discussion in section 4.4 below).
3 Terrestrial ecosystems: the effects of solar UV radiation and interactions with climate change
3.1 Agriculture and food security are likely to be more adversely affected as a result of the projected climate variability and changing levels of UV radiation, but some beneficial outcomes may also become evident
Projected increases in the intensity and frequency of unfavourable climate events such as heatwaves, flooding, and recurring dry seasons (see sections 1.4 above and 3.10 below) together with changing levels of UV radiation that are linked to climate change, can have adverse effects on the production of crops. On the other hand, in addition to an improved understanding of the way in which interactions between exposure to UV radiation and climate change can alter plant response, opportunities for exploiting some of the resultant modifications of plants are relevant with respect to food production, nutrition, and resilience of plants to stress in general. However, crop plants are usually cultivated in dense stands, where beneficial effects of solar UV-B radiation on e.g., the immunity of plants, could be lost.162,163
Further work has been directed to productivity, quality traits of plants, and adaptive mechanisms164,165 in order to address the effects of UV radiation and rising temperatures on plants. Across a range of plant species, increasing temperature during the early development of plants enhances growth, while exposure to realistic levels of UV-B radiation165,166 counteracts the growth response. Other growth stages, such as the onset of flowering, are delayed by elevated levels of UV-B radiation, with resultant decreases in fruit and seed production (ref. 167 and references therein). These modifications during plant growth may become more common in areas that are receiving high levels of UV radiation, mostly because of projected decreases in cloud cover and aerosols in some regions.4 Current high UV radiation levels also occur in certain high-altitude and low latitude locations.8
UV-B-mediated shifts in the allocation of resources (such as sugars, mineral ions, and water) within the plant may have important consequences for agriculture. Redirection of these resources away from defense mechanisms and toward processes for rapid growth in response to attenuation of UV-B radiation in high-density stands168 may have negative consequences for plant health in agricultural settings.169 Further research on the molecular connections between the perception of UV-B radiation and the regulation of plant immunity may provide clues to improve the resistance of crop plants to pests and pathogens under the conditions of high-density agricultural practices.
The use of pesticides in agriculture continues to be a concern. It is known that UV radiation facilitates the breakdown of pesticides170,171 and may in some cases increase the toxicity of certain pesticides and/or their degradation products (see section 5.5). The detrimental effects to the plant may result in an increase in the permeability of membranes leading to increased uptake of pesticides when plants are exposed to elevated levels of UV radiation.172,173 Because of the potentially harmful effects of pesticides on the environment, more selective biological methods of control are being developed. The use of certain fungi (entomopathogenic) constitute one biological control measure, although unfortunately they also can be very sensitive to UV-B radiation. This will decrease their usefulness as biological controls in regions of enhanced UV-B radiation.174,175
3.2 In terms of plant adaptation, there are several implications for food production and altered plant attributes as a consequence of exposure to UV radiation and a rapidly changing and variable climate
While plant response can indicate mechanisms of adaptation, some of these adaptations are also of interest from an agricultural, horticultural, and nutritional perspective (ref. 163, 176, 177, and references therein) and can be managed by changing certain practices of crop management for beneficial outcomes. For example, stimulation by UV radiation of polyphenolics can be used to increase the nutritional quality of plant products and plant tolerance to stress conditions by manipulating the exposure of crops to UV radiation through e.g., opening of the plant canopy or the use of filters.162,176,178
Other examples include the modulating effect of UV radiation on the growth of grape vines and subsequent alterations in the composition of the berry fruit and resulting juice or wine. These changes can be used by growers to experimentally manipulate conditions to alter the taste, quality of the berry, and appearance of the wine, and cross-tolerance to environmental stressors179–181 including fungal infections,182,183 and temperature.179 In many geographical regions, a concern arising from increasing temperature is that certain crops, including grape vines, show more rapid ripening that affects quality (e.g. increased alcohol content in wine181). However, when multiple, interacting environmental variables are taken into account, such as exposure to increasing temperature and elevated carbon dioxide levels, UV-B radiation may also alleviate oxidative damage as well as offset the hastening of ripening by the increased carbon dioxide levels and temperature.181
3.3 Some male and female plants have been reported to respond differently to temperature and UV radiation
Changes in sex ratios in these plants as a result of exposure to UV-B radiation and temperature may modify ecosystems and the spatial distribution of plant habitats. For example, in certain trees and shrubs, male (pollen-producing) plants establish and grow more rapidly than females (seed producing), perhaps because less energy is allocated to reproduction than in female plants. Tolerance of environmental stresses, such as UV-B radiation, water-deficiency, increased salinity, and temperature, also appears to differ in populations of male and female plants resulting in the ability for gender proportions across a population to be changed. Male plants may be more tolerant of stresses than females.184–187 This greater stress tolerance can result from increased temperature and UV-B radiation.188 However, the temperature-driven enhanced production of protective flavonoid compounds in female plants187 further illustrates differences in the sexes. Thus modifications at certain life stages in male and female plants reflect complex interactions between exposure to enhanced UV-B radiation and rising temperature, and may therefore alter the balance of plant gender and the establishment of a species.
3.4 Solar UV radiation can increase rates of decomposition of plant litter via photodegradation, especially in ecosystems with arid and semi-arid climates (i.e., grasslands, savannas, and deserts)
Litter from different plant species varies considerably in its rate of degradation and the precise chemical changes of litter resulting from photodegradation remain unclear.177 Lignin is thought to be a primary molecular target of photodegradation,177,189,190 but variation among species in the susceptibility of litter to photodegradation appears not to be solely related to lignin levels191 or optical properties that influence the penetration of UV radiation.192 Results from a meta-analysis191 suggest that the density of litter and thus the surface area of litter exposed to UV radiation can be an important attribute influencing the photodegradation of litter (see section 5.3 below). UV radiation directly degrades lignin structures193 and indirectly alters other cellular constituents, such as cellulose and hemicellulose, and these chemical changes may then enhance microbial decomposition of litter (ref. 190, 194 but see also ref. 195).
3.5 UV radiation influences the decomposition of litter through several interacting and possibly synergistic abiotic and biotic mechanisms
The indirect enhancement effects of UV radiation on litter can apparently persist for some time and potentially carry over from seasonal dry periods (i.e., summer when UV radiation levels are typically high) to cooler, moist periods (winters) when the temperature and moisture conditions are more favourable for microbial decomposition.196 This enhanced stimulation of microbial decomposition through UV radiation also appears to extend to partially decomposed soil organic matter.197 Results from a recent meta-analysis198 suggest that the combined effect of UV radiation on abiotic (photodegradation) and biotic (microbial) decomposition processes contributes to a greater mass loss of litter than photodegradation alone; and lignin loss is greater when photodegradation interacts with microbial decomposition (see also section 5). An increased understanding of the mechanisms of the effects of UV radiation on the decomposition of litter will increase our ability to predict how climate change will affect this process.
3.6 Climate change can potentially mediate the effects of UV radiation on the decomposition of litter through various direct and indirect pathways
However, few studies have experimentally examined the interactive direct effects of climate change and UV radiation on litter decomposition (also see Section 5.3 below). Results from an experimental study of Mediterranean grasslands (see also section 5.3 below) indicate that reduced rainfall and increased temperature decreased the rates of decomposition in both the standing and ground litter of a perennial grass species (Stipa tenacissima). In addition, ambient UV radiation increased the rate of decomposition but only under dry, warm conditions.199 These findings suggest that, at least in these ecosystems, the relative importance of UV-driven photodegradation may increase with climate change with potential consequences for carbon cycling and sequestration. Climate change may also influence litter decomposition indirectly via changes in vegetation composition, structure, and fire (see section 3.10 below).
3.7 The molecular mechanisms by which terrestrial plants respond to changes in UV-B radiation are becoming better understood
Since the mechanism of perception of UV-B radiation by the UVR8 photoreceptor protein was elucidated in plants,200 there has been substantial progress in the understanding of the downstream signaling events that lead to physiological responses, including the activation of protection mechanisms (reviewed in ref. 201). This improved understanding of the perception of UV-B radiation and signaling in plants has important implications for biotechnology. Biotechnological applications of this knowledge include the enhancement of desirable responses to UV-B radiation in crops (such as the accumulation of pigments and antioxidants in fruits, vegetables and other crops)177,202 and the development of novel tools that allow the manipulation of cellular activities with light.203
3.8 There is further evidence that plants use solar UV-B radiation as a signal of light availability and the intensity of competition from other plants
Although high levels of UV-B radiation can be harmful for some organisms, it is becoming increasingly clear that terrestrial plants have effective protective mechanisms against the damaging effects of UV-B radiation. These mechanisms include the accumulation of protective UV-absorbing pigments in epidermal cells and the cell walls of plants,177,204–209 the activation of antioxidant systems210 and increased expression of genes involved in DNA repair mechanisms. In certain species, the UV-absorbing pigments can be adjusted on a diurnal basis in response to changes in solar UV radiation.211,212 Many of these protective responses are regulated by the UV-photoreceptor, UVR8.213 In addition, plants use UVR8, along with other photoreceptor proteins, to sense changes in the light environment caused by the proximity of other plants168,214). Under low light conditions (shade) that inactivate UVR8, resources are redirected from defense to rapid growth.168 This strategy helps the plant to compete for light with its neighbours, but also makes it more vulnerable to the attack of pathogens and pests.
3.9 High sensitivity to UV-B radiation has been demonstrated in some invertebrates
Terrestrial arthropods (such as insects, spiders, and millipedes) can be affected by UV-B radiation via effects on their food sources (mainly plants, e.g., ref. 202, 215 and 216). It has usually been assumed, without much hard evidence, that the exoskeleton of arthropods affords good protection against the direct effects of UV-B radiation for most terrestrial arthropods. However, high sensitivity to UV-B radiation occurs in aphids217–219 and mites.220–222 Some arthropods also sense and respond to solar UV-B radiation with avoidance behaviour.223
Adult damselflies are able to coat the mouthparts of ectoparasitic water mites with melanin (invertebrate melanotic encapsulation) as an effective response to deter these parasites. However, if damselfly larvae are exposed to UV-B radiation, they produce more melanin in their exoskeleton for protection from UV-B damage. As a result, the adults are smaller and have a reduced immunity response (30% reduction in melanotic encapsulation). This constitutes the first evidence for a UV-driven impaired immune response in an insect.224
3.10 Ozone depletion is changing the global climate (wind patterns, temperature, and precipitation) across the globe with consequences for agriculture, ecosystems, and human health
These ozone-related changes are manifested at the Earth's surface in the Southern Hemisphere during summer (December–February) and in the Northern Hemisphere in spring (April–May),29,31,177,189,225–229 with widespread implications for terrestrial and aquatic ecosystems, human health and food security, some of which are detailed below.
Changing wind patterns and intensity.
More intense mid-latitude winds linked to ozone depletion could reduce or enhance the ability of the oceans to sequester carbon.189,227,230 Enhanced wind-driven upwelling of carbon-rich deep water would result in less uptake of atmospheric carbon dioxide (CO2) by the oceans, reducing their potential to absorb carbon.177,189 However, winds can also transport more dust from drying areas, such as South America, into the oceans, enhancing fertilisation by iron and resulting in more plankton and greater carbon uptake.189,227,231 These stronger more southerly winds have also been shown to have positive impacts on some foraging sea birds232–234 with the sub-Antarctic wandering albatross showing a 15% increase in both body weight and breeding success.232 However, for the flightless southern rockhopper penguins, the poleward shift of the jet stream is predicted to reduce the number of days with westerly winds in the future, leading to fewer days with favourable foraging conditions.235
Changing temperatures.
Ozone depletion at the South Pole is associated with the cooling of East Antarctica (see ref. 227), while ozone depletion at the North Pole is associated with springtime cooling over China, Greenland and north-eastern Canada,228 but northern Europe and Siberia show enhanced springtime warming. These changes in temperature have likely reduced melting of the ice sheet in polar regions and resulted in changed phenology (e.g., mismatches between plants and their pollinators). The depletion of Antarctic ozone has possibly offset a substantial portion of the summer warming that would otherwise have occurred in Eastern Australia, Southern Africa and South America, due to increasing amounts of greenhouse gases (ref. 30 and section 1.9 above). As ozone concentrations recover, this amelioration may reduce with potential implications for the populations of these regions.
Changing precipitation.
Ozone depletion is similarly associated with changing precipitation across the globe.225–229,237 More springtime precipitation occurs at high latitudes in the North Atlantic Ocean (70°W to 20°E) and in Siberia (50–120°E), associated with extremely low polar stratospheric ozone. Regional changes in precipitation will likely alter the levels and transparency of water and hence the exposure to UV radiation in aquatic ecosystems by the mechanisms mentioned in section 4.1. As detailed in our last assessment,177 terrestrial ecosystems in the Southern Hemisphere are being affected by these ozone-related climate changes. Wetter summers have seen increased tree growth in eastern New Zealand236 and the expansion of agriculture in south-eastern South America.237 Conversely, in Patagonia and East Antarctica, declining growth of trees and moss beds has been linked to a reduced availability of water.177,227,238
Changes in wind patterns, temperature, ocean salinity and precipitation are known to have an impact on agriculture and food security, human health, marine and terrestrial ecosystem health.239 However, there have been relatively few studies (i.e., none for the Northern Hemisphere) that directly consider these climate-related effects of ozone depletion. Ecological and health studies aimed at revealing such effects would probably be most effective if they focused on regions with statistically significant correlations between the spring-time depletion of ozone and either summertime surface temperature (in the Southern Hemisphere) or springtime surface temperature (in the Northern Hemisphere).
4 Aquatic ecosystems: the effects of solar UV radiation and interactions with climate change
4.1 Climate change is altering the exposure of aquatic ecosystems to UV radiation through a variety of mechanisms
Incident UV radiation is no longer increasing due to stratospheric ozone depletion, and a slow return to pre-1980 levels of stratospheric ozone is expected in the coming decades. However, exposure to UV radiation in inland, coastal, and open ocean waters continues to be altered by changes in ice and snow cover, cloud cover, increases in thermal stratification of the water column induced by warmer air temperatures, and by heavy precipitation that reduces the UV transparency of water.
Climate change is altering the concentrations of UV-absorbing substances such as terrestrially-derived dissolved organic matter (DOM) in inland and coastal waters through altering the amount, timing, and type of precipitation and the frequency of extreme precipitation and drought events.240,241 Increases in precipitation, including extreme events and flooding, increase the concentrations of DOM and the turbidity in inland and coastal aquatic ecosystems, reducing their UV transparency (Fig. 2).242
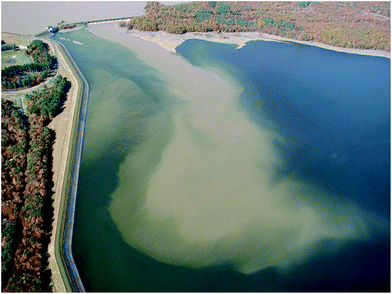 |
| Fig. 2 Plume of turbid water entering the east basin of New York City's Ashokan Reservoir following an extreme runoff event. The increase in extreme precipitation events in many regions of the world will decrease UV transparency, the potential for solar disinfection of parasites and pathogens, and increase the cost of effective disinfection of drinking water sources. See the text for details. Photo credit: Randall Hurlbert. | |
An 18-year study in Sagami Bay, Japan, found that seasonal reductions in UV transparency and shallower mixing depths are related to increases in DOM concentrations and to weather patterns related to the Pacific Decadal Oscillation index (an ocean-based oscillation that influences regional weather, as does El Niño).243 Increases in DOM and changes in UV transparency can alter microbial food webs244 and the distribution and abundance of zooplankton.245–249 Dietary photoprotective compounds can decrease the threat of damage by UV radiation and reduce the need for behavioural responses in zooplankton.245
The fate of DOM in inland waters is also important for the global carbon cycle, because inland waters release large amounts of CO2 (∼2 petagrams (Pg) per year), much of which is derived from DOM.250 While microbial activity has generally been thought to be the primary mechanism of breakdown of DOM in aquatic ecosystems, recent data suggest that solar UV radiation may be responsible for 70–95% of the breakdown of this substantial pool of organic carbon in aquatic ecosystems in the Arctic.250,251 This sunlight-driven degradation of DOM has important implications for biogeochemical cycles and greenhouse gas production, as discussed in more detail in section 5.2 below.
The seasonal duration and thickness of ice cover in aquatic ecosystems is decreasing globally, increasing exposure to UV radiation.252 In the Arctic, the areal extent and thickness of sea ice continue to decline. Recent modeling efforts estimate that exposure to the UV-B wavelength range in the surface waters of the Arctic Sea may increase by as much as tenfold between 1950 and 2100 due to the melting of sea ice.253 Copepods, which are the dominant grazers in the oceans, ascend to shallower waters around the time of ice-out in the Arctic, which has the potential to increase their exposure to UV radiation. Increases in photoprotective compounds in the copepods at this time of year reduces the potential for damage by UV radiation.254 In the Antarctic there is a different pattern of sea ice. The areal extent of Antarctic Sea ice has been increasing by about 1% per year,255 but the thickness of the ice has been decreasing at an accelerating rate with as much as 18% loss within two decades in some regions.256 Recent modeling indicates that polar stratospheric ozone depletion may alter the areal extent of sea ice; however, the direction of this effect is still a matter of debate.31,255 In contrast, other modeling has shown that while ozone depletion is altering the temperature and salinity of the Southern Ocean, effects on Antarctic Sea ice are not likely.31 In the Arctic, models that compare the increase in seasonal loss of sea ice in the late twenty-first versus the late twentieth century predict that climate change-induced sea ice loss will reduce ozone by 13 Dobson Units during the spring.28 This small percentage loss in ozone is not likely to lead to important feedbacks between ozone depletion and sea ice. These potential feedbacks between ozone and sea ice are covered in more detail in sections 1.2, 1.8, and 1.9 above.
4.2 Increasing air temperatures have led to a warming of surface waters in lakes and oceans, increasing the strength of thermal stratification and exposure of surface-dwelling organisms to UV radiation
Mean annual temperatures of oceanic surface water have become warmer by about 1 °C over the last 125 years with increases in thermal stratification and a reduction in the depth of the upper mixed layer, as well as deep ocean warming.257 Since most of the primary producers are found in this sunlit zone, they are effectively exposed to higher levels of visible light and UV radiation. Stronger stratification also hampers the upwelling of nutrients from deeper water, reducing productivity and altering the species composition of primary producers.258 There is also some evidence that, by reducing the average exposure of algae to UV radiation, greater concentrations of DOM may thus reduce the photoprotection and UV tolerance of algae. Under some conditions these algae may be circulated into the near surface waters where they are exposed to high UV radiation and subsequently more readily damaged.259
4.3 In contrast to the warming of most ocean waters, there is a significant cooling in the North Atlantic between Greenland and Ireland
This is due to a weakening of the Gulf Stream that heats the North Atlantic, the American east coast, and Northern Europe,260 and a decline in the Atlantic Meridional Overturning Circulation (AMOC).261 These changes in oceanic currents and surface temperatures alter thermal stratification and thus the exposure of marine organisms to UV radiation.
Warming of the climate is altering the strength, depth, and duration of thermal stratification in inland water bodies as well. This will change the exposure to UV radiation with the response varying with the depth, surface area, and water clarity of the ecosystem.262,263 Deeper, warmer lakes (e.g., tropical lakes and reservoirs) are increasingly becoming stratified faster and remain so for longer than shallower, cooler lakes.263 The transparency of water modulates the amount of solar UV radiation penetrating the water column and is also a primary modifier of thermal stratification.264 While lower transparency reduces exposure to UV radiation on a depth-averaged basis, it can also warm surface waters, cool bottom waters, and increase stratification. Reduced transparency in clear-water systems substantially increases the duration of stratification and delays the onset of ice cover,264 thereby extending the ice-free season. Longer ice-free periods and increased thermal stratification increase the exposure of surface-dwelling organisms to UV radiation, alter vertical habitat gradients of food and predation in the water column, and modify nutrient cycling and gas exchange rates including those for greenhouse gases. These changes in aquatic ecosystems have the potential to reduce biodiversity through increased exposure to UV radiation, increase greenhouse gas production, and create mismatches between consumers and their food resources that can decrease consumer abundance.
4.4 Acidification of the oceans and associated increases in the concentrations of CO2 in the oceans interact with the effects of UV radiation, climate change, and other stressors in affecting marine organisms
A recent review highlighted the fact that acidification of the oceans is exacerbated by inputs of CO2 derived from the degradation of DOM as well as from the atmosphere.265 In coastal regions, increased runoff containing DOM and nutrients associated with climate-driven extreme precipitation events can further increase the concentrations of CO2 in seawater and accelerate acidification beyond that expected from elevated atmospheric CO2 alone.265
The rates of growth and/or photosynthesis in ecologically important algae can be inhibited by interactions between the effects of UV radiation and acidification266 as well as by interactions between these two stressors and limitation of nutrients.267 In contrast, the elevated concentrations of CO2 associated with acidification may ameliorate the negative effects of photoinhibition by UV radiation in some marine diatoms by increasing photosynthesis as long as exposure to UV radiation is not so high as to cause damage.268
4.5 An increase in the prevalence of water-borne parasites is a likely response to reduced solar disinfection from decreased penetration of UV radiation in aquatic ecosystems induced by increases in precipitation and runoff from terrestrial ecosystems
It has long been recognised that viral, bacterial, and protozoan pathogens and parasites are inactivated by exposure to solar UV radiation. Decreases in the UV-transparency of inland and coastal waters related to increases in inputs of DOM suggest that inactivation of parasites and pathogens by solar radiation is becoming progressively less effective.163 Outbreaks of water-borne pathogens are frequently preceded by high precipitation events.269 While mobilisation of pathogens from terrestrial ecosystems to inland waters is thought to account for a substantial portion of these outbreaks, the sensitivity of many pathogens to UV radiation coupled with reductions in solar disinfection related to decreases in the UV transparency of inland and coastal waters may also be a contributing factor.
A variety of environmental factors as well as the characteristics of parasites and pathogens may influence the effectiveness of solar disinfection by UV radiation in surface waters (see also section 5.5 below). Pathogenic bacteria270 and fungi271 are inactivated by natural and simulated solar radiation, and this inactivation may be reduced by a shading of UV radiation due to DOM and algal blooms.272 Hydrodynamic modeling based on data from Lake Tahoe indicates that variations in the depth of the thermocline and vertical mixing alter the exposure to UV radiation and are thus an important element in the effectiveness of the solar disinfection of parasites.273
In contrast to the UV-disinfection of surface waters, exposure to high levels of UV radiation can either stress or suppress the immune system of hosts, making them more susceptible to infection. For example, high levels of UV radiation combined with warm temperatures may increase the infection of freshwater fish by the protozoan parasite that causes white spot disease.274 Similarly, exposure of larval damselflies to UV radiation can suppress the immune response of adults, reducing their ability to defend against parasitic mites (see section 3.9 above).224
4.6 The effects and transport of pollutants in aquatic ecosystems are altered by UV radiation
Our general knowledge of the interactions between UV radiation and pollutants is limited because they have not been studied for many compounds. UV radiation can either enhance or reduce the effects of pollutants (see also section 5.5 below). Studies of the interaction of UV radiation and four toxic compounds on the growth and metabolism of algae revealed both synergistic and antagonistic effects.275 The mechanism of action of UV radiation may involve more than just photosynthesis. For example, the uptake and thus toxicity of copper in aquatic plants can be enhanced by exposure to UV radiation.276 Pesticides may also inhibit the repair of UV-induced DNA damage in embryos of African clawed frogs,277 as well as decrease the ability of their tadpoles to behaviourally avoid UV radiation.278
The toxic effects of oil spills are enhanced by UV radiation due to radical formation, alone or in combination with warming of the climate. In contrast, UV radiation has been shown to alleviate the negative effects of the combination of oil and ocean acidification on bacterial communities,279 and to play a role in photodegrading antibiotics in the environment.280
Mercury is an important aquatic pollutant and the toxicity and transport in the food web depends on the form of the mercury compound, with toxicity being especially severe for organic methylmercury. In nature there is a balance between the production of methylmercury and its breakdown by either bacterial or photochemical processes. Photochemical degradation of methylmercury is assumed to be the more dominant of these two pathways in lakes. UV-B radiation is the most active component of the solar spectrum in the photodegradation of methylmercury in Norwegian lakes;281 likewise in laboratory studies.282
Anthropogenic sunscreens (commercially produced sun lotions) contain a variety of chemicals that include organic compounds such as oxybenzone (benzophenone-3, or BP-3) that absorb damaging UV radiation, or inorganic compounds such as titanium dioxide or zinc oxide that reflect UV radiation. With the hundreds of millions of tourists that crowd beaches and use these sun lotions each year, there is the potential for these chemicals to accumulate to concentrations that may damage inland and coastal aquatic ecosystems. The organic compound BP-3 is toxic to coral planula larvae as well as to the cells of corals.283 When exposed to BP-3, even in the μg L−1 range, the larvae of coral lose their motility and their algal symbionts, and suffer substantial DNA and tissue damage, which leads to increased mortality. Since BP-3 is photo-toxic, these negative effects of BP-3 are worse in the sunlit waters where coral larvae are found.283 In the presence of solar UV radiation, titanium dioxide nanoparticles produce the strong oxidising agent hydrogen peroxide. While these nanoparticles are coated to prevent human skin damage in sun lotions, the coatings dissolve in natural waters and thus become more reactive. Combined laboratory experiments and field sampling at a beach in the Mediterranean Sea demonstrated that the levels of hydrogen peroxide produced in the presence of UV radiation by titanium dioxide in sun lotions can damage phytoplankton.284 These nanoparticles and other chemical compounds may concentrate in the surface microlayer where critical processes such as gas exchange and solar flux are regulated, as well as accumulate in the sediments where they may harm sediment-dwelling organisms.284,285 Other sun lotions release inorganic compounds that include not only toxins, but also phosphorus and nitrogen that are important nutrients that may increase primary productivity.285 While our knowledge of the effects of sun lotions on aquatic ecosystems is very limited, the available data suggest that the chemicals released by sun lotions may damage corals and alter phytoplankton dynamics, leading to negative effects on aquatic ecosystems and their food webs.
4.7 Several recent advances have been made in our understanding of how exposure to UV radiation can alter species interactions from phytoplankton to invertebrates and vertebrates in aquatic ecosystems, and also how some responses to UV radiation are temperature-dependent
Even closely related species may differ in their sensitivity to UV-B radiation owing to variation in the type and amounts of UV-absorbing compounds and detoxifying enzymes that they each have.286 Photoprotective defense mechanisms differ widely among species of phytoplankton287 and invertebrates.288 UV-induced changes in the composition of species at one trophic level can have consequences for other trophic levels. For example, concentrations of fatty acids in sub-Antarctic phytoplanktonic algae, important to grazing zooplankton, increase when these algae are grown without UV-B radiation.289
The role of UV radiation in the vision, communication, and performance of vertebrates is becoming more widely recognised. For example, it was recently found that hooded seals have enhanced vision in the UV wavelength range.290 The development of UV markings used in communication among coral reef fish is absent in captivity and is dependent on exposure to UV radiation on the reef.291 When exposed to UV radiation, spring-born mosquitofish reared at temperatures higher or lower than their 28 °C optimum exhibit decreases in swimming performance and higher metabolic rate accompanied by increased damage to proteins and lipids in their tail muscles. The responses of fish born later in the year are less temperature dependent.292 These new findings indicate that the effects of UV radiation are altered by the ability of aquatic organisms to detect, respond to, and defend against solar UV radiation, and that these responses can be temperature-dependent.
5 Biogeochemical cycles: the effects of solar UV radiation and interactions with climate change
5.1 Climate change could enhance the production of short-lived halogens that cause depletion of ozone in the stratosphere and troposphere
Short-lived halocarbons (with lifetimes <6 months; e.g., methylene chloride and bromoform) that cause depletion of ozone are produced in marine environments;293,294 for example, at the sea surface microlayer,295 and when terrestrial dissolved organic matter (DOM) is introduced into seawater.296 These short-lived brominated and iodinated halocarbons are formed via reactions associated with the natural breakdown of DOM in marine systems.297,298 Some of these products can reach the stratosphere where they participate in the destruction of ozone.298 Climate-induced increases in runoff of DOM from land to the ocean299 are likely to enhance the formation of these short-lived halocarbons with potentially important consequences for the depletion of ozone in both the stratosphere and troposphere.293,294
5.2 Ultraviolet radiation stimulates the production of carbon dioxide from terrestrial dissolved organic matter due to melting permafrost, and contributes to a significant positive feedback on global warming
Terrestrial permafrost contains nearly 1500 petagrams (1 Pg = 1015 g) of organic carbon.300,301 The release of CO2 from thawing permafrost in the Arctic is known to be increasing299 and is considered to be a major positive feedback in climate change by amplifying global warming.300 A range of processes is responsible for this release of CO2, but central in this change is the increased transport of terrestrial dissolved organic matter (DOM) into streams, rivers and lakes due to melting permafrost.250,301 Recent evidence has highlighted the role of solar radiation in the degradation of terrestrial DOM to produce CO2.250,251 In parallel, the mechanisms and organic precursors underlying the photodegradation of terrestrial DOM are becoming better understood.302,303 Photodegradation accounts for 70–95% of the total breakdown of DOM in Arctic lakes and rivers, and is therefore a major factor driving the release of CO2 from thawing permafrost.
5.3 Land-use and climate change alter vegetative cover and affect UV-mediated carbon turnover of plant litter in terrestrial ecosystems
Substantial differences in vegetative cover occur in terrestrial ecosystems due to influences from natural climatic factors coupled with human activities such as agriculture, deforestation, or the intentional planting of exotic species.304 Plant cover is a major determinant of the amount of UV radiation and total solar radiation reaching the soil surface,305 which means that plant cover modulates the effect of UV radiation on carbon turnover.306,307 Reductions in plant-cover due to decreased rainfall increase the direct photochemical degradation of carbon compounds in soil and may indirectly enhance microbial litter decomposition.196,199 Afforestation (the planting of woody species in areas that were previously unforested), which increases plant cover, dramatically reduces carbon loss and photodegradation in arid land ecosystems.305 In ecosystems with marked seasonality of rainfall, UV radiation in particular has been shown to stimulate litter decomposition (see section 3.4 above).192,194
5.4 Increases in the frequency, intensity, and extent of fires due to anthropogenic climate change and other human activities alter the effects of UV radiation on carbon cycling in terrestrial ecosystems
Estimates of the severity of extreme events highlight drought, and the consequent increased fire frequency, as potentially having the largest impact on the carbon cycle in the coming decades.308 Fires (Fig. 3) can interact with the effects of UV radiation on carbon cycling in a number of contrasting ways. The burning of above-ground biomass produces CO2, methane and other greenhouse gases and increases intercepted solar irradiance at the soil surface, all of which lead to increased fluxes of carbon into the atmosphere. In contrast, fires also generate short-lived aerosols that reduce UV and visible radiation and act as cloud-condensation nuclei (see sections 1.6 above and 6.8 below). Fires can increase diffuse radiation that would enhance the uptake of CO2 by plants.309 Charred biomass on the land surface includes a broad spectrum of organic constituents (including black carbon), which have different photoreactivities,310 and which can emit gaseous products from photodegradation. This charred organic matter can then run off into streams, lakes, and coastal inland waters, where photochemical mineralisation and biological degradation can occur. Taken together, the interaction of UV radiation and fires is complex, but suggests that an increased frequency of fires, particularly large-scale intense fires, will amplify the importance of UV-driven carbon losses due to changes in exposure and the characteristics of post-fire organic matter.
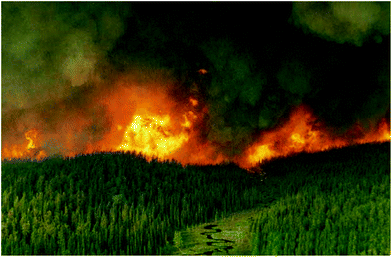 |
| Fig. 3 Climate change is leading to an increased frequency and extent of high-latitude fires that interact in various ways with changing UV radiation. Photo credit: Brian J. Stocks, Canadian Forest Service, Sault St. Marie, Ontario, Canada. | |
5.5 The fate and transport of pesticides, pharmaceuticals, heavy metals, nanomaterials, and pathogens are affected by UV-induced photochemical processes
UV radiation induces photoreactions that dissipate pollutants and pathogens. For example, protection from UV radiation by overlying plastics enhances the residues of some photosensitive pesticides in water and on crops.311,312 Pharmaceuticals, heavy metals, nanomaterials, organic pollutants, and pathogens enter aquatic environments where they are degraded through exposure to UV radiation. This photodegradation involves both direct photolysis and photoreactions sensitised by terrestrially-derived DOM189,313–316 (and see section 4.5 above). UV-initiated photoreactions play a key role in the release of nanomaterials from their composites with polymers in commercial products,317 carbon nanotubes,318 and graphene oxide.319 Future concentrations of certain environmental contaminants will thus depend on their release into the environment coupled with climate change events and photodegradation by UV radiation.
5.6 The production of methane and other trace gases from the foliage of a wide variety of plant species under realistic levels of ultraviolet radiation has been confirmed to be negligible for the global methane budget
Leaves from a wide range of species of plants were evaluated for emissions of greenhouse gases under environmentally relevant levels of UV-B radiation.320 All had measurable, but small, emissions of methane, carbon monoxide, and ethane. These fluxes are consistent with previously published studies,321 thus confirming that the global contribution of methane from this source is small (≤1 Teragram [1 Tg = 1012 g] y−1), representing less than 0.2% of global sources.320
6 Tropospheric air quality, composition, and processes: the effects of solar UV radiation and interactions with climate change
6.1 Ground-level ozone concentrations may increase substantially over large geographic regions due to a combination of stratospheric ozone recovery and climate change in the coming decades
Model simulations indicate that ground-level ozone would have decreased in northern mid-latitudes due to stratospheric ozone depletion, but this decrease was offset by changes in atmospheric circulation.322 As stratospheric ozone recovers, future background (non-polluted) ground-level ozone concentrations are expected to increase due to slower destruction by decreased UV radiation,323 and from increased transport of ozone from the stratosphere to ground-level. These increases in background ozone are likely to have negative implications for agriculture and ecosystem health. In addition, significant increases in ground-level ozone are expected due to increased storm-associated lightning,324 although the magnitude of this effect is uncertain due to a lack of understanding of the process for the production of nitrogen oxides (NOx) by lightning. These changes in ground-level ozone are in addition to any increases due to anthropogenic325 and biogenic emissions that are expected to be temperature-dependent.326 In urban areas, high emissions of NOx may counteract some of these effects and urban ozone concentrations may decrease.
6.2 Significant positive long-term trends in the concentrations of ozone at the Earth's surface have been observed over the last 25 years at most locations327
The exceptions are for Europe since 2000, and the east coast of the USA since the 1990s, where the trends were negative.327 In the Southern Hemisphere the increase was small but statistically significant. It remains unclear what is driving these trends. Model simulations for the west coast of the USA suggest that there has been an increase in ozone due to the export of NOx from Asia during 2005–2010, offsetting about half of the tropospheric column ozone reductions attained by reductions in local emissions.328 However, stratospheric-tropospheric ozone transport can also contribute to this trend.
6.3 An analysis of records of ground-level ozone in the UK concludes that the magnitude of estimated long-term trends depends strongly on the metrics used to quantify ozone patterns and amounts329
The trends depend on how ozone metrics are computed, e.g., from ozone peak levels, averages, or other algorithms (SOMO10, SOMO35).330 The choice of metric has implications for assessing the effectiveness of regulations and air quality improvements, and their relationship to background ozone concentrations that are beyond local/national regulation.
6.4 Increasing evidence indicates that the known sources and sinks of hydroxyl (˙OH) radicals are insufficient to explain concentrations of ˙OH actually observed or inferred in the atmosphere
UV-generated ˙OH radicals perform a critical function by accelerating the removal of many compounds from the atmosphere. Global observations of chemicals removed by ˙OH (e.g., methyl chloroform) indicate little or no difference in OH concentrations between the Northern and Southern Hemisphere, while models predict ∼25% more ˙OH in the Northern Hemisphere due to larger emissions of nitrogen oxides (NOx).331 Direct ˙OH measurements in locations with high amounts of biogenic hydrocarbons (e.g. isoprene) and low anthropogenic pollution (low NOx) also show larger amounts of ˙OH than predicted – likely produced through complicated radical recycling chemistry recently identified in laboratory experiments.332–336 Additional sources include the photolysis of ozone on the surface of cloud droplets337 and the reaction of methyl peroxy radicals with bromine monoxide.338 Measurements also show that the total rate of removal of ˙OH (the reactivity of ˙OH) is larger than predicted, further indicating the occurrence of recycling of ˙OH or other sources.339–343 Better quantification of the chemical reactions of ˙OH will improve our ability to predict future atmospheric compositions in response to changing emissions of critically important gases, e.g., methane, sulfur and nitrogen oxides, as well as HFCs and HCFCs.
6.5 Halogens contribute to the atmospheric oxidation (self-cleaning) capacity in marine environments, and new data suggest that they also contribute inland
Nitryl chloride (ClNO2) has been identified in the nocturnal atmosphere in a range of environments including over the coast and well inland. While stable in the dark, ClNO2 is photolysed at sunrise releasing Cl atoms that are significant oxidants at that time.344 Natural and anthropogenic emissions of short-lived halogens appear to supplement ˙OH in the atmospheric removal of critically important gases (methane, sulfur, and nitrogen oxides, HFCs and HCFCs; see section 5.6 above).
6.6 A new study demonstrates a clear linkage between the tropospheric oxidation capacity and stratospheric UV transmission even when modulated by factors other than ozone
Simulations of the 1991 eruption of Mt. Pinatubo confirmed that SO2 gas and sulfate particles injected into the stratosphere by the volcano caused a substantial reduction in tropospheric UV radiation, leading to lower ˙OH and higher methane concentrations over much of the globe for several months after the eruption.345
6.7 UV radiation is known to be a critical driver of the formation of photochemical smog, e.g. ozone and aerosols. UV radiation may also play a role in the destruction of aerosol particles
A new modeling study suggests that UV radiation also destroys some organic aerosols, a removal process which is comparable with hitherto recognised losses such as removal by rain.346 Such processes, if confirmed, could have a profound effect on the spatial and temporal distribution of aerosols, their effects on human health, and their response to UV radiation and other environmental changes.
6.8 Air pollution, which includes UV-generated ground-level ozone and particulate matter, is expected to worsen in many locations and improve in others, with direct consequences for human health
A recent global burden of disease study using a business-as-usual emission scenario projected that premature mortality due to outdoor air pollution could double by 2050, mostly in Asia.347 In the USA and EU, regulators have concluded that adverse health effects in some cases occur at air pollution concentrations lower than those of the currently established standards.348,349 However, recent studies have questioned the strength of the evidence supporting causal relationships between short- or long-term exposures to ozone, and e.g., cardiovascular effects.350–352 The problem with all of these analyses lies in their complexity: choices of databases, models and statistical methods can substantially affect the conclusions.353
6.9 Based on current data, the amount of trifluoroacetic acid (TFA) formed from HCFCs and HFCs in the troposphere is too small to be a risk to the health of humans and the environment
The fate and effects of TFA in the environment have been critically assessed.354 In summary, the formation of TFA from the degradation of HCFCs and HFCs continues to be a concern, in part because of its very long environmental lifetime. TFA is produced naturally and synthetically and is widely used in the chemical industry. In addition, TFA is a potential environmental breakdown product of a large number of chemicals (> one million), many of which are in commerce. These chemicals include pharmaceuticals, pesticides, and polymers. While the contribution to global amounts of TFA from HCFCs and HFCs is well understood, that from these other chemicals is very uncertain. TFA salts are stable in the environment and will accumulate in terminal sinks such as playas, salt lakes, and oceans where the only process for the loss of water is evaporation. The total contribution to the existing amounts of TFA in the oceans as a result of the continued use of HCFCs, HFCs, and HFOs up to 2050 was estimated to be a small fraction (<7.5%) of the ≈0.2 μg of acid equivalents per L estimated to be present at the start of the millennium. TFA, as an acid or as a salt, is of moderate to low toxicity to a range of tested organisms. Based on the worst-case exposure scenarios for the salts of TFA, risks to mammals, plants growing in soil, and aquatic organisms is de minimis. The risks are potentially greater for plants exposed directly to the acid form of TFA but the short times of exposure in most natural environments will likely mitigate adverse effects.
7 Materials: the effects of solar UV radiation and interactions with climate change
7.1 In addition to UV radiation, air pollutants in urban areas may contribute very significantly to the discoloration of plastics routinely exposed to outdoor conditions even in the absence of direct solar radiation
In the weathering of materials outdoors, solar UV radiation is generally the most important agent responsible for discoloration and the loss of mechanical strength.355 In a 2-year outdoor exposure study of 17 types of plastics, where direct solar radiation and precipitation were excluded, diffuse light and pollutants, especially NO2 and O3, were found to be the dominant secondary agents of damage. These were more important than temperature or relative humidity in causing the yellowing of plastics in art and heritage uses.356 The concentrations of traffic-generated air pollutants need to be taken into account in weathering studies, especially in urban areas.
7.2 Carbon black pigment, when used together with some UV stabilisers, enhances their stability synergistically
Carbon black (CB) is a good UV stabiliser in plastics357,358 as well as in rubber products. Recently, synergistic stabilisation of polyethylene using a combination of 1.5% CB and 0.1 to 0.2% (w/w) of the popular hindered-amine light stabilisers (HALS) was reported.359 In accelerated weathering studies indicators of weathering such as the gel content, carbonyl index, elastic modulus and elongation at break were the lowest for the HALS/CB system. The mechanism of the synergism has not as yet been fully elucidated. With dark-coloured plastic products, CB can be used to extend service lifetimes outdoors.
7.3 Polypropylenes containing recycled plastic show changes in bulk morphology that results in a reduced outdoor service life
Replacing a part of the virgin resin with recycled polypropylene (PP) in molding products is commonly practiced and is both economical and environmentally desirable. The mechanical properties of thick (>3 mm) molded PP with a recycled PP content of up to 40% are not very different from those of samples made from virgin PP. However, the outdoor durability of the former was shown to be much poorer when exposed to solar UV radiation, based on decreased mechanical properties,360 than that for virgin PP. Also, after 12 hours of accelerated UV irradiation, the decrease in toughness of molded laminates was larger by 13% for PP with a 30% recycled content compared to that for virgin PP. Despite the ‘green’ advantage of using a higher recycled content, at least for PP, it reduces the service life of the product.
7.4 In cable-jackets with the new aluminum-based fire retardants, initial degradation by UV radiation yields a filler-rich surface layer that screens the underlying polymer from further degradation
There is a growing trend in the industry to phase out polybrominated fire retardant additives. Ethylene vinyl-acetate co-polymer (EVA), with aluminum hydroxide (∼40% w/w) filler as the fire retardant, is used in cable jacketing. During exposure to UV radiation, the material undergoes heterogeneous degradation with the filler progressively migrating to the surface. This yields a protective surface layer that is enriched with aluminum oxide.361,362 Where the underlying material is thick enough to provide the needed mechanical integrity to the cable, this phenomenon provides incidental UV stability at no additional cost.
7.5 Some residual additives may enhance the degradation by UV radiation of light-sensitive polymer layers in organic photovoltaic cells
Emerging organic photovoltaic technology, including that of polymer photovoltaic (PV) cells, is expected to pose a strong challenge to the silicon counterparts. However, improving their durability to ensure a long service life (>20 years) is critical for their commercialisation. The mechanisms of photodegradation have been studied for the light-sensitive polymers in organic PV cells363–365 as well as their plastic encapsulants.366–368 In a recent report, residual additives left in the light-sensitive polymer during manufacturing, which are confined and sandwiched between plastic laminates, were shown to promote accelerated degradation of these polymers.369 Complete removal of these additives prior to sealing the light-sensitive layers during fabrication of the cells may help avoid this complication and extend service life.
7.6 Colour changes in wood exposed to solar UV radiation correlate well with the extent of lignin photodegradation
UV-induced degradation of the lignin in wood exposed to solar radiation results in yellowing.370 The surface yellowness index and the extent of lignin degradation (measured spectroscopically) during solar UV-induced degradation in several hardwood species were found to be correlated.371–373 Since colour measurements are more convenient, they could be used as a good surrogate for detecting changes in the surface chemistry of weathered wood.
7.7 Surface treatments, especially nanomaterial-based coatings, show continuing promise as UV stabilisers for wood
Nanomaterial UV stabilisers perform exceptionally well in a variety of wood species. The high specific surface area of nanoparticles provides efficient light-shielding at low weight fractions of the filler. Graphene,374 zirconium dioxide,374 iron oxide,375 titania,376 and cerium oxide377 can control UV-induced yellowing in several wood species. Similarly, surface modification of wood with nanocellulose crystals378 and epoxidised soybean oil379 also result in good UV stabilisation. There can be adverse environmental implications of the large-scale use of nanofiller technologies as the particles can be released during use. However, these approaches are yet to be developed for commercial use and the effects on the environment and human health from the potential release of these nanoparticles due to weathering are not yet known.
7.8 The efficacy of thermal surface modification of wood to control solar UV-induced damage remains unclear
Thermal modification of wood was developed as a means of improving the structural properties and biodegradation of wood outdoors. As the wood is used outdoors, how UV stability is affected by the heat treatment has been of recent interest. Two reports380,381 indicated an increase in the rate of discoloration and degradation of lignin in heat treated hard- and soft-wood species upon UV irradiation; others382,383 found the opposite. The stabilisation might be either species-specific or process-dependent. This is a promising environmentally-friendly technique for the protection from UV radiation of selected types of wood.
7.9 Modifying textile fibres with nanoparticles effectively improves their UV protective function
Nanoparticles used as fillers in textile fibres dramatically decrease the transmission of solar UV radiation. The incorporation of nanoparticulate titanium oxide,384 cerium oxide,377 and silver385,386 into natural textile fibres at <5% (w/w), lead to very significant reductions in the transmission of solar UV-A radiation through the fabrics. The service life of these treated fabrics is not reliably known (see section 2.11 above).
7.10 The protection against exposure to UV radiation provided by cotton fabrics can be increased by an order of magnitude through treatment with graphene nanoplates
Cotton fabric typically used in garments allows ∼15% of solar UV radiation to pass through.387 Good UV radiation absorbers coated on the fibre or fabric can significantly reduce this value. Surface modification of cotton fabric with graphene nanoplates increases the ultraviolet protection factor (UPF) 10-fold388 for 0.4% (w/w) graphene. Using a chitosan dispersant with 1% (w/w) graphene enhances it 60-fold,389 With the growth in graphene technology the technique has commercial potential. However, the thickness and weave of the fabric also strongly determines the level of protection from UV radiation. As is the case for fabrics treated with certain nanoparticles, the service life of these treated fabrics is not reliably known.
Acknowledgements
The Environmental Effects Assessment Panel gratefully acknowledges the following: Generous contributions by UNEP/Ozone Secretariat and the World Meteorological Organisation for the convened author meeting were much appreciated. Participation for the following authors is also acknowledged: Prof. Carlos Ballaré, Drs Pieter Aucamp, Amy Austin, Krishna Pandey, and Halim Redhwi were supported by UNEP. Prof. Sharon Robinson was supported by the Centre for Sustainable Ecosystem Solutions, University of Wollongong, and self-funded. Prof. Robyn Lucas was supported by the Australia National Health and Medical Research Council Career Development Fellowship, the Telethon Kids Institute and the Australian National University. Prof. Stephen Wilson was supported by the Centre for Atmospheric Chemistry, University of Wollongong. Prof. Lars Olof Björn was supported by the South China Normal University. Prof. Janet Bornman was supported by Curtin University, Western Australia. Prof. Alkiviadis Bais was supported by the Greek General Secretariat for Research and Technology (contract 100380/2014). Dr Kevin Rose was supported by the Rensselaer Polytechnic Institute. Prof. Donat-P Häder was supported by the Bundesministerium für Umwelt Naturschutz und Reaktorsicherheit. Drs Antony Andrady, Paul Barnes, Germar Bernhard, Janice Longstreth, Sasha Madronich, Craig Williamson were supported by the U.S. Global Change Research Program. PB was also supported by the U.S. National Science Foundation (DEB 0815897) and Loyola University J.H. Mullahy Endowment for Environmental Biology. GB was also supported by the U.S. National Science Foundation (ARC-1203250) and Biospherical Instruments Inc. CW was also supported by the U.S. National Science Foundation DEB-1360066. Prof. Nigel Paul was supported by the UK Department for Environment, Food and Rural Affairs (DEFRA), Dr Richard McKenzie was sponsored by the New Zealand Government's Ministry for the Environment through the Ministry of Business, Innovation and Employment's research contract C01X1008. Dr Rachel Neale was funded by a fellowship from the National Health and Medical Research Council. Prof. M. Shao was supported by the Natural Science Foundation of China for Outstanding Young Scholars (Grant No. 41125018). Prof. Keith Solomon's participation was self-funded. Prof. Yukio Takizawa's participation was self-funded. Dr Ayako Torikai's participation was self-funded. Prof. Sten-Åke Wängberg was supported by the Swedish Agency for Marine and Water Management. Dr Antony Young's participation was self-funded. Dr Richard Zepp was supported by the National Exposure Research Laboratory, Exposure Methods & Measurement Division, U.S. Environmental Protection Agency.
References
- M. P. Chipperfield, S. S. Dhomse, W. Feng, R. L. McKenzie, G. J. M. Velders and J. A. Pyle, Quantifying the ozone and ultraviolet benefits already achieved by the Montreal Protocol, Nat. Commun., 2015, 6 DOI:10.1038/ncomms8233.
- K. Eleftheratos, S. Kazadzis, C. S. Zerefos, K. Tourpali, C. Meleti, D. Balis, I. Zyrichidou, K. Lakkala, U. Feister, T. Koskela, A. Heikkila and J. M. Karhu, Ozone and spectroradiometric UV changes in the past 20 years over high latitudes, Atmos.-Ocean, 2015, 53, 117–125 CrossRef CAS.
- V. De Bock, H. De Backer, R. Van Malderen, A. Mangold and A. Delcloo, Relations between erythemal UV dose, global solar radiation, total ozone column and aerosol optical depth at Uccle, Belgium, Atmos. Chem. Phys., 2014, 14, 12251–12270 CrossRef.
- R. Román, J. Bilbao and A. de Miguel, Erythemal ultraviolet irradiation trends in the Iberian Peninsula from 1950 to 2011, Atmos. Chem. Phys., 2015, 15, 375–391 CrossRef.
- K. Fragkos, A. F. Bais, I. Fountoulakis, D. Balis, K. Tourpali, C. Meleti and P. Zanis, Extreme total column ozone events and effects on UV solar radiation at Thessaloniki, Greece, Theotet. Appl. Climatol., 2015, 1–13, DOI:10.1007/s00704-015-1562-3.
- M. Beckmann, T. Václavík, A. M. Manceur, L. Šprtová, H. von Wehrden, E. Welk and A. F. Cord, glUV: A global UV-B radiation data set for macroecological studies, Methods Ecol. Evolut., 2014, 5, 372–383 CrossRef.
- A. Damiani, R. R. Cordero, J. Carrasco, S. Watanabe, M. Kawamiya and V. E. Lagun, Changes in the UV Lambertian equivalent reflectivity in the Southern Ocean: Influence of sea ice and cloudiness, Rem. Sens. Environ., 2015, 169, 75–92 CrossRef.
- G. Norsang, Y.-C. Chen, N. Pingcuo, A. Dahlback, Ø. Frette, B. Kjeldstad, B. Hamre, K. Stamnes and J. J. Stamnes, Comparison of ground-based measurements of solar UV radiation at four sites on the Tibetan Plateau, Appl. Opt., 2014, 53, 736–747 CrossRef PubMed.
- D. Muyimbwa, A. Dahlback, T. Ssenyonga, Y.-C. Chen, J. J. Stamnes, Ø. Frette and B. Hamre, Validation of ozone monitoring instrument ultraviolet index against ground-based UV index in Kampala, Uganda, Appl. Opt., 2015, 54, 8537–8545 CrossRef PubMed.
- F. Zaratti, R. D. Piacentini, H. A. Guillen, S. H. Cabrera, J. Ben Liley and R. L. McKenzie, Proposal for a modification of the UVI risk scale, Photochem. Photobiol. Sci., 2014, 13, 980–985 CAS.
- D. Serrano, M. J. Marín, M. Núñez, M. P. Utrillas, S. Gandía and J. A. Martínez-Lozano, Wavelength dependence of the effective cloud optical depth, J. Atmos. Sol.-Terr. Phys., 2015, 130–131, 14–22 CrossRef.
- R. D. García, V. E. Cachorro, E. Cuevas, C. Toledano, A. Redondas, M. Blumthaler and Y. Benounna, Comparison of measured and modelled spectral UV irradiance at Izaña high mountain station: estimation of the underlying effective albedo, Int. J. Climatol., 2016, 36, 377–388 CrossRef.
- D. Mateos, G. Pace, D. Meloni, J. Bilbao, A. di Sarra, A. de Miguel, G. Casasanta and Q. Min, Observed influence of liquid cloud microphysical properties on ultraviolet surface radiation, J. Geophys. Res.: Atmos, 2014, 119, 2013JD020309 Search PubMed.
- J. Li, B. E. Carlson, O. Dubovik and A. A. Lacis, Recent trends in aerosol optical properties derived from AERONET measurements, Atmos. Chem. Phys., 2014, 14, 12271–12289 CAS.
- J. P. Putaud, F. Cavalli, S. Martins dos Santos and A. Dell'Acqua, Long-term trends in aerosol optical characteristics in the Po Valley, Italy, Atmos. Chem. Phys., 2014, 14, 9129–9136 CrossRef CAS.
- A. R. Attwood, R. A. Washenfelder, C. A. Brock, W. Hu, K. Baumann, P. Campuzano-Jost, D. A. Day, E. S. Edgerton, D. M. Murphy, B. B. Palm, A. McComiskey, N. L. Wagner, S. S. de Sá, A. Ortega, S. T. Martin, J. L. Jimenez and S. S. Brown, Trends in sulfate and organic aerosol mass in the Southeast U.S.: Impact on aerosol optical depth and radiative forcing, Geophys. Res. Lett., 2014, 41, 2014GL061669 CrossRef.
- D. Mateos, M. Antón, C. Toledano, V. E. Cachorro, L. Alados-Arboledas, M. Sorribas, M. J. Costa and J. M. Baldasano, Aerosol radiative effects in the ultraviolet, visible, and near-infrared spectral ranges using long-term aerosol data series over the Iberian Peninsula, Atmos. Chem. Phys., 2014, 14, 13497–13514 CrossRef CAS.
- H. Che, X. Y. Zhang, X. Xia, P. Goloub, B. Holben, H. Zhao, Y. Wang, X. C. Zhang, H. Wang, L. Blarel, B. Damiri, R. Zhang, X. Deng, Y. Ma, T. Wang, F. Geng, B. Qi, J. Zhu, J. Yu, Q. Chen and G. Shi, Ground-based aerosol climatology of China: aerosol optical depths from the China Aerosol Remote Sensing Network (CARSNET) 2002–2013, Atmos. Chem. Phys., 2015, 15, 7619–7652 CAS.
- C. M. Gan, J. Pleim, R. Mathur, C. Hogrefe, C. N. Long, J. Xing, S. Roselle and C. Wei, Assessment of the effect of air pollution controls on trends in shortwave radiation over the United States from 1995 through 2010 from multiple observation networks, Atmos. Chem. Phys., 2014, 14, 1701–1715 CAS.
- M. G. Tosca, J. T. Randerson and C. S. Zender, Global impact of smoke aerosols from landscape fires on climate and the Hadley circulation, Atmos. Chem. Phys., 2013, 13, 5227–5241 CAS.
- D. V. Spracklen, L. J. Mickley, J. A. Logan, R. C. Hudman, R. Yevich, M. D. Flannigan and A. L. Westerling, Impacts of climate change from 2000 to 2050 on wildfire activity and carbonaceous aerosol concentrations in the western United States, J. Geophys. Res.: Atmos, 2009, 114, D20301, DOI:20310.21029/22008JD010966.
- X. Yue, L. J. Mickley, J. A. Logan and J. O. Kaplan, Ensemble projections of wildfire activity and carbonaceous aerosol concentrations over the western United States in the mid-21st century, Atmos. Environ., 2013, 77, 767–780 CrossRef CAS PubMed.
- J. C. Péré, B. Bessagnet, V. Pont, M. Mallet and F. Minvielle, Influence of the aerosol solar extinction on photochemistry during the 2010 Russian wildfires episode, Atmos. Chem. Phys., 2015, 15, 10983–10998 CrossRef.
- I. Fountoulakis and A. F. Bais, Projected changes in erythemal and vitamin D effective irradiance over northern-hemisphere high latitudes, Photochem. Photobiol. Sci., 2015, 14, 1251–1264 CAS.
- M. D. Correa, S. Godin-Beekmann, M. Haeffelin, S. Bekki, P. Saiag, J. Badosa, F. Jegou, A. Pazmino and E. Mahe, Projected changes in clear-sky erythemal and vitamin D effective UV doses for Europe over the period 2006 to 2100, Photochem. Photobiol. Sci., 2013, 12, 1053–1064 CAS.
- T. Egorova, E. Rozanov, J. Grobner, M. Hauser and W. Schmutz, Montreal Protocol benefits simulated with CCM SOCOL, Atmos. Chem. Phys., 2013, 13, 3811–3823 CrossRef CAS.
- H. E. Rieder and L. M. Polvani, Are recent Arctic ozone losses caused by increasing greenhouse gases?, Geophys.
Res. Lett., 2013, 40, 4437–4441 CrossRef CAS.
- L. Sun, C. Deser, L. Polvani and R. Tomas, Influence of projected Arctic sea ice loss on polar stratospheric ozone and circulation in spring, Environ. Res. Lett., 2014, 9, 084016 CrossRef.
- A. Solomon, L. M. Polvani, K. L. Smith and R. P. Abernathey, The impact of ozone depleting substances on the circulation, temperature and salinity of the Southern Ocean: An attribution study with CESM1(WACCM), Geophys. Res. Lett., 2015, 42, 5547–5555 CrossRef.
- J. Bandoro, S. Solomon, A. Donohoe, D. W. J. Thompson and B. D. Santer, Influences of the Antarctic ozone hole on Southern Hemispheric summer climate change, J. Climate, 2014, 27, 6245–6264 CrossRef.
- D. Ferreira, J. Marshall, C. M. Bitz, S. Solomon and A. Plumb, Antarctic Ocean and sea ice response to ozone depletion: A two-time-scale problem, J. Climate, 2014, 28, 1206–1226 CrossRef.
- J. Zhang, W. Tian, F. Xie, Y. Li, F. Wang, J. Huang and H. Tian, Influence of the El Niño southern oscillation on the total ozone column and clear-sky ultraviolet radiation over China, Atmos. Environ., 2015, 120, 205–216 CrossRef CAS.
- P. Gies, R. Hooke, R. McKenzie, J. O'Hagan, S. Henderson, A. Pearson, M. Khazova, J. Javorniczky, K. King, M. Tully, M. Kotkamp, B. Forgan and S. Rhodes, International Intercomparison of Solar UVR Spectral Measurement Systems in Melbourne in 2013, Photochem. Photobiol., 2015, 91, 1237–1246 CrossRef CAS PubMed.
- L. Fan, W. Li, A. Dahlback, J. J. Stamnes, S. Stamnes and K. Stamnes, Long-term comparisons of UV index values derived from a NILU-UV instrument, NWS, and OMI in the New York area, Appl. Opt., 2015, 54, 1945–1951 CrossRef CAS PubMed.
- G. Bernhard, A. Arola, A. Dahlback, V. Fioletov, A. Heikkilä, B. Johnsen, T. Koskela, K. Lakkala, T. Svendby and J. Tamminen, Comparison of OMI UV observations with ground-based measurements at high northern latitudes, Atmos. Chem. Phys., 2015, 15, 7391–7412 CAS.
- J. Kujanpää and N. Kalakoski, Operational surface UV radiation product from GOME-2 and AVHRR/3 data, Atmos. Meas. Tech. Discuss., 2015, 8, 4537–4580 CrossRef.
- P. Setlow and L. Li, Photochemistry and photobiology of the spore photoproduct: A 50-year journey, Photochem. Photobiol., 2015, 91, 1263–1290 CrossRef CAS PubMed.
- A. M. Siani, G. R. Casale, S. Modesti, A. V. Parisi and A. Colosimo, Investigation on the capability of polysulphone for measuring biologically effective solar UV exposures, Photochem. Photobiol. Sci., 2014, 13, 521–530 CAS.
- G. R. Casale, M. Borra, A. Colosimo, M. Colucci, A. Militello, A. M. Siani and R. Sisto, Variability among polysulphone calibration curves, Phys. Med. Biol., 2006, 51, 4413–4427 CrossRef CAS PubMed.
- M.-A. Serrano, J. Canada, J. C. Moreno and G. Gurrea, Personal UV exposure for different outdoor sports, Photochem. Photobiol. Sci., 2014, 13, 671–679 CAS.
- G. Seckmeyer, S. Riechelmann, M. Schrempf, A. Stuhrmann and A. Niedzwiedz, Solar simulators for a healthy vitamin D synthesis, Anticancer Res., 2015, 35, 3607–3607 Search PubMed.
- A. Russell, M. Gohlan, A. Smedley and M. Densham, The ultraviolet radiation environment during an expedition across the Drake Passage and on the Antarctic Peninsula, Antarctic Sci., 2015, 27, 307–316 CrossRef.
- M. Grobner, J. Grobner and G. Hulsen, Quantifying UV exposure, vitamin D status and their relationship in a group of high school students in
an alpine environment, Photochem. Photobiol. Sci., 2015, 14, 352–357 CAS.
- U. Feister, G. Meyer, G. Laschewski and C. Boettcher, Validation of modeled daily erythemal exposure along tropical and subtropical shipping routes by ship-based and satellite-based measurements, J. Geophys. Res.: Atmos, 2015, 120, 4117–4131 Search PubMed.
- G. R. Casale, A. M. Siani, H. Diémoz, G. Agnesod, A. V. Parisi and A. Colosimo, Extreme UV index and solar exposures at Plateau Rosà (3500 m a.s.l.) in Valle d'Aosta Region, Italy, Sci. Total Environ., 2015, 512–513, 622–630 CrossRef CAS PubMed.
- V. Nurse, C. Y. Wright, M. Allen and R. L. McKenzie, Solar ultraviolet radiation exposure of South African marathon runners during competition marathon runs and training sessions: A feasibility study, Photochem. Photobiol., 2015, 91, 971–979 CrossRef CAS PubMed.
- D. M. Holman, Z. Berkowitz, G. P. Guy Jr., A. M. Hartman and F. M. Perna, The association between demographic and behavioral characteristics and sunburn among U.S. adults - National Health Interview Survey, 2010, Prevent. Med., 2014, 63, 6–12 CrossRef PubMed.
- C. H. Basch, C. E. Basch, S. Rajan and K. V. Ruggles, Use of sunscreen and indoor tanning devices among a nationally representative sample of high school students, 2001–2011, Prev. Chronic. Dis., 2014, 11, E144 Search PubMed.
- J. M. Satagopan, S. A. Oliveria, A. Arora, M. A. Marchetti, I. Orlow, S. W. Dusza, M. A. Weinstock, A. Scope, A. C. Geller, A. A. Marghoob and A. C. Halpern, Sunburn, sun exposure, and sun sensitivity in the Study of Nevi in Children, Ann. Epidemiol., 2015, 25, 839–843 CrossRef PubMed.
- Q. Gao, G. Liu and Y. Liu, Knowledge, attitude and practice regarding solar ultraviolet exposure among medical university students in Northeast China, J. Photochem. Photobiol., B, 2014, 140, 14–19 CrossRef CAS PubMed.
- S. Guo, P. Gies, K. King and R. M. Lucas, Sun exposure and vitamin D status as Northeast Asian migrants become acculturated to life in Australia, Photochem. Photobiol., 2014, 90, 1455–1461 CrossRef CAS PubMed.
- Cancer Research UK, Cancer Statistics Report: Cancer Incidence in the UK in 2011, Cancer Research UK Report No., 2014. http://publications.cancerresearchuk.org/downloads/product/CS_REPORT_INCIDENCE.pdf.
- Australian Institute of Health and Welfare, Australian Cancer Incidence and Mortality: melanoma of the skin, Australian Institute of Health and Welfare Report No., Canberra, 2015. http://www.aihw.gov.au/acim-books/.
- G. P. Guy Jr., C. C. Thomas, T. Thompson, M. Watson, G. M. Massetti, L. C. Richardson and C. Centers for Disease and Prevention, Vital signs: melanoma incidence and mortality trends and projections - United States, 1982–2030, MMWR Morb Mortal Wkly Rep, 2015, 64, 591–596 Search PubMed.
- A. D. Slade and M. T. Austin, Childhood melanoma: an increasingly important health problem in the USA, Curr. Opin. Pediatr., 2014, 26, 356–361 CrossRef PubMed.
- M. R. Iannacone, D. R. Youlden, P. D. Baade, J. F. Aitken and A. C. Green, Melanoma incidence trends and survival in adolescents and young adults in Queensland, Australia, Int. J. Cancer, 2015, 136, 603–609 CAS.
- S. C. Wallingford, M. R. Iannacone, D. R. Youlden, P. D. Baade, A. Ives, J. Verne, J. F. Aitken and A. C. Green, Comparison of melanoma incidence and trends among youth under 25 years in Australia and England, 1990–2010, Int. J. Cancer, 2015, 137, 2227–2233 CrossRef CAS PubMed.
- M. Arnold, C. Holterhues, L. M. Hollestein, J. W. Coebergh, T. Nijsten, E. Pukkala, B. Holleczek, L. Tryggvadottir, H. Comber, M. J. Bento, S. Diba Ch, R. Micallef, M. Primic-Zakelj, M. I. Izarzugaza, J. Perucha, R. Marcos-Gragera, J. Galceran, E. Ardanaz, R. Schaffar, A. Pring and E. de Vries, Trends in incidence and predictions of cutaneous melanoma across Europe up to 2015, J. Eur. Acad. Dermatol. Venereol., 2014, 28, 1170–1178 CrossRef CAS PubMed.
- F. Erdmann, J. Lortet-Tieulent, J. Schuz, H. Zeeb, R. Greinert, E. W. Breitbart and F. Bray, International trends in the incidence of malignant melanoma 1953–2008–are recent generations at higher or lower risk?, Int. J. Cancer, 2013, 132, 385–400 CrossRef CAS PubMed.
- R. Greinert, E. de Vries, F. Erdmann, C. Espina, A. Auvinen, A. Kesminiene and J. Schuz, European Code against Cancer 4th edition: Ultraviolet radiation and cancer, Cancer Epidemiol., Biomarkers Prev., 2015, 39, S75–S83 Search PubMed.
- P. Autier, A. Koechlin and M. Boniol, The forthcoming inexorable decline of cutaneous melanoma mortality in light-skinned populations, Eur. J. Cancer, 2015, 51, 869–878 CrossRef PubMed.
- L. W. Idorn, P. Datta, J. Heydenreich, P. A. Philipsen and H. C. Wulf, A 3-year follow-up of sun behavior in patients with cutaneous malignant melanoma, JAMA Dermatol., 2014, 150, 163–168 CrossRef PubMed.
- B. A. Glenn, T. Lin, L. C. Chang, A. Okada, W. K. Wong, K. Glanz and R. Bastani, Sun protection practices and sun exposure among children with a parental history of melanoma, Cancer Epidemiol., Biomarkers Prev., 2015, 24, 169–177 CrossRef PubMed.
- M. Berwick, B. K. Armstrong, L. Ben-Porat, J. Fine, A. Kricker, C. Eberle and R. Barnhill, Sun exposure and mortality from melanoma, J. Nat. Cancer Inst., 2005, 97, 195–199 CrossRef PubMed.
- A. Juzeniene, Z. Baturaite and J. Moan, Sun exposure and melanomas on sun-shielded and sun-exposed body areas, Adv. Exp. Med. Biol., 2014, 810, 375–389 Search PubMed.
- T. Bald, T. Quast, J. Landsberg, M. Rogava, N. Glodde, D. Lopez-Ramos, J. Kohlmeyer, S. Riesenberg, D. van den Boorn-Konijnenberg, C. Homig-Holzel, R. Reuten, B. Schadow, H. Weighardt, D. Wenzel, I. Helfrich, D. Schadendorf, W. Bloch, M. E. Bianchi, C. Lugassy, R. L. Barnhill, M. Koch, B. K. Fleischmann, I. Forster, W. Kastenmuller, W. Kolanus, M. Holzel, E. Gaffal and T. Tuting, Ultraviolet-radiation-induced inflammation promotes angiotropism and metastasis in melanoma, Nature, 2014, 507, 109–113 CrossRef CAS PubMed.
- H. W. Rogers, M. A. Weinstock, S. R. Feldman and B. M. Coldiron, Incidence estimate of nonmelanoma skin cancer (keratinocyte carcinomas) in the US population, 2012, JAMA Dermatol., 2015, 151, 1081–1086 CrossRef PubMed.
- S. Deady, L. Sharp and H. Comber, Increasing skin cancer incidence in young, affluent, urban populations: a challenge for prevention, Br. J. Dermatol., 2014, 171, 324–331 CrossRef CAS PubMed.
- J. Moan, M. Grigalavicius, Z. Baturaite, A. Dahlback and A. Juzeniene, The relationship between UV exposure and incidence of skin cancer, Photodermatol., Photoimmunol. Photomed., 2015, 31, 26–35 CrossRef PubMed.
- M. Norval, P. Kellett and C. Y. Wright, The incidence and body site of skin cancers in the population groups of South Africa, Photodermatol., Photoimmunol. Photomed., 2014, 30, 262–265 CrossRef PubMed.
- L. G. Gordon and D. Rowell, Health system costs of skin cancer and cost-effectiveness of skin cancer prevention and screening: a systematic review, Eur. J. Cancer Prev., 2015, 24, 141–149 CrossRef PubMed.
- C. Marionnet, C. Pierrard, C. Golebiewski and F. Bernerd, Diversity of biological effects induced by longwave UVA rays (UVA1) in reconstructed skin, PLoS One, 2014, 9, e105263 Search PubMed.
- E. Emanuele, J. M. Spencer and M. Braun, From DNA repair to proteome protection: new molecular insights for preventing non-melanoma skin cancers and skin aging, J. Drugs Dermatol., 2014, 13, 274–281 CAS.
- D. Karlica-Utrobicic, D. J. Batistic and M. Urlic, Changes in the eyelids and conjunctiva caused by ultraviolet radiation, Coll. Antropol., 2014, 38, 1111–1113 Search PubMed.
- S. Gichuhi, S. Ohnuma, M. S. Sagoo and M. J. Burton, Pathophysiology of ocular surface squamous neoplasia, Exp. Eye Res., 2014, 129, 172–182 CrossRef CAS PubMed.
- M. Stamenkovic, T. Radic, I. Stefanovic, V. Coric, I. Sencanic, M. Pljesa-Ercegovac, M. Matic, V. Jaksic, T. Simic and A. Savic-Radojevic, Glutathione S-transferase omega-2 polymorphism Asn142Asp modifies the risk of age-related cataract in smokers and subjects exposed to ultraviolet irradiation, Clin. Exp. Ophthalmol., 2014, 42, 277–283 Search PubMed.
- Y. Wang, F. Li, G. Zhang, L. Kang, B. Qin and H. Guan, Altered DNA methylation and expression profiles of 8-oxoguanine DNA glycosylase 1 in lens tissue from age-related cataract patients, Curr. Eye Res., 2015, 40, 815–821 CrossRef CAS PubMed.
- M. Zhu, J. Yu, Q. Gao, Y. Wang, L. Hu, Y. Zheng, F. Wang and Y. Liu, The relationship between disability-adjusted life years of cataracts and ambient erythemal ultraviolet radiation in China, J. Epidemiol., 2015, 25, 57–65 CrossRef PubMed.
- L. R. Pasquale, A. Z. Jiwani, T. Zehavi-Dorin, A. Majd, D. J. Rhee, T. Chen, A. Turalba, L. Shen, S. Brauner, C. Grosskreutz, M. Gardiner, S. Chen, S. Borboli-Gerogiannis, S. H. Greenstein, K. Chang, R. Ritch, S. Loomis, J. H. Kang, J. L. Wiggs and H. Levkovitch-Verbin, Solar exposure and residential geographic history in relation to exfoliation syndrome in the United States and Israel, JAMA Ophthalmol., 2014, 132, 1439–1445 CrossRef PubMed.
- P. Logan, M. Bernabeu, A. Ferreira and M. N. Burnier Jr., Evidence for the role of blue light in the development of uveal melanoma, J. Ophthalmol., 2015, 2015, 386986 Search PubMed.
- J. C. Yam and A. K. Kwok, Ultraviolet light and ocular diseases, Int. Ophthalmol., 2014, 34, 383–400 CrossRef PubMed.
- C. M. McKnight, J. C. Sherwin, S. Yazar, H. Forward, A. X. Tan, A. W. Hewitt, C. E. Pennell, I. L. McAllister, T. L. Young, M. T. Coroneo and D. A. Mackey, Myopia in young adults is inversely related to an objective marker of ocular sun exposure: the Western Australian Raine cohort study, Am. J. Ophthalmol., 2014, 158, 1079–1085 CrossRef PubMed.
- S. Yazar, A. W. Hewitt, L. J. Black, C. M. McKnight, J. A. Mountain, J. C. Sherwin, W. H. Oddy, M. T. Coroneo, R. M. Lucas and D. A. Mackey, Myopia is associated with lower vitamin D status in young adults, Invest. Ophthalmol. Visual Sci., 2014, 55, 4552–4559 Search PubMed.
- C. Ludema, S. R. Cole, C. Poole, J. S. Smith, V. J. Schoenbach and K. R. Wilhelmus, Association between unprotected ultraviolet radiation exposure and recurrence of ocular herpes simplex virus, Am. J. Epidemiol., 2014, 179, 208–215 CrossRef PubMed.
- H. S. Jung, J. K. Kang and S. H. Yoo, Epidemiological study on the incidence of Herpes zoster in nearby Cheonan, Korean J. Pain, 2015, 28, 193–197 CrossRef PubMed.
- P. Y. Wu, H. D. Wu, T. C. Chou and F. C. Sung, Varicella vaccination alters the chronological trends of herpes zoster and varicella, PLoS One, 2013, 8, e77709 CAS.
- P. Mangtani, I. Abubakar, C. Ariti, R. Beynon, L. Pimpin, P. E. Fine, L. C. Rodrigues, P. G. Smith, M. Lipman, P. F. Whiting and J. A. Sterne, Protection by BCG vaccine against tuberculosis: a systematic review of randomized controlled trials, Clin. Infect. Dis., 2014, 58, 470–480 CrossRef PubMed.
- G. C. Koh, G. Hawthorne, A. M. Turner, H. Kunst and M. Dedicoat, Tuberculosis incidence correlates with sunshine: an ecological 28-year time series study, PLoS One, 2013, 8, e57752 CAS.
- D. Mukhopadhyay, J. E. Dalton, P. M. Kaye and M. Chatterjee, Post kala-azar dermal leishmaniasis: an unresolved mystery, Trends Parasitol., 2014, 30, 65–74 CrossRef PubMed.
- D. E. Godar, R. Tang and S. J. Merrill, Pharyngeal and cervical cancer incidences significantly correlate with personal UV doses among whites in the United States, Anticancer Res., 2014, 34, 4993–4999 Search PubMed.
- P. Autier, M. Boniol, C. Pizot and P. Mullie, Vitamin D status and ill health: a systematic review, Lancet Diabetes Endocrinol., 2014, 2, 76–89 CrossRef CAS PubMed.
- J. Hilger, A. Friedel, R. Herr, T. Rausch, F. Roos, D. A. Wahl, D. D. Pierroz, P. Weber and K. Hoffmann, A systematic review of vitamin D status in populations worldwide, Br. J. Nutr., 2014, 111, 23–45 CrossRef CAS PubMed.
- C. Palacios and L. Gonzalez, Is vitamin D deficiency a major global public health problem?, J. Steriod Biochem. Mol. Biol., 2014, 144PA, 138–145 CrossRef PubMed.
- R. Kelishadi, R. Moeini, P. Poursafa, S. Farajian, H. Yousefy and A. A. Okhovat-Souraki, Independent association between air pollutants and vitamin D deficiency in young children in Isfahan, Iran, Paediatr. Int. Child Health, 2014, 34, 50–55 CrossRef PubMed.
- A. R. Webb and O. Engelsen, Ultraviolet exposure scenarios: risks of erythema from recommendations on cutaneous vitamin D synthesis, Adv. Exp. Med. Biol., 2014, 810, 406–422 Search PubMed.
- S. Durvasula, P. Gies, R. S. Mason, J. S. Chen, S. Henderson, M. J. Seibel, P. N. Sambrook, L. M. March, S. R. Lord, C. Kok, M. Macara, T. R. Parmenter and I. D. Cameron, Vitamin D response of older people in residential aged care to sunlight-derived ultraviolet radiation, Arch. Osteoporos, 2014, 9, 197 CrossRef PubMed.
- E. Theodoratou, I. Tzoulaki, L. Zgaga and J. P. Ioannidis, Vitamin D and multiple health outcomes: umbrella review of systematic reviews and meta-analyses of observational studies and randomised trials, BMJ, 2014, 348, g2035 CrossRef PubMed.
- K. S. Yap, M. Northcott, A. B. Hoi, E. F. Morand and M. Nikpour, Association of low vitamin D with high disease activity in an Australian systemic lupus erythematosus cohort, Lupus Sci. Med., 2015, 2, e000064 CrossRef CAS PubMed.
- E. Cutillas-Marco, A. Marquina-Vila, W. Grant, J. Vilata-Corell and M. Morales-Suarez-Varela, Vitamin D and cutaneous lupus erythematosus: effect of vitamin D replacement on disease severity, Lupus, 2014, 23, 615–623 CrossRef CAS PubMed.
- C. Lu, J. Yang, W. Yu, D. Li, Z. Xiang, Y. Lin and C. Yu, Association between 25(OH)D Level, ultraviolet exposure, geographical location, and inflammatory bowel disease activity: A systematic review and meta-analysis, PLoS One, 2015, 10, e0132036 Search PubMed.
- A. Ascherio, K. L. Munger, R. White, K. Kochert, K. C. Simon, C. H. Polman, M. S. Freedman, H. P. Hartung, D. H. Miller, X. Montalban, G. Edan, F. Barkhof, D. Pleimes, E. W. Radu, R. Sandbrink, L. Kappos and C. Pohl, Vitamin D as an early predictor of multiple sclerosis activity and progression, JAMA Neurol., 2014, 71, 306–314 CrossRef PubMed.
- R. Jorde, E. B. Mathiesen, S. Rogne, T. Wilsgaard, M. Kjaergaard, G. Grimnes and H. Schirmer, Vitamin D and cognitive function: The Tromso Study, J. Neurol. Sci., 2015, 355, 155–161 CrossRef CAS PubMed.
- M. Kamal, A. Bener and M. S. Ehlayel, Is high prevalence of vitamin D deficiency a correlate for attention deficit hyperactivity disorder?, Atten Defic Hyperact Disord, 2014, 6, 73–78 CrossRef PubMed.
- S. B. Goksugur, A. E. Tufan, M. Semiz, C. Gunes, M. Bekdas, M. Tosun and F. Demircioglu, Vitamin D status in children with attention-deficit-hyperactivity disorder, Pediatr. Int., 2014, 56, 515–519 CrossRef CAS PubMed.
- R. M. Lucas, A. L. Ponsonby, K. Dear, P. C. Valery, B. Taylor, I. van der Mei, A. J. McMichael, M. P. Pender, C. Chapman, A. Coulthard, T. J. Kilpatrick, J. Stankovich, D. Williams and T. Dwyer, Vitamin D status: multifactorial contribution of environment, genes and other factors in healthy Australian adults across a latitude gradient, J. Steroid Biochem. Mol. Biol., 2013, 136, 300–308 CrossRef CAS PubMed.
- K. Saad, A. A. Abdel-Rahman, Y. M. Elserogy, A. A. Al-Atram, J. J. Cannell, G. Bjorklund, M. K. Abdel-Reheim, H. A. Othman, A. A. El-Houfey, N. H. Abd El-Aziz, K. A. Abd El-Baseer, A. E. Ahmed and A. M. Ali, Vitamin D status in autism spectrum disorders and the efficacy of vitamin D supplementation in autistic children, Nutr. Neurosci., 2015 DOI:10.1179/1476830515Y.0000000019.
- E. Morales, J. Julvez, M. Torrent, F. Ballester, C. L. Rodriguez-Bernal, A. Andiarena, O. Vegas, A. M. Castilla, C. Rodriguez-Dehli, A. Tardon and J. Sunyer, Vitamin D in pregnancy and attention deficit hyperactivity disorder-like symptoms in childhood, Epidemiology, 2015, 26, 458–465 CrossRef PubMed.
- N. Baiz, P. Dargent-Molina, J. D. Wark, J. C. Souberbielle, I. Annesi-Maesano and Eden Mother-Child Cohort Study Group, Cord serum 25-hydroxyvitamin D and risk of early childhood transient wheezing and atopic dermatitis, J. Allergy Clin. Immunol., 2014, 133, 147–153 CrossRef CAS PubMed.
- M. Strom, T. I. Halldorsson, S. Hansen, C. Granstrom, E. Maslova, S. B. Petersen, A. S. Cohen and S. F. Olsen, Vitamin D measured in maternal serum and offspring neurodevelopmental outcomes: a prospective study with long-term follow-up, Ann. Nutr. Metab., 2014, 64, 254–261 CrossRef CAS PubMed.
- K. Amrein, S. A. Quraishi, A. A. Litonjua, F. K. Gibbons, T. R. Pieber, C. A. Camargo Jr., E. Giovannucci and K. B. Christopher, Evidence for a U-shaped relationship between prehospital vitamin D status and mortality: a cohort study, J. Clin. Endocrinol. Metab., 2014, 99, 1461–1469 CrossRef CAS PubMed.
- Y. Xu, X. Shao, Y. Yao, L. Xu, L. Chang, Z. Jiang and Z. Lin, Positive association between circulating 25-hydroxyvitamin D levels and prostate cancer risk: new findings from an updated meta-analysis, J. Cancer Res. Clin. Oncol., 2014, 140, 1465–1477 CrossRef CAS PubMed.
- L. Ke, R. S. Mason, M. Kariuki, E. Mpofu and K. E. Brock, Vitamin D status and hypertension: a review, Integr. Blood Press. Control, 2015, 8, 13–35 Search PubMed.
- A. W. Turunen, A. L. Suominen, H. Kiviranta, P. K. Verkasalo and E. Pukkala, Cancer incidence in a cohort with high fish consumption, Cancer Cause Control, 2014, 25, 1595–1602 CrossRef PubMed.
- E. K. Cahoon, R. M. Pfeiffer, D. C. Wheeler, J. Arhancet, S. W. Lin, B. H. Alexander, M. S. Linet and D. M. Freedman, Relationship between ambient ultraviolet radiation and non-Hodgkin lymphoma subtypes: a U.S. population-based study of racial and ethnic groups, Int. J. Cancer, 2015, 136, E432–E441 CrossRef CAS PubMed.
- S. B. Mohr, E. D. Gorham, C. F. Garland, W. B. Grant, F. C. Garland and R. E. Cuomo, Are low ultraviolet B and vitamin D associated with higher incidence of multiple myeloma?, J. Steroid Biochem. Mol. Biol., 2015, 148, 245–252 CrossRef CAS PubMed.
- A. Coste, S. Goujon, M. Boniol, F. Marquant, L. Faure, J. F. Dore, D. Hemon and J. Clavel, Residential exposure to solar ultraviolet radiation and incidence of childhood hematological malignancies in France, Cancer Cause Control, 2015, 26, 1339–1349 CrossRef PubMed.
- P. Jantchou, F. Clavel-Chapelon, A. Racine, M. Kvaskoff, F. Carbonnel and M. C. Boutron-Ruault, High residential sun exposure is associated with a low risk of incident Crohn's disease in the prospective E3N cohort, Inflamm. Bowel Dis., 2014, 20, 75–81 CrossRef PubMed.
- B. N. Limketkai, T. M. Bayless, S. R. Brant and S. M. Hutfless, Lower regional and temporal ultraviolet exposure is associated with increased rates and severity of inflammatory bowel disease hospitalisation, Aliment. Pharmacol. Ther., 2014, 40, 508–517 CrossRef CAS PubMed.
- T. Spelman, O. Gray, M. Trojano, T. Petersen, G. Izquierdo, A. Lugaresi, R. Hupperts, R. Bergamaschi, P. Duquette, P. Grammond, G. Giuliani, C. Boz, F. Verheul, C. Oreja-Guevara, M. Barnett, F. Grand'Maison, M. Edite Rio, J. Lechner-Scott, V. Van Pesch, R. Fernandez Bolanos, S. Flechter, L. Den Braber-Moerland, G. Iuliano, M. P. Amato, M. Slee, E. Cristiano, M. L. Saladino, M. Paine, N. Vella, K. Kasa, N. Deri, J. Herbert, F. Moore, T. Petkovska-Boskova, R. Alroughani, A. Savino, C. Shaw, S. Vucic, V. Santiago, E. A. Bacile, E. Skromne, D. Poehlau, J. A. Cabrera-Gomez, R. Lucas and H. Butzkueven, Seasonal variation of relapse rate in multiple sclerosis is latitude dependent, Ann. Neurol., 2014, 76, 880–890 CrossRef PubMed.
- R. Hoyos-Bachiloglu, P. S. Morales, J. Cerda, E. Talesnik, G. Gonzalez, C. A. Camargo Jr. and A. Borzutzky, Higher latitude and lower solar radiation influence on anaphylaxis in Chilean children, Pediatr. Allergy Immu., 2014, 25, 338–343 CrossRef PubMed.
- B. B. Shapiro, E. Streja, J. L. Chen, C. P. Kovesdy, K. Kalantar-Zadeh and C. M. Rhee, The relationship between ultraviolet light exposure and mortality in dialysis patients, Am. J. Nephrol., 2014, 40, 224–232 CrossRef PubMed.
- P. G. Lindqvist, E. Epstein, M. Landin-Olsson, C. Ingvar, K. Nielsen, M. Stenbeck and H. Olsson, Avoidance of sun exposure is a risk factor for all-cause mortality: results from the melanoma in southern Sweden cohort, J. Int. Med., 2014, 276, 77–86 CrossRef CAS PubMed.
- A. A. Salas, K. A. Smith, M. D. Rodgers, V. Phillips and N. Ambalavanan, Seasonal variation in solar ultraviolet radiation and early mortality in extremely preterm infants, Am. J. Perinatol., 2015, 32, 1273–1276 CrossRef PubMed.
- E. Viola, A. Coggiola Pittoni, A. Drahos, U. Moretti and A. Conforti, Photosensitivity with angiotensin II receptor blockers: A retrospective
study using data from VigiBase, Drug Saf., 2015, 38, 889–894 CrossRef CAS PubMed.
- S. A. Schmidt, M. Schmidt, F. Mehnert, S. Lemeshow and H. T. Sorensen, Use of antihypertensive drugs and risk of skin cancer, J. Eur. Acad. Dermatol. Venereol., 2015, 29, 1545–1554 CrossRef CAS PubMed.
- L. Z. Hasoun, S. W. Bailey, K. K. Outlaw and J. E. Ayling, Rearrangement and depletion of folate in human skin by ultraviolet radiation, Br. J. Dermatol., 2015, 173(4), 1087–1090 CrossRef CAS PubMed.
- D. Borradale, E. Isenring, E. Hacker and M. G. Kimlin, Exposure to solar ultraviolet radiation is associated with a decreased folate status in women of childbearing age, J. Photochem. Photobiol., B, 2014, 131, 90–95 CrossRef CAS PubMed.
- K. Butzbach and B. Epe, Photogenotoxicity of folic acid, Free Radicals Biol. Med., 2013, 65, 821–827 CrossRef CAS PubMed.
- M. Donnenfeld, M. Deschasaux, P. Latino-Martel, A. Diallo, P. Galan, S. Hercberg, K. Ezzedine and M. Touvier, Prospective association between dietary folate intake and skin cancer risk: results from the Supplementation en Vitamines et Mineraux Antioxydants cohort, Am. J. Clin. Nutr., 2015, 102, 471–478 CrossRef CAS PubMed.
- D. M. Holman, Z. Berkowitz, G. P. Guy Jr., N. A. Hawkins, M. Saraiya and M. Watson, Patterns of sunscreen use on the face and other exposed skin among US adults, J. Am. Acad. Dermatol., 2015, 73, 83–92 CrossRef PubMed.
- U. Sattler, S. Thellier, V. Sibaud, C. Taieb, S. Mery, C. Paul and N. Meyer, Factors associated with sun protection compliance: results from a nationwide cross-sectional evaluation of 2215 patients from a dermatological consultation, Br. J. Dermatol., 2014, 170, 1327–1335 CrossRef CAS PubMed.
- H. I. Mahler, Reasons for using and failing to use sunscreen: comparison among whites, Hispanics, and Asian/Pacific Islanders in Southern California, JAMA Dermatol., 2014, 150, 90–91 CrossRef PubMed.
- B. Y. Kong, S. L. Sheu and R. V. Kundu, Assessment of consumer knowledge of new sunscreen labels, JAMA Dermatol., 2015, 151, 1028–1030 CrossRef PubMed.
- B. Petersen and H. C. Wulf, Application of sunscreen–theory and reality, Photodermatol., Photoimmunol. Photomed., 2014, 30, 96–101 CrossRef PubMed.
- A. Bauer, K. Hault, A. Puschel, H. Ronsch, P. Knuschke and S. Beissert, Acceptance and usability of different sunscreen formulations among outdoor workers: a randomized, single-blind, cross-over study, Acta Derm.-Venereol., 2014, 94, 152–156 CrossRef PubMed.
- M. Jeanmougin, A. Bouloc and J. L. Schmutz, A new sunscreen application technique to protect more efficiently from ultraviolet radiation, Photodermatol., Photoimmunol. Photomed., 2014, 30, 323–331 CrossRef PubMed.
- A. Fourtanier, D. Moyal and S. Seite, UVA filters in sun-protection products: regulatory and biological aspects, Photochem. Photobiol. Sci., 2012, 11, 81–89 CAS.
- M. R. Iannacone, M. C. Hughes and A. C. Green, Effects of sunscreen on skin cancer and photoaging, Photodermatol., Photoimmunol. Photomed., 2014, 30, 55–61 CrossRef PubMed.
- R. Ghiasvand, E. Lund, K. Edvardsen, E. Weiderpass and M. B. Veierod, Prevalence and trends of sunscreen use and sunburn among Norwegian women, Br. J. Dermatol., 2015, 172, 475–483 CrossRef CAS PubMed.
- F. Xie, T. Xie, Q. Song, S. Xia and H. Li, Analysis of association between sunscreens use and risk of malignant melanoma, Int. J. Clin. Exp. Med., 2015, 8, 2378–2384 Search PubMed.
- A. C. Chen, D. L. Damian and G. M. Halliday, Oral and systemic photoprotection, Photodermatol., Photoimmunol. Photomed., 2014, 30, 102–111 CrossRef CAS PubMed.
- S. M. Pilkington, N. K. Gibbs, P. S. Friedmann and L. E. Rhodes, Nutritional abrogation of photoimmunosuppression: in vivo investigations, Photodermatol., Photoimmunol.
Photomed., 2014, 30, 112–127 CrossRef CAS PubMed.
- N. Saewan and A. Jimtaisong, Natural products as photoprotection, J. Cosmet. Dermatol., 2015, 14, 47–63 CrossRef PubMed.
- R. Calo and L. Marabini, Protective effect of Vaccinium myrtillus extract against UVA- and UVB-induced damage in a human keratinocyte cell line (HaCaT cells), J. Photochem. Photobiol., B, 2014, 132, 27–35 CrossRef CAS PubMed.
- I. F. Almeida, J. Maleckova, R. Saffi, H. Monteiro, F. Goios, M. H. Amaral, P. C. Costa, J. Garrido, P. Silva, N. Pestana and M. F. Bahia, Characterization of an antioxidant surfactant-free topical formulation containing Castanea sativa leaf extract, Drug Dev. Ind. Pharm., 2015, 41, 148–155 CrossRef CAS PubMed.
- I. F. Almeida, A. S. Pinto, C. Monteiro, H. Monteiro, L. Belo, J. Fernandes, A. R. Bento, T. L. Duarte, J. Garrido, M. F. Bahia, J. M. Sousa Lobo and P. C. Costa, Protective effect of C. sativa leaf extract against UV mediated-DNA damage in a human keratinocyte cell line, J. Photochem. Photobiol., B, 2015, 144, 28–34 CrossRef CAS PubMed.
- A. Hartmann, J. Gostner, J. E. Fuchs, E. Chaita, N. Aligiannis, L. Skaltsounis and M. Ganzera, Inhibition of collagenase by mycosporine-like amino cids afrom marine sources, Planta Med., 2015, 81, 813–820 CrossRef CAS PubMed.
- A. Hartmann, A. Holzinger, M. Ganzera and U. Karsten, Prasiolin, a new UV-sunscreen compound in the terrestrial green macroalga Prasiola calophylla (Carmichael ex Greville) Kutzing (Trebouxiophyceae, Chlorophyta), Planta, 2015, 243, 161–169 CrossRef PubMed.
- A. R. Osborn, K. H. Almabruk, G. Holzwarth, S. Asamizu, J. LaDu, K. M. Kean, P. A. Karplus, R. L. Tanguay, A. T. Bakalinsky and T. Mahmud, De novo synthesis of a sunscreen compound in vertebrates, eLife, 2015, 4, e05919 Search PubMed.
- R. P. Rastogi, R. R. Sonani and D. Madamwar, Cyanobacterial sunscreen scytonemin: role in photoprotection and biomedical research, Appl. Biochem. Biotechnol., 2015, 176, 1551–1563 CrossRef CAS PubMed.
- A. R. Heurung, S. I. Raju and E. M. Warshaw, Adverse reactions to sunscreen agents: epidemiology, responsible irritants and allergens, clinical characteristics, and management, Dermatitis, 2014, 25, 289–326 CrossRef CAS PubMed.
- A. C. de Groot and D. W. Roberts, Contact and photocontact allergy to octocrylene: a review, Contact Dermatitis, 2014, 70, 193–204 CrossRef PubMed.
- S. Maipas and P. Nicolopoulou-Stamati, Sun lotion chemicals as endocrine disruptors, Hormones, 2015, 14, 32–46 Search PubMed.
- F. Behar-Cohen, G. Baillet, T. de Ayguavives, P. O. Garcia, J. Krutmann, P. Pena-Garcia, C. Reme and J. S. Wolffsohn, Ultraviolet damage to the eye revisited: eye-sun protection factor (E-SPF(R)), a new ultraviolet protection label for eyewear, Clin. Ophthalmol., 2014, 8, 87–104 Search PubMed.
- J. Krutmann, F. Behar-Cohen, G. Baillet, T. de Ayguavives, P. Ortega Garcia, P. Pena-Garcia, C. Reme and J. Wolffsohn, Towards standardization of UV eye protection: what can be learned from photodermatology?, Photodermatol., Photoimmunol. Photomed., 2014, 30, 128–136 CrossRef PubMed.
- N. Bazzazi, S. Heydarian, R. Vahabi, S. Akbarzadeh and D. F. Fouladi, Quality of sunglasses available in the Iranian market; a study with emphasis on sellers’ license, Indian J. Ophthalmol., 2015, 63, 152–156 CrossRef PubMed.
- S. Rahmani, M. Mohammadi Nia, A. Akbarzadeh Baghban, M. R. Nazari and M. Ghassemi-Broumand, Spectral transmittance of UV-blocking soft contact lenses: a comparative study, Cont. Lens. Anterior Eye, 2014, 37, 451–454 CrossRef PubMed.
- J.
S. Wolffsohn, T. Drew and A. Sulley, Conjunctival UV autofluorescence–prevalence and risk factors, Cont. Lens. Anterior Eye, 2014, 37, 427–430 CrossRef PubMed.
- G. Tetreau, A. Chandor-Proust, F. Faucon, R. Stalinski, I. Akhouayri, S. M. Prud'homme, M. Regent-Kloeckner, M. Raveton and S. Reynaud, UV light and urban pollution: bad cocktail for mosquitoes?, Aquat. Toxicol., 2014, 146, 52–60 CrossRef CAS PubMed.
- M. D. Freedman, C. M. Kitahara, M. S. Linet, B. H. Alexander, G. Neta, M. P. Little and E. K. Cahoon, Ambient temperature and risk of first primary basal cell carcinoma: A nationwide United States cohort study, J. Photochem. Photobiol., B, 2015, 148, 284–289 CrossRef PubMed.
- F. Xiang, S. Harrison, M. Nowak, M. Kimlin, I. Van der Mei, R. E. Neale, C. Sinclair, R. M. Lucas and D. S. I. G. Aus, Weekend personal ultraviolet radiation exposure in four cities in Australia: influence of temperature, humidity and ambient ultraviolet radiation, J. Photochem. Photobiol., B, 2015, 143, 74–81 CrossRef CAS PubMed.
- C. L. Ballaré, C. A. Mazza, A. T. Austin and R. Pierik, Canopy light and plant health, Plant Physiol., 2012, 160, 145–155 CrossRef PubMed.
- C. E. Williamson, R. G. Zepp, R. M. Lucas, S. Madronich, A. T. Austin, C. L. Ballare, M. Norval, B. Sulzberger, A. F. Bais, R. L. McKenzie, S. A. Robinson, D.-P. Haeder, N. D. Paul and J. F. Bornman, Solar ultraviolet radiation in a changing climate, Nat. Clim. Change, 2014, 4, 434–441 CrossRef.
- M. Anwar, D. Liu, I. Macadam and G. Kelly, Adapting agriculture to climate change: a review, Theoret. Appl. Climatol., 2013, 113, 225–245 CrossRef.
- S. K. Singh, K. R. Reddy, V. R. Reddy and W. Gao, Maize growth and developmental responses to temperature and ultraviolet-B radiation interaction, Photosynthetica, 2014, 52, 262–271 CrossRef CAS.
- U. Sivadasan, T. R. Randriamanana, R. Julkunen-Tiitto and L. Nybakken, The vegetative buds of Salix myrsinifolia are responsive to elevated UV-B and temperature, Plant Physiol. Biochem., 2015, 93, 66–73 CrossRef CAS PubMed.
- L. Llorens, F. Ruben Badenes-Perez, R. Julkunen-Tiitto, C. Zidorn, A. Fereres and M. A. K. Jansen, The role of UV-B radiation in plant sexual reproduction, Perspect. Plant Ecol. Evolution Systematics, 2015, 17, 243–254 CrossRef.
- C. A. Mazza and C. L. Ballare, Photoreceptors UVR8 and phytochrome B cooperate to optimize plant growth and defense in patchy canopies, New Phytol., 2015, 207, 4–9 CrossRef PubMed.
- J. A. Zavala, C. A. Mazza, F. M. Dillon, H. D. Chludil and C. L. Ballaré, Soybean resistance to stink bugs (Nezara viridula and Piezodorus guildinii) increases with exposure to solar UV-B radiation and correlates with isoflavonoid content in pods under field conditions, Plant, Cell Environ., 2015, 38, 920–928 CrossRef CAS PubMed.
-
D. Crosby, Experimental approaches to pesticide photodecomposition, in Residues of Pesticides and Other Foreign Chemicals in Foods and Feeds, ed. F. Gunther, Springer, New York, NY, USA, 1969, pp. 1–12 Search PubMed.
- S. Rani and D. Sud, Role of enhanced solar radiation for degradation of triazophos pesticide in soil matrix, Solar Energy, 2015, 120, 494–504 CrossRef CAS.
- M. P. R. Lima, A. M. V. M. Soares and S. Loureiro, Responses of wheat (Triticum aestivum) and turnip (Brassica rapa) to the combined exposure of carbaryl and ultraviolet radiation, Environ. Toxicol. Chem., 2015, 34, 1665–1674 CrossRef CAS PubMed.
- S. B. Agrawal and S. Mishra, Effects of supplemental ultraviolet-B and cadmium on growth, antioxidants and yield of Pisum sativum L, Ecotoxicol. Environ. Saf., 2009, 72, 610–618 CrossRef CAS PubMed.
- L. B. Costa, D. E. N. Rangel, M. A. B. Morandi and W. Bettiol, Effects of UV-B radiation on the antagonistic ability of Clonostachys rosea to Botrytis cinerea on strawberry leaves, Biol. Control, 2013, 65, 95–100 CrossRef.
- J. A. H. Galvao and W. Bettiol, Effects of UV-B radiation on Lecanicillium spp., biological control agents of the coffee leaf rust pathogen, Trop. Plant Pathol., 2014, 39, 392–400 CrossRef.
- J. J. Wargent and B. R. Jordan, From ozone depletion to agriculture: understanding the role of UV radiation in sustainable crop production, New Phytol., 2013, 197, 1058–1076 CrossRef CAS PubMed.
- J. F. Bornman, P. W. Barnes, S. A. Robinson, C. L. Ballaré, S. D. Flint and M. M. Caldwell, Solar ultraviolet radiation and ozone depletion-driven climate change: effects on terrestrial ecosystems, Photochem. Photobiol. Sci., 2015, 14, 88–107 CAS.
- J. Song, R. Smart, H. Wang, B. Dambergs, A. Sparrow and M. C. Qian, Effect of grape bunch sunlight exposure and UV radiation on phenolics and volatile composition of Vitis vinifera L. cv. Pinot noir wine, Food Chem., 2015, 173, 424–431 CrossRef CAS PubMed.
- K. Šuklje, G. Antalick, Z. Coetzee, L. M. Schmidtke, H. B. Česnik, J. Brandt, W. J. du Toit, K. Lisjak and A. Deloire, Effect of leaf removal and ultraviolet radiation on the composition and sensory perception of Vitis viniferaL. cv. Sauvignon Blanc wine, Aust. J. Grape Wine Res., 2014, 20, 223–233 CrossRef.
- P. Carbonell-Bejerano, M. P. Diago, J. Martinez-Abaigar, J. M. Martinez-Zapater, J. Tardaguila and E. Nunez-Olivera, Solar ultraviolet radiation is necessary to enhance grapevine fruit ripening transcriptional and phenolic responses, BMC Plant Biol., 2014, 14 DOI:10.1186/1471-2229-1114-1183.
- J. Martinez-Luescher, N. Torres, G. Hilbert, T. Richard, M. Sanchez-Diaz, S. Delrot, J. Aguirreolea, I. Pascual and E. Gomes, Ultraviolet-B radiation modifies the quantitative and qualitative profile of flavonoids and amino acids in grape berries, Phytochemistry, 2014, 102, 106–114 CrossRef PubMed.
- C. N. Austin and W. F. Wilcox, Effects of sunlight exposure on grapevine powdery mildew development, Phytopathology, 2012, 102, 857–866 CrossRef PubMed.
- E. García-Cela, S. Marín, M. Reyes, V. Sanchis and A. J. Ramos, Conidia survival of Aspergillus section Nigri, Flavi and Circumdati under UV-A and UV-B radiation with cycling temperature/light regime, J. Sci. Food Agric., 2015 DOI:10.1002/jsfa.7343.
- L. Li, Y. Zhang, J. Luo, H. Korpelainen and C. Li, Sex-specific responses of Populus yunnanensis exposed to elevated CO2 and salinity, Physiol. Plant., 2013, 147, 477–488 CrossRef CAS PubMed.
- M. Juvany, M. Mueller, M. Pinto-Marijuan and S. Munne-Bosch, Sex-related differences in lipid peroxidation and photoprotection in Pistacia lentiscus, J. Exp. Bot., 2014, 65, 1039–1049 CrossRef CAS PubMed.
- S. Munne-Bosch, Sex ratios in dioecious plants in the framework of global change, Environ. Exp. Bot., 2015, 109, 99–102 CrossRef.
- T. R. Randriamanana, K. Nissinen, J. Moilanen, L. Nybakken and R. Julkunen-Tiitio, Long-term UV-B and temperature enhancements suggest that females of Salix myrsinifolia plants are more tolerant to UV-B than males, Environ. Exp. Bot., 2015, 109, 296–305 CrossRef CAS.
- T. R. Randriamanana, A. Lavola and R. Julkunen-Tiitto, Interactive effects of supplemental UV-B and temperature in European aspen seedlings: Implications for growth, leaf traits, phenolic defense and associated organisms, Plant Physiol. Biochem., 2015, 93, 84–93 CrossRef CAS PubMed.
- D. J. Erickson, B. Sulzberger, R. G. Zepp and A. T. Austin, Effects of stratospheric ozone depletion, solar UV radiation, and climate change on biogeochemical cycling: interactions and feedbacks, Photochem. Photobiol. Sci., 2015, 14, 127–148 CAS.
- A. T. Austin and C. L. Ballaré, Dual role of lignin in plant litter decomposition in terrestrial ecosystems, Proc.
Natl. Acad. Sci. U. S. A., 2010, 107, 4618–4622 CrossRef CAS PubMed.
- J. Y. King, L. A. Brandt and E. C. Adair, Shedding light on plant litter decomposition: advances, implications and new directions in understanding the role of photodegradation, Biogeochemistry, 2012, 111, 57–81 CrossRef.
- T. A. Day, R. Guénon and C. T. Ruhland, Photodegradation of plant litter in the Sonoran Desert varies by litter type and age, Soil Biol. Biochem., 2015, 89, 109–122 CrossRef CAS.
- Y. Lin, J. King, S. Karlen and J. Ralph, Using 2D NMR spectroscopy to assess effects of UV radiation on cell wall chemistry during litter decomposition, Biogeochemistry, 2015, 1–10 Search PubMed.
- N. R. Baker and S. D. Allison, Ultraviolet photodegradation facilitates microbial litter decomposition in a Mediterranean climate, Ecology, 2015, 96, 1994–2003 Search PubMed.
- Y. Lin, R. D. Scarlett and J. Y. King, Effects of UV photodegradation on subsequent microbial decomposition of Bromus diandrus litter, Plant Soil, 2015, 1–9 Search PubMed.
- A. Gaxiola and J. J. Armesto, Understanding litter decomposition in semiarid ecosystems: linking leaf traits, UV exposure and rainfall variability, Front. Plant Sci., 2015, 6, 140, DOI:110.3389/fpls.2015.00140.
- S. F. Yanni, E. C. Suddick and J. Six, Photodegradation effects on CO2 emissions from litter and SOM and photo-facilitation of microbial decomposition in a California grassland, Soil Biol. Biochem., 2015, 91, 40–49 CrossRef CAS.
- J. Wang, L. L. Liu, X. Wang and Y. W. Chen, The interaction between abiotic photodegradationand microbial decomposition under ultraviolet radiation, Global Change Biol., 2015, 21, 2095–2104 CrossRef PubMed.
- M. Almagro, F. T. Maestre, J. Martínez-López, E. Valencia and A. Rey, Climate change may reduce litter decomposition while enhancing the contribution of photodegradation in dry perennial Mediterranean grasslands, Soil Biol. Biochem., 2015, 90, 214–223 CrossRef CAS.
- L. Rizzini, J. J. Favory, C. Cloix, D. Faggionato, A. O'Hara, E. Kaiserli, R. Baumeister, E. Schäfer, F. Nagy, G. I. Jenkins and R. Ulm, Perception of UV-B by the Arabidopsis UVR8 protein, Science, 2011, 332, 103–106 CrossRef CAS PubMed.
- G. I. Jenkins, The UV-B photoreceptor UVR8: From structure to physiology, Plant Cell, 2014, 26, 21–37 CrossRef CAS PubMed.
- C. L. Ballaré, Light regulation of plant defense, Annu. Rev. Plant Biol., 2014, 65, 335–363 CrossRef PubMed.
- K. Zhang and B. Cu, Optogenetic control of intracellular signaling pathways, Trends Biotechnol., 2015, 33, 92–100 CrossRef CAS PubMed.
- L. J. Clarke and S. A. Robinson, Cell wall-bound ultraviolet-screening compounds explain the high ultraviolet tolerance of the Antarctic moss, Ceratodon purpureus, New Phytol., 2008, 179, 776–783 CrossRef PubMed.
- S. A. Robinson and M. J. Waterman, Sunsafe bryophytes: photoprotection from excess and damaging solar radiation, Adv. Photosyn. Resp., 2014, 37, 113–130 Search PubMed.
-
J. Bramley-Alves, D. H. King, S. A. Robinson and R. E. Miller, Dominating the Antarctic environment: bryophytes in a time of change, in Photosynthesis in Bryophytes and Early Land Plants, Springer, Netherlands, 2014, pp. 309–324 Search PubMed.
- L. Monforte, R. Tomas-Las-Heras, M.-A. Del-Castillo-Alonso, J. Martinez-Abaigar and E. Nunez-Olivera, Spatial variability of ultraviolet-absorbing compounds in an aquatic liverwort and their usefulness as biomarkers of current and past UV radiation: A case study in the Atlantic-Mediterranean transition, Sci. Total Environ., 2015, 518, 248–257 CrossRef PubMed.
- H. Hespanhol, G. Fabon, L. Monforte, J. Martinez-Abaigar and E. Nunez-Olivera, Among- and within-genus variability of the UV-absorption capacity in saxicolous mosses, Bryologist, 2014, 117, 1–9 CrossRef.
- W. T. Fraser, B. H. Lomax, P. E. Jardine, W. D. Gosling and M. A. Sephton, Pollen and spores as a passive monitor of ultraviolet
radiation, Front. Ecol. Evolution, 2014, 2 DOI:10.3389/fevo.2014.00012.
- J. Dinamarca, A. Sandoval-Alvarez, M. Gidekel and A. Gutierrez-Moraga, Differentially expressed genes induced by cold and UV-B in Deschampsia antarctica Desv, Polar Biol., 2013, 36, 409–418 CrossRef.
- P. W. Barnes, M. A. Tobler, K. Keefover-Ring, S. D. Flint, A. E. Barkley, R. J. Ryel and R. L. Lindroth, Rapid modulation of UV-shielding in plants is influenced by solar UV radiation and linked to alterations in flavonoids, Plant, Cell Environ., 2016, 39, 222–230 CrossRef CAS PubMed.
- P. W. Barnes, S. D. Flint, R. J. Ryel, M. A. Tobler, A. E. Barkley and J. J. Wargent, Rediscovering leaf optical properties: New insights into plant acclimation to solar UV radiation, Plant Physiol. Biochem., 2015, 93, 94–100 CrossRef CAS PubMed.
- R. Ulm and G. Jenkins, Q&A: How do plants sense and respond to UV-B radiation?, BMC Biol., 2015, 13, 45 CrossRef PubMed.
- S. Hayes, C. N. Velanis, G. I. Jenkins and K. A. Franklin, UV-B detected by the UVR8 photoreceptor antagonizes auxin signaling and plant shade avoidance, Proc. Natl. Acad. Sci. U. S. A., 2014, 111, 11894–11899 CrossRef CAS PubMed.
- O. Rechner and H.-M. Poehling, UV exposure induces resistance against herbivorous insects in broccoli, J. Plant Dis. Prot., 2014, 121, 125–132 Search PubMed.
- S. T. Đinh, I. Gális and I. T. Baldwin, UVB radiation and 17-hydroxygeranyllinalool diterpene glycosides provide durable resistance against mirid (Tupiocoris notatus) attack in field-grown Nicotiana attenuata plants, Plant, Cell Environ., 2013, 36, 590–606 CrossRef PubMed.
- D. Zhou, Y. M. Du, J. Yang, L. Zhang, H. Y. Zhao, Z. Q. Hu and X. S. Hu, Effects of UVB radiation in successive generations on the activities of protective enzymes in the grain aphid, Sitobion avenae (Hemiptera:Aphididae), Acta Entomol. Sin., 2014, 57, 762–768 CAS.
- Y. Du, W. Ping, Y. Jie, Z. Dong, L. GuangYu, Z. HuiYan, H. ZuQing and H. XiangShun, Effects of UV-B radiation intensity and duration on the growth, development and fecundity of Sitobion avenae (Hemiptera: Aphididae), Acta Entomol. Sin., 2014, 57, 1395–1401 Search PubMed.
- S. C. Burdick, D. A. Prischmann-Voldseth and J. P. Harmon, Density and distribution of soybean aphid, Aphis glycines Matsumura (Hemiptera: Aphididae) in response to UV radiation, Popul. Ecol., 2015, 1–10 Search PubMed.
- Y. Murata and M. Osakabe, Factors affecting photoreactivation in UVB-irradiated herbivorous spider mite (Tetranychus urticae), Exp. Appl. Acarol., 2014, 63, 253–265 CrossRef CAS PubMed.
- F. Tachi and M. Osakabe, Spectrum-specific UV egg damage and dispersal responses in the phytoseiid predatory mite Neoseiulus californicus (Acari: Phytoseiidae), Environ. Entomol., 2014, 43, 787–794 CrossRef PubMed.
- T. Suzuki, Y. Yoshioka, O. Tsarsitalidou, V. Ntalia, S. Ohno, K. Ohyama, Y. Kitashima, T. Gotoh, M. Takeda and D. S. Koveos, An LED-based UV-B irradiation system for tiny organisms: System description and demonstration experiment to determine the hatchability of eggs from four Tetranychus spider mite species from Okinawa, J. Insect Physiol., 2014, 62, 1–10 CrossRef CAS PubMed.
- C. L. Ballaré, M. M. Caldwell, S. D. Flint, S. A. Robinson and J. F. Bornman, Effects of solar ultraviolet radiation on terrestrial ecosystems. Patterns, mechanisms, and interactions with climate change, Photochem. Photobiol. Sci., 2011, 10, 226–241 Search PubMed.
- S. Debecker, R. Sommaruga, T. Maes and R. Stoks, Larval UV exposure impairs adult immune function through a trade-off with larval investment in cuticular melanin, Funct. Ecol., 2015, 29, 1292–1299 CrossRef.
- T. L. Delworth and F. Zeng, Regional rainfall decline in Australia attributed to anthropogenic greenhouse gases and ozone levels, Science, 2014, 7, 583–587 CAS.
- P. J. Young, S. M. Davis, B. Hassler, S. Solomon and K. H. Rosenlof, Modeling the climate impact of Southern Hemisphere ozone depletion: The importance of the ozone data set, Geophys. Res. Lett., 2015, 41.24, 9033–9039 Search PubMed.
- S. A. Robinson and D. J. Erickson III, Not just about sunburn–the ozone hole's profound effect on climate has significant implications for Southern Hemisphere ecosystems, Global Change Biol., 2015, 21, 515–527 CrossRef PubMed.
- N. Calvo, L. M. Polvani and S. Solomon, On the surface impact of Arctic stratospheric ozone extremes, Environ. Res. Lett., 2015, 10, 094003–094008 CrossRef.
- S. Zieger, A. V. Babanin and I. R. Young, Changes in ocean surface wind with a focus on trends in regional and monthly mean values, Deep Sea Res., Part I, 2014, 86, 56–67 CrossRef.
- P. Landschützer, N. Gruber, F. A. Haumann, C. Rödenbeck, D. C. E. Bakker, S. van Heuven, M. Hoppema, N. Metzl, C. Sweeney, T. Takahashi, B. Tilbrook and R. Wanninkhof, The reinvigoration of the Southern Ocean carbon sink, Science, 2015, 349, 1221–1224 CrossRef PubMed.
- V. H. L. Winton, A. R. Bowie, R. Edwards, M. Keywood, A. T. Townsend, P. van der Merwe and A. Bollhöfer, Fractional iron solubility of atmospheric iron inputs to the Southern Ocean, Mar. Chem., 2015, 1–13 Search PubMed.
- H. Weimerskirch, M. Louzao, S. de Grissac and K. Delord, Changes in wind pattern alter Albatross distribution and life-history traits, Science, 2012, 335, 211–214 CrossRef CAS PubMed.
- G. S. Blanco, J. P. Pisoni and F. Quintana, Characterization of the seascape used by juvenile and wintering adult Southern Giant Petrels from Patagonia Argentina, Estuaries Coasts Shelf Sci., 2015, 153, 135–144 CrossRef.
- R. Fay, H. Weimerskirch, K. Delord and C. Barbraud, Population density and climate shape early-life survival and recruitment in a long-lived pelagic seabird, J. Anim. Ecol., 2015, 84, 1423–1433 CrossRef PubMed.
- N. Dehnhard, K. Ludynia, M. Poisbleau, L. Demongin and P. Quillfeldt, Good days, bad days: Wind as a driver of foraging success in a flightless seabird, the southern rockhopper penguin, PLoS One, 2013, 8, e79487–e79489 Search PubMed.
- R. Villalba, A. Lara, M. H. Masiokas, R. Urrutia, B. H. Luckman, G. J. Marshall, I. A. Mundo, D. A. Christie, E. R. Cook, R. Neukom, K. Allen, P. Fenwick, J. A. Boninsegna, A. M. Srur, M. S. Morales, D. Araneo, J. G. Palmer, E. Cuq, J. C. Aravena, A. Holz and C. LeQuesne, Unusual Southern Hemisphere tree growth patterns induced by changes in the Southern Annular Mode, Nat. Geosci., 2012, 5, 793–798 CrossRef CAS.
- P. L. M. Gonzalez, L. M. Polvani, R. Seager and G. J. P. Correa, Stratospheric ozone depletion: a key driver of recent precipitation trends in South Eastern South America, Clim. Dyn., 2014, 42, 1775–1792 CrossRef.
- L. J. Clarke, S. A. Robinson, Q. Hua, D. J. Ayre and D. Fink, Radiocarbon bomb spike reveals biological effects of Antarctic climate change, Global Change Biol., 2012, 18, 301–310 CrossRef.
-
IPCC, Climate Change 2014: Impacts, Adaptation, and Vulnerability. Contribution of Working Group II to the Fifth Assessment Report of the Intergovernmental Panel on Climate Change. WGII AR5 Summary for Policymakers., Report No., IPCC, Geneva, Switzerland, 2014, p. 44.
-
IPCC, Summary for Policymakers: Climate Change 2014- Impacts, Adaptation, and Vulnerability, in Working Group II Contribution to the IPCC Fifth Assessment Report, 2014 Search PubMed.
- U.S Global Change Research Program, U.S. National Climate Assessment, 2014, U.S Global Change Research Program, http://nca2014.globalchange.gov/, accessed November, 2015.
- C. E. Williamson, J. A. Brentrup, J. Zhang, W. H. Renwick, B. R. Hargreaves, L. B. Knoll, E. Overholt and K. Rose, Lakes as sensors in the landscape: Optical metrics as scalable sentinel responses to climate change, Limnol. Oceanogr., 2014, 59, 840–850 CrossRef CAS.
- V. S. Kuwahara, S. Nozaki, J. Nakano, T. Toda, T. Kikuchi and S. Taguchi, 18-year variability of ultraviolet radiation penetration in the mid-latitude coastal waters of the western boundary Pacific, Estuar. Coast. Shelf Sci., 2015, 160, 1–9 CrossRef CAS.
- R. W. Sanders, S. L. Cooke, J. M. Fischer, S. B. Fey, A. W. Heinze, W. H. Jeffrey, A. L. Macaluso, R. E. Moeller, D. P. Morris, P. J. Neale, M. H. Olson, J. D. Pakulski, J. A. Porter, D. M. Schoener and C. E. Williamson, Shifts in microbial food web structure and productivity after additions of naturally occurring dissolved organic matter: Results from large-scale lacustrine mesocosms, Limnol. Oceanogr., 2015, 60, 2130–2144 CrossRef CAS.
- S. Hylander, M. T. Ekvall, G. Bianco, X. Yang and L.-A. Hansson, Induced tolerance expressed as relaxed behavioural threat response in millimetre-sized aquatic organisms, Proc. R. Soc. London, Ser. B, 2014, 281 DOI:10.1098/rspb.2014.0364.
- M. T. Ekvall, S. Hylander, T. Walles, X. Yang and L.-A. Hansson, Diel vertical migration, size distribution and photoprotection in zooplankton as response to UV-A radiation, Limnol. Oceanogr., 2015, 60, 2048–2058 Search PubMed.
- J. M. Fischer, M. H. Olson, N. Theodore, C. E. Williamson, K. C. Rose and J. Hwang, Diel vertical migration of copepods in mountain lakes: the changing role of ultraviolet radiation across a transparency gradient, Limnol. Oceanogr., 2015, 60, 252–262 CrossRef.
- S. L. Cooke, J. M. Fischer, K. Kessler, C. E. Williamson, R. W. Sanders, D. P. Morris, J. A. Porter, W. H. Jeffrey, S. DeVaul Princiotta and J. D. Pakulski, Direct and indirect effects of additions of chromophoric dissolved organic matter on zooplankton during large-scale mesocosm experiments in an oligotrophic lake, Freshwater Biol., 2015, 60, 2362–2378 CrossRef CAS.
- T. H. Leach, C. E. Williamson, N. Theodore, J. M. Fischer and M. H. Olson, The role of ultraviolet radiation in the diel vertical migration of zooplankton: an experimental test of the transparency-regulator hypothesis, J. Plankton Res., 2015, 37, 886–896 CrossRef.
- R. M. Cory, C. P. Ward, B. C. Crump and G. W. Kling, Sunlight controls water column processing of carbon in arctic fresh waters, Science, 2014, 345, 925–928 CrossRef CAS PubMed.
- R. M. Cory, K. H. Harrold, B. T. Neilson and G. W. Kling, Controls on dissolved organic matter (DOM) degradation in a headwater stream: the influence of photochemical and hydrological conditions in determining light-limitation or substrate-limitation of photo-degradation, Biogeosci. Discuss., 2015, 12, 9793–9838 Search PubMed.
- D.-P. Häder, C. E. Williamson, S.-Å. Wängberg, M. Rautio, K. C. Rose, K. Gao, E. W. Helbling, R. P. Sinha and R. Worrest, Effects of UV radiation on aquatic ecosystems and interactions with other environmental factors, Photochem. Photobiol. Sci., 2015, 14, 108–126 Search PubMed.
- I. Fountoulakis, A. F. Bais, K. Tourpali, K. Fragkos and S. Misios, Projected changes in solar UV radiation in the Arctic and sub-Arctic Oceans: Effects from changes in reflectivity, ice transmittance, clouds, and ozone, J. Geophys. Res.: Atmos, 2014, 119, 8073–8090 Search PubMed.
- S. Hylander, T. Kiørboe, P. Snoeijs, R. Sommaruga and T. G. Nielsen, Concentrations of sunscreens and antioxidant pigments in Arctic Calanus spp. in relation to ice cover, ultraviolet radiation, and the phytoplankton spring bloom, Limnol. Oceanogr., 2015, 60, 2197–2206 CrossRef CAS.
- M. Sigmond and J. C. Fyfe, The Antarctic sea ice response to the ozone hole in climate models, J. Climate, 2014, 27, 1336–1342 CrossRef.
- F. S. Paolo, H. A. Fricker and L. Padman, Volume loss from Antarctic ice shelves is accelerating, Science, 2015, 348, 327–331 CrossRef CAS PubMed.
- M. Fischetti, Deep heat threatens marine life, Sci. Am., 2013, 308, 92 CrossRef PubMed.
- Y. Xie, G. H. Tilstone, C. Widdicombe, E. M. S. Woodward, C. Harris and M. K. Barnes, Effect of increases in temperature and nutrients on phytoplankton community structure and photosynthesis in the western English Channel, Mar. Ecol.: Prog. Ser., 2015, 519, 61–73 CrossRef CAS.
- E. W. Helbling, A. T. Banaszak and V. E. Villafañe, Global change feed-back inhibits cyanobacterial photosynthesis, Sci. Rep., 2015, 5, 14514 CrossRef PubMed.
- J. B. Palter, The role of the Gulf Stream in European climate, Annu. Rev. Mater. Sci., 2015, 7, 113–137 Search PubMed.
- S. Rahmstorf, J. E. Box, G. Feulner, M. E. Mann, A. Robinson, S. Rutherford and E. J. Schaffernicht, Exceptional twentieth-century slowdown in Atlantic Ocean overturning circulation, Nat. Clim. Change, 2015, 5, 475–480 CrossRef.
- J. B. Butcher, D. Nover, T. E. Johnson and C. M. Clark, Sensitivity of lake thermal and mixing dynamics to climate change, Clim. Change, 2015, 129, 295–305 CrossRef CAS.
- B. M. Kraemer, O. Anneville, S. Chandra, M. Dix, E. Kuusisto, D. M. Livingstone, A. Rimmer, G. Schladow, E. A. Silow, L. M. Sitoki, R. Tamatamah, Y. Vadeboncoeur and P. B. McIntyre, Morphometry and average temperature affect lake stratification responses to climate change, Geophys. Res. Lett., 2015, 42, 4981–4988 CrossRef.
- J. J. Heiskanen, I. Mammarella, A. Ojala, V. Stepanenko, K.-M. Erkkilä, H. Miettinen, H. Sandström, W. Eugster, M. Leppäranta, H. Järvinen, T. Vesala and A. Nordbo, Effects of water clarity on lake stratification and lake-atmosphere heat exchange, J. Geophys. Res., 2015, 120, 7412–7428 Search PubMed.
- K. M. G. Mostofa, C. Q. Liu, W. D. Zhai, M. Minella, D. Vione, K. Gao, D. Minakata, T. Arakaki, T. Yoshioka, K. Hayakawa, E. Konohira, E. Tanoue, A. Akhand, A. Chanda, B. Wang and H. Sakugawa, Reviews and Syntheses: Ocean acidification and its potential impacts on marine ecosystems, Biogeosci. Discuss., 2015, 12, 10939–10983 Search PubMed.
- K. Xu and K. Gao, Solar UV irradiances modulate effects of ocean acidification on the coccolithophorid Emiliania huxleyi, Photochem. Photobiol., 2015, 91, 92–101 CrossRef CAS PubMed.
- W. Li, K. Gao and J. Beardall, Nitrate limitation and ocean acidification interact with UV-B to reduce photosynthetic performance in the diatom Phaeodactylum tricornutum, Biogeosciences, 2015, 12, 2383–2393 CAS.
- H. Chen, W. Guan, G. Zeng, P. Li and S. Chen, Alleviation of solar ultraviolet radiation (UVR)-induced photoinhibition in diatom Chaetoceros curvisetus by ocean acidification, J. Mar. Biol. Assoc. U. K., 2015, 95, 661–667 CrossRef CAS.
- F. C. Curriero, J. A. Patz, J. B. Rose and S. Lele, The association between extreme precipitation and waterborne disease outbreaks in the United States, 1948–1994., Am. J. Public Health, 2001, 91, 1194–1199 CrossRef CAS PubMed.
- A. Ouali, H. Jupsin, J. L. Vasel and A. Ghrabi, Removal of E. coli and enterococci in maturation pond and kinetic modelling under sunlight conditions, Desalin. Water Treat., 2015, 53, 1068–1074 CAS.
- G. U. L. Braga, D. E. N. Rangel, E. K. K. Fernandes, S. D. Flint and D. W. Roberts, Molecular and physiological effects of environmental UV radiation on fungal conidia, Curr. Genet., 2015, 61, 405–425 CrossRef CAS PubMed.
- A. Beckinghausen, A. Martinez, D. Blersch and B. Z. Haznedaroglu, Association of nuisance filamentous algae Cladophora spp. with E. coli and Salmonella in public beach waters: impacts of UV protection on bacterial survival, Environ. Sci.: Processes Impacts, 2014, 16, 1267–1274 CAS.
- A. B. Hoyer, S. G. Schladow and F. J. Rueda, A hydrodynamics-based approach to evaluating the risk of waterborne pathogens entering drinking water intakes in a large, stratified lake, Water Res., 2015, 83, 227–236 CrossRef CAS PubMed.
- R. L. Cramp, S. Reid, F. Seebacher and C. E. Franklin, Synergistic interaction between UVB radiation and temperature increases susceptibility to parasitic infection in a fish, Biol. Lett., 2014, 10 DOI:10.1098/rsbl.2014.0449.
- M. Korkaric, R. Behra, B. B. Fischer, M. Junghans and R. I. L. Eggen, Multiple stressor effects in Chlamydomonas reinhardtii – Toward understanding mechanisms of interaction between effects of ultraviolet radiation and chemical pollutants, Aquat. Toxicol., 2015, 162, 18–28 CrossRef CAS PubMed.
- N. Regier, C. Cosio, N. von Moos and V. I. Slaveykova, Effects of copper-oxide nanoparticles, dissolved copper and ultraviolet radiation on copper bioaccumulation, photosynthesis and oxidative stress in the aquatic macrophyte Elodea nuttallii, Chemosphere, 2015, 128, 56–61 CrossRef CAS PubMed.
- S. Yu, S. Tang, G. D. Mayer, G. P. Cobb and J. D. Maul, Interactive effects of ultraviolet-B radiation and pesticide exposure on DNA photo-adduct accumulation and expression of DNA damage and repair genes in Xenopus laevis embryos, Aquat. Toxicol., 2015, 159, 256–266 CrossRef CAS PubMed.
- S. Yu, S. M. Weir, G. P. Cobb and J. D. Maul, The effects of pesticide exposure on ultraviolet-B radiation avoidance behavior in tadpoles, Sci. Total Environ., 2014, 481, 75–80 CrossRef CAS PubMed.
- F. J. R. C. Coelho, D. F. R. Cleary, R. J. M. Rocha, R. Calado, J. M. Castanheira, S. M. Rocha, A. M. S. Silva, M. M. Q. Simões, V. Oliveira, A. I. Lillebø, A. Almeida, Â. Cunha, I. Lopes, R. Ribeiro, M. Moreira-Santos, C. R. Marques, R. Costa, R. Pereira and N. C. M. Gomes, Unraveling the interactive effects of climate change and oil contamination on laboratory-simulated estuarine benthic communities, Global Change Biol., 2015, 21, 1871–1886 CrossRef PubMed.
- S. R. Batchu, V. R. Panditi, K. E. O'Shea and P. R. Gardinali, Photodegradation of antibiotics under simulated solar radiation: Implications for their environmental fate, Sci. Total Environ., 2014, 470, 299–310 CrossRef PubMed.
- A. E. Poste, H. F. V. Braaten, H. A. de Wit, K. Sørensen and T. Larssen, Effects of photodemethylation on the methylmercury budget of boreal Norwegian lakes, Environ. Toxicol. Chem., 2015, 34, 1213–1223 CrossRef CAS PubMed.
- C. H. Rose, S. Ghosh, J. D. Blum and B. A. Bergquist, Effects of ultraviolet radiation on mercury isotope fractionation during photo-reduction for inorganic and organic mercury species, Chem. Geol., 2015, 405, 102–111 CrossRef CAS.
- C. A. Downs, E. Kramarsky-Winter, R. Segal, J. Fauth, S. Knutson, O. Bronstein, F. R. Ciner, R. Jeger, Y. Lichtenfeld, C. M. Woodley, P. Pennington, K. Cadenas, A. Kushmaro and Y. Loya, Toxicopathological effects of the sunscreen UV filter, Oxybenzone (Benzophenone-3), on coral planulae and cultured primary cells and its environmental contamination in Hawaii and the U.S. Virgin Islands, Arch. Environ. Contam. Toxicol., 2015 DOI:10.1007/s00244-00015-00227-00247.
- D. Sánchez-Quiles and A. Tovar-Sánchez, Sunscreens as a source of hydrogen peroxide production in coastal waters, Environ. Sci. Technol., 2014, 48, 9037–9042 CrossRef PubMed.
- A. Tovar-Sanchez, D. Sanchez-Quiles, G. Basterretxea, J. L. Benede, A. Chisvert, A. Salvador, I. Moreno-Garrido and J. Blasco, Sunscreen products as emerging pollutants to coastal waters, PLoS One, 2013, 8, e65451 CAS.
- J. Beardall, S. Stojkovic and K. Gao, Interactive effects of nutrient supply and other environmental factors on the sensitivity of marine primary producers to ultraviolet radiation: implications for the impacts of global change, Aquat. Biol., 2014, 22, 5–23 CrossRef.
- S. R. Halac, V. E. Villafane, R. J. Goncalves and E. W. Helbling, Photochemical responses of three marine phytoplankton species exposed to ultraviolet radiation and increased temperature: Role of photoprotective mechanisms, J. Photochem. Photobiol., B, 2014, 141, 217–227 CrossRef CAS PubMed.
- K. Svanfeldt, L. Lundqvist, C. Rabinowitz, H. N. Skold and B. Rinkevich, Repair of UV-induced DNA damage in shallow water colonial marine species, J. Exp. Mar. Biol. Ecol., 2014, 452, 40–46 CrossRef CAS.
- S. Y. Ha, H. M. Joo, S. H. Kang, I. Y. Ahn and K. H. Shin, Effect of ultraviolet irradiation on the production and composition of fatty acids in plankton in a sub-Antarctic environment, J. Oceanogr., 2014, 70, 1–10 CrossRef CAS.
- C. Hogg, M. Neveu, L. Folkow, K.-A. Stokkan, J. H. Kam, R. H. Douglas and G. Jeffery, The eyes of the deep diving hooded seal (Cystophora cristata) enhance sensitivity to ultraviolet light, Biol. Open, 2015, 4, 812–818 CrossRef PubMed.
- M. Gagliano, M. Depczynski and U. E. Siebeck, Facing the environment: onset and development of UV markings in young fish, Sci. Rep., 2015, 5, 13193 CrossRef CAS PubMed.
- E. Ghanizadeh Kazerouni, C. E. Franklin, F. Seebacher and J. Grindstaff, UV-B radiation interacts with temperature to determine animal performance, Funct.Ecol., 2015 DOI:10.1111/1365-2435.12520.
- R. Hossaini, M. P. Chipperfield, S. A. Montzka, A. Rap, S. Dhomse and W. Feng, Efficiency of short-lived halogens at influencing climate through depletion of stratospheric ozone, Nat. Geosci., 2015, 8, 186–190 CrossRef CAS.
- B.-M. Sinnhuber and S. Meul, Simulating the impact of emissions of brominated very short lived substances on past stratospheric ozone trends, Geophys. Res. Lett., 2015, 42, 2449–2456 CrossRef CAS.
- L. J. Carpenter and P. D. Nightingale, Chemistry and release of gases from the surface ocean, Chem. Rev., 2015, 115, 4015–4034 CrossRef CAS PubMed.
- J. D. Méndez-Díaz, K. K. Shimabuku, J. Ma, Z. O. Enumah, J. J. Pignatello, W. A. Mitch and M. C. Dodd, Sunlight-driven photochemical halogenation of dissolved organic matter in seawater: a natural abiotic source of organobromine and organoiodine, Environ. Sci. Technol., 2014, 48, 7418–7427 CrossRef PubMed.
- C. Y. Lin and S. L. Manley, Bromoform production from seawater treated with bromoperoxidase, Limnol. Oceanogr., 2012, 57, 1857–1866 CrossRef CAS.
- M. Rex, I. Wohltmann, T. Ridder, R. Lehmann, K. Rosenlof, P. Wennberg, D. Weisenstein, J. Notholt, K. Krüger, V. Mohr and S. Tegtmeier, A tropical West Pacific OH minimum and implications for stratospheric composition, Atmos. Chem. Phys., 2014, 14, 4827–4841 CrossRef.
- X. Feng, J. E. Vonk, B. E. van Dongen, O. Gustafsson, I. P. Semiletov, O. V. Dudarev, Z. Wang, D. B. Montlucon, L. Wacker and T. I. Eglinton, Differential mobilization of terrestrial carbon pools in Eurasian Arctic river basins, Proc. Natl. Acad. Sci. U. S. A., 2013, 110, 14168–14173 CrossRef CAS PubMed.
- E. A. G. Schuur, A. D. McGuire, C. Schaedel, G. Grosse, J. W. Harden, D. J. Hayes, G. Hugelius, C. D. Koven, P. Kuhry, D. M. Lawrence, S. M. Natali, D. Olefeldt, V. E. Romanovsky, K. Schaefer, M. R. Turetsky, C. C. Treat and J. E. Vonk, Climate change and the permafrost carbon feedback, Nature, 2015, 520, 171–179 CrossRef CAS PubMed.
- J. Vonk, S. Tank, W. Bowden, I. Laurion, W. Vincent, P. Alekseychik, M. Amyot, M. Billet, J. Canário and R. Cory, Reviews and Syntheses: Effects of permafrost thaw on arctic aquatic ecosystems, Biogeosci. Discuss., 2015, 12, 7129–7167 CrossRef.
- C. M. Sharpless, M. Aeschbacher, S. E. Page, J. Wenk, M. Sander and K. McNeill, Photooxidation-induced changes in optical, electrochemical, and photochemical properties of humic substances, Environ. Sci. Technol., 2014, 48, 2688–2696 CrossRef CAS PubMed.
- C. M. Sharpless and N. V. Blough, The importance of charge-transfer interactions in determining chromophoric dissolved organic matter (CDOM) optical and photochemical properties, Environ. Sci.: Processes Impacts, 2014, 16, 654–671 CAS.
- T. Davies-Barnard, P. J. Valdes, J. S. Singarayer, A. J. Wiltshire and C. D. Jones, Quantifying the relative importance of land cover change from climate and land use in the representative concentration pathways, Global Biogeochem. Cycles, 2015, 29, 842–853 CrossRef CAS.
- P. I. Araujo and A. T. Austin, A shady business: Pine afforestation alters the primary controls on litter decomposition along a precipitation gradient in Patagonia, Argentina, J. Ecol., 2015, 103, 1408–1420 CrossRef CAS.
- A. T. Austin, Has water limited our imagination for aridland biogeochemistry?, Trends in Ecology and Evolution, 2011, 26, 229–235 CrossRef PubMed.
-
P. W. Barnes, H. L. Throop, S. R. Archer, D. D. Breshears, R. L. McCulley and M. A. Tobler, Sunlight and soil–litter mixing: drivers oflitter decomposition in drylands, in Progress in Botany, Springer, 2015, pp. 273–302 Search PubMed.
- D. Frank, M. Reichstein, M. Bahn, K. Thonicke, D. Frank, M. D. Mahecha, P. Smith, M. Velde, S. Vicca and F. Babst, Effects of climate extremes on the terrestrial carbon cycle: concepts, processes and potential future impacts, Global Change Biol., 2015, 8, 2861–2880 CrossRef PubMed.
- A. Rap, D. V. Spracklen, L. Mercado, C. L. Reddington, J. M. Haywood, R. J. Ellis, O. L. Phillips, P. Artaxo, D. Bonal, N. Restrepo Coupe and N. Butt, Fires increase Amazon forest productivity through increases in diffuse radiation, Geophys. Res. Lett., 2015, 42, 4654–4662 CrossRef.
- S. Wagner and R. Jaffé, Effect of photodegradation on molecular size distribution and quality of dissolved black carbon, Org. Geochem., 2015, 86, 1–4 CrossRef CAS.
- G. Allen, C. J. Halsall, J. Ukpebor, N. D. Paul, G. Ridall and J. J. Wargent, Increased occurrence of pesticide residues on crops grown in protected environments compared to crops grown in open field conditions, Chemosphere, 2015, 119, 1428–1435 CrossRef CAS PubMed.
- J. Weber, C. J. Halsall, J. J. Wargent and N. D. Paul, The aqueous photodegradation of fenitrothion under various agricultural plastics: Implications for pesticide longevity in agricultural ‘micro-environments’, Chemosphere, 2009, 76, 147–150 CrossRef CAS PubMed.
- S. Bahnmuller, U. von Gunten and S. Canonica, Sunlight-induced transformation of sulfadiazine and sulfamethoxazole in surface waters and wastewater effluents, Water Res., 2014, 57, 183–192 CrossRef CAS PubMed.
- M. J. Mattle, D. Vione and T. Kohn, Conceptual model and experimental framework to determine the contributions of direct and indirect photoreactions to the solar disinfection of MS2, phiX174, and adenovirus, Environ. Sci. Technol., 2015, 49, 334–342 CrossRef CAS PubMed.
- M. Ratti, S. Canonica, K. McNeill, J. Bolotin and T. B. Hofstetter, Isotope fractionation associated with the photochemical dechlorination of chloroanilines, Environ. Sci. Technol., 2015, 49, 9797–9806 CrossRef CAS PubMed.
- A. I. Silverman, M. T. Nguyen, I. E. Schilling, J. Wenk and K. L. Nelson, Sunlight inactivation of viruses in open-water unit process treatment wetlands: modeling endogenous and exogenous inactivation rates, Environ. Sci. Technol., 2015, 49, 2757–2766 CrossRef CAS PubMed.
- C. Kingston, R. Zepp, A. Andrady, D. Boverhof, R. Fehir, D. Hawkins, J. Roberts, P. Sayre, B. Shelton, Y. Sultan, V. Vejins and W. Wohlleben, Release characteristics of selected carbon nanotube polymer composites, Carbon, 2014, 68, 33–57 CrossRef CAS.
- W. C. Hou, S. BeigzadehMilani, C. T. Jafvert and R. G. Zepp, Photoreactivity of unfunctionalized single-wall carbon nanotubes involving hydroxyl radical: chiral dependency and surface coating effect, Environ. Sci. Technol., 2014, 48, 3875–3882 CrossRef CAS PubMed.
- W. C. Hou, I. Chowdhury, D. G. Goodwin, W. M. Henderson, D. H. Fairbrother, D. Bouchard and R. G. Zepp, Photochemical transformation of graphene oxide in sunlight, Environ. Sci. Technol., 2015, 49, 3435–3443 CrossRef CAS PubMed.
- W. T. Fraser, E. Blei, S. C. Fry, M. F. Newman, D. S. Reay, K. A. Smith and A. R. McLeod, Emission of methane, carbon monoxide, carbon dioxide and short-chain hydrocarbons from vegetation foliage under ultraviolet irradiation, Plant, Cell Environ., 2015, 38, 980–989 CrossRef CAS PubMed.
- A. A. Bloom, J. Lee-Taylor, S. Madronich, D. J. Messenger, P. I. Palmer, D. S. Reay and A. R. McLeod, Global methane emission estimates from ultraviolet irradiation of terrestrial plant foliage, New Phytol., 2010, 187, 417–425 CrossRef CAS PubMed.
- P. Hess, D. Kinnison and Q. Tang, Ensemble simulations of the role of the stratosphere in the attribution of northern extratropical tropospheric ozone variability, Atmos. Chem. Phys., 2015, 15, 2341–2365 CrossRef CAS.
- H. Zhang, S. Wu, Y. Huang and Y. Wang, Effects of stratospheric ozone recovery on photochemistry and ozone air quality in the troposphere, Atmos. Chem. Phys., 2014, 14, 4079–4086 CrossRef.
- A. Banerjee, A. T. Archibald, A. C. Maycock, P. Telford, N. L. Abraham, X. Yang, P. Braesicke and J. A. Pyle, Lightning NOx, a key chemistry–climate interaction: impacts of future climate change and consequences for tropospheric oxidising capacity, Atmos. Chem. Phys., 2014, 14, 9871–9881 CrossRef.
- M. Meinshausen, S. J. Smith, K. Calvin, J. S. Daniel, M. L. T. Kainuma, J. F. Lamarque, K. Matsumoto, S. A. Montzka, S. C. B. Raper, K. Riahi, A. Thomson, G. J. M. Velders and D. P. P. van Vuuren, The RCP greenhouse gas concentrations and their extensions from 1765 to 2300, Clim. Change, 2011, 109, 213–241 CrossRef CAS.
- P. J. Young, A. T. Archibald, K. W. Bowman, J. F. Lamarque, V. Naik, D. S. Stevenson, S. Tilmes, A. Voulgarakis, O. Wild, D. Bergmann, P. Cameron-Smith, I. Cionni, W. J. Collins, S. B. Dalsøren, R. M. Doherty, V. Eyring, G. Faluvegi, L. W. Horowitz, B. Josse, Y. H. Lee, I. A. MacKenzie, T. Nagashima, D. A. Plummer, M. Righi, S. T. Rumbold, R. B. Skeie, D. T. Shindell, S. A. Strode, K. Sudo, S. Szopa and G. Zeng, Pre-industrial to end 21st century projections of tropospheric ozone from the Atmospheric Chemistry and Climate Model Intercomparison Project (ACCMIP), Atmos. Chem. Phys., 2013, 13, 2063–2090 CAS.
- O. R. Cooper, D. D. Parrish, J. Ziemke, N. V. Balashov, M. Cupeiro, I. E. Galbally, S. Gilge, L. Horowitz, N. R. Jensen, J. F. Lamarque, V. Naik, S. J. Oltmans, J. Schwab, D. T. Shindell, A. M. Thompson, V. Thouret, Y. Wang and R. M. Zbinden, Global distribution and trends of tropospheric ozone: An observation-based review, Elementa, 2014, 2, 000029–000028 Search PubMed.
- W. W. Verstraeten, J. L. Neu, J. E. Williams, K. W. Bowman, J. R. Worden and K. F. Boersma, Rapid increases in tropospheric ozone production and export from China, Nat. Geosci., 2015, 8, 690–695 CrossRef CAS.
- C. S. Malley, M. R. Heal, G. Mills and C. F. Braban, Trends and drivers of ozone human health and vegetation impact metrics from UK EMEP supersite measurements (1990–2013), Atmos. Chem. Phys., 2015, 15, 4025–4042 CAS.
-
WHO, Review of evidence on health aspects of air pollution – REVIHAAP Project, World Health Organization, Regional Office for Europe Report No., Geneva, Switzerland, Aug 16 2013, pp. 1–309.
- P. K. Patra, M. C. Krol, S. A. Montzka, T. Arnold, E. L. Atlas, B. R. Lintner, B. B. Stephens, B. Xiang, J. W. Elkins, P. J. Fraser, A. Ghosh, E. J. Hintsa, D. F. Hurst, K. Ishijima, P. B. Krummel, B. R. Miller, K. Miyazaki, F. L. Moore, J. Mühle, S. O'Doherty, R. G. Prinn, L. P. Steele, M. Takigawa, H. J. Wang, R. F. Weiss, S. C. Wofsy and D. Young, Observational evidence for interhemispheric hydroxyl-radical parity, Nature, 2014, 513, 219–223 CrossRef CAS PubMed.
- P. Achakulwisut, L. J. Mickley, L. T. Murray, A. P. K. Tai, J. O. Kaplan and B. Alexander, Uncertainties in isoprene photochemistry and emissions: implications for the oxidative capacity of past and present atmospheres and for climate forcing agents, Atmos. Chem. Phys., 2015, 15, 7977–7998 CrossRef CAS.
- H. Fuchs, I. H. Acir, B. Bohn, T. Brauers, H. P. Dorn, R. Häseler, A. Hofzumahaus, F. Holland, M. Kaminski, X. Li, K. Lu, A. Lutz, S. Nehr, F. Rohrer, R. Tillmann, R. Wegener and A. Wahner, OH regeneration from methacrolein oxidation investigated in the atmosphere simulation chamber SAPHIR, Atmos. Chem. Phys., 2014, 14, 7895–7908 CrossRef CAS.
- T. B. Nguyen, J. D. Crounse, R. H. Schwantes, A. P. Teng, K. H. Bates, X. Zhang, J. M. St Clair, W. H. Brune, G. S. Tyndall, F. N. Keutsch, J. H. Seinfeld and P. O. Wennberg, Overview of the Focused Isoprene eXperiment at the California Institute of Technology (FIXCIT): mechanistic chamber studies on the oxidation of biogenic compounds, Atmos. Chem. Phys., 2014, 14, 13531–13549 CrossRef.
- J. Peeters, J.-F. Müller, T. Stavrakou and V. S. Nguyen, Hydroxyl Radical Recycling in Isoprene Oxidation Driven by Hydrogen Bonding and Hydrogen Tunneling: The Upgraded LIM1 Mechanism, J. Phys. Chem. A, 2014, 118, 8625–8643 CrossRef CAS PubMed.
- F. Rohrer, K. Lu, A. Hofzumahaus, B. Bohn, T. Brauers, C.-C. Chang, H. Fuchs, R. Häseler, F. Holland, M. Hu, K. Kita, Y. Kondo, X. Li, S. Lou, A. Oebel, M. Shao, L. Zeng, T. Zhu, Y. Zhang and A. Wahner, Maximum efficiency in the hydroxyl-radical-based self-cleansing of the troposphere, Nat. Geosci., 2014, 7, 559–563 CrossRef CAS.
- J. M. Anglada, M. Martins-Costa, M. F. Ruiz-Lopez and J. S. Francisco, Spectroscopic signatures of ozone at the air-water interface and photochemistry implications, Proc. Natl. Acad. Sci. U. S. A., 2014, 111, 11618–11623 CrossRef CAS PubMed.
- D. E. Shallcross, K. E. Leather, A. Bacak, P. Xiao, E. P. F. Lee, M. Ng, D. K. W. Mok, J. M. Dyke, R. Hossaini, M. P. Chipperfield, M. A. H. Khan and C. J. Percival, Reaction between CH3O2 and BrO Radicals: A New Source of Upper Troposphere Lower Stratosphere Hydroxyl Radicals, J. Phys. Chem. A, 2015, 119, 4618–4632 CrossRef CAS PubMed.
- R. F. Hansen, S. M. Griffith, S. Dusanter, P. S. Rickly, P. S. Stevens, S. B. Bertman, M. A. Carroll, M. H. Erickson, J. H. Flynn, N. Grossberg, B. T. Jobson, B. L. Lefer and H. W. Wallace, Measurements of total hydroxyl radical reactivity during CABINEX 2009 - Part 1: field measurements, Atmos. Chem. Phys., 2014, 14, 2923–2937 Search PubMed.
- K. Hens, A. Novelli, M. Martinez, J. Auld, R. Axinte, B. Bohn, H. Fischer, P. Keronen, D. Kubistin, A. C. Nölscher, R. Oswald, P. Paasonen, T. Petäjä, E. Regelin, R. Sander, V. Sinha, M. Sipilä, D. Taraborrelli, C. Tatum Ernest, J. Williams, J. Lelieveld and H. Harder, Observation and modelling of HOx radicals in a boreal forest, Atmos. Chem. Phys., 2014, 14, 8723–8747 Search PubMed.
- R. T. Lidster, J. F. Hamilton, J. D. Lee, A. C. Lewis, J. R. Hopkins, S. Punjabi, A. R. Rickard and J. C. Young, The impact of monoaromatic hydrocarbons on OH reactivity in the coastal UK boundary layer and free troposphere, Atmos. Chem. Phys., 2014, 14, 6677–6693 Search PubMed.
- A. C. Nölscher, T. Butler, J. Auld, P. Veres, A. Muñoz, D. Taraborrelli, L. Vereecken, J. Lelieveld and J. Williams, Using total OH reactivity to assess isoprene photooxidation via measurement and model, Atmos. Environ., 2014, 89, 453–463 CrossRef.
- J. Williams and W. Brune, A roadmap for OH reactivity research, Atmos. Environ., 2015, 106, 371–372 CrossRef CAS.
- W. R. Simpson, S. S. Brown, A. Saiz-Lopez, J. A. Thornton and R. v. Glasow, Tropospheric Halogen Chemistry: Sources, Cycling, and Impacts, Chem. Rev., 2015, 115, 4035–4062 CrossRef CAS PubMed.
-
N. Bândă, M. Krol, T. Noije, M. Weele, J. E. Williams, P. Le Sager, U. Niemeier, L. Thomason and T. Rockmann, The effect of stratospheric sulfur from Mount Pinatubo on tropospheric oxidizing capacity and methane, 2015, pp. 1–19 Search PubMed.
- A. Hodzic, S. Madronich, P. S. Kasibhatla, G. Tyndall, B. Aumont, J. L. Jimenez, J. Lee-Taylor and J. Orlando, Organic photolysis reactions in tropospheric aerosols: effect on secondary organic aerosol formation and lifetime, Atmos. Chem. Phys., 2015, 15, 9253–9269 CAS.
- J. Lelieveld, J. S. Evans, M. Fnais, D. Giannadaki and A. Pozzer, The contribution of outdoor air pollution sources to premature mortality on a global scale, Nature, 2015, 525, 367–371 CrossRef CAS PubMed.
-
U.S. EPA, Health Risk and Exposure Assessment for Ozone - Final Report, Report No.EPA-452/R-14-004a, 8/2014, 2014, p. 502.
-
WHO, Health Risks of Air Pollution in Europe – HRAPIE Project, Recommendations for Concentration–Response Functions for Cost–Benefit Analysis of Particulate Matter, Ozone and Nitrogen Dioxide, World Health Organization, Regional Office for Europe Report No., Geneva, Switzerland, Dec 13 2013, p. 60.
- J. E. Goodman, R. L. Prueitt, S. N. Sax, H. N. Lynch, K. Zu, J. C. Lemay, J. M. King and F. J. Venditti, Weight-of-evidence evaluation of short-term ozone exposure and cardiovascular effects, Crit. Rev. Toxicol., 2014, 44, 725–790 CrossRef CAS PubMed.
- C. Petito Boyce, J. E. Goodman, S. N. Sax and C. T. Loftus, Providing perspective for interpreting cardiovascular mortality risks associated with ozone exposures, Regul. Toxicol. Pharmacol., 2015, 72, 107–116 CrossRef CAS PubMed.
- R. L. Prueitt, H. N. Lynch, K. Zu, S. N. Sax, F. J. Venditti and J. E. Goodman, Weight-of-evidence evaluation of long-term ozone exposure and cardiovascular effects, Crit. Rev. Toxicol., 2014, 44, 791–822 CrossRef CAS PubMed.
- S. H. Moolgavkar, R. O. McClellan, A. Dewanji, J. Turim, E. G. Luebeck and M. Edwards, Time-series analyses of air pollution and mortality in the United States: a subsampling approach, Environ. Health Perspect., 2013, 121, 73–78 CrossRef PubMed.
-
K. R. Solomon, G. J. M. Velder, S. R. Wilson, S. Madronich, J. Longstreth, P. J. Aucamp and J. F. Bornman, Sources, Fates, Toxicity, and Risks of Trifluoroacetic Acid and its Salts: Relevance to Substances Regulated under the Montreal Protocol, A Report Prepared by the UNEP Environmental Effects Assessment Panel, UNEP Ozone Secretariat Report No. 2016-01, Nairobi, Kenya, January 2016, p. 25.
- J. Tocháček and Z. Vrátníčková, Polymer life-time prediction: The role of temperature in UV accelerated ageing of polypropylene and its copolymers, Polym. Test., 2014, 36, 82–87 CrossRef.
- G. Pastorelli, C. Cucci, O. Garcia, G. Piantanida, A. Ellnaggar, M. Casser and M. Strlic, Environmentary induced colour change during natural degradation of selected polymers, Polym. Degrad. Stab., 2014, 107, 198–209 CrossRef CAS.
- S. Butylinal and T. Kark, Resistance to weathering of wood-polypropylene and wood-wollastonite-polypropylene composites made with and without carbon black, Pigm. Resin Technol., 2014, 43, 185–193 CrossRef.
- D. J. Growney, P. W. Fowler, O. O. Mykhaylyk, L. A. Fielding, M. J. Derry, N. Aragrag, G. D. Lamb and S. P. Armes, Determination of effective particle density for sterically stabilized carbon black particles: Effect of diblock copolymer stabilizer composition, Langmuir, 2015, 31, 8764–8773 CrossRef CAS PubMed.
- Y. Javadi, M. S. Hosseini and M. K. R. Aghjeh, The effect of carbon black and HALS hybrid systems on the UV stability of high-density polyethylene (HDPE), Iran. Polym. J., 2014, 23, 793–799 CrossRef CAS.
- S. Yao, A. Tominaga, H. Sekiguchi and E. Takatori, UV degradation properties of virgin/recycled polymer blend, Nihon Reoroji Gakkaishi, 2014, 42, 61–64 CrossRef CAS.
- R. El-Hage, A. Vinetto, R. Sonnier, L. Fery and J. Lopez–Cuesta, Flame retardancy of ethylene vinyl-acetate EVA using alminum based fillers, Polym. Degrad. Stab., 2014, 108, 56–67 CrossRef CAS.
- J. F. G. Larche, L. Boudiaf-Lomri, C. Fouland, I. Duemmler and M. Meyer, Evidence of surface accumulation of fillers during thr photo-oxidatin of flame retrdant ATH filled EVA used for cable applications, Polym. Degrad. Stab., 2014, 103, 63–68 CrossRef CAS.
- D. C. Bento, E. C. R. Maia, R. V. Fernandes, E. Laureto, G. Louarn and H. de Santana, Photoluminescence and Raman spectroscopy studies of the photodegradation of poly(3-octylthiophene), J. Mater. Sci.: Mater. Electron., 2014, 25, 185–189 CrossRef CAS.
- I. F. Dominguez, P. D. Topham, P. O. Bussiere, D. Begue and A. Rivaton, Photodegradation mechanisms of a low bandgap polymer by combining experimental and modeling approaches, J. Phys. Chem. C, 2014, 63, 1335–1345 Search PubMed.
- N. Sai, K. Leung, J. Zádord and J. Henkelmana, First principles study of photo-oxidation degradation mechanisms in P3HT for organic solar cells, Phys. Chem. Chem. Phys., 2014, 16, 8092–8099 RSC.
-
A. Badiee, R. Wildman and I. Ashcroft, Effect of UV aging on degradation of Ethylene-vinyl Acetate (EVA) as encapsulant in photovoltaic (PV) modules, in SPIE, 9179, Reliability of Photovoltaic Cells, Modules, Components, and Systems VI, 2014, p. 7, DOI:10.1117/12.2062007.
- F. Liu, L. Jiang and S. Yang, Ultra-violet degradation behavior of polymeric backsheets for photovoltaic modules, Solar Energy, 2014, 108, 88–100 CrossRef CAS.
- A. Rivaton, A. Tournebize, J. Gaume, P. O. Bussiere, J. L. Gardette and S. Therias, Photostability of organaic materials used in polymer solar cells, Polym. Int., 2014, 63, 1335–1345 CrossRef CAS.
- A. Tournebize, A. Rivaton, H. Peisert and T. Chasse, The crucial role of confined residual additives on the photostability of P3HT:PCBM active layers, J. Phys. Chem., 2015, 119, 9142–9148 CAS.
- M. Zborowska, A. Stachowiak-Wencek, M. Nowaczyk-Organista, B. Waliszewska and W. Pradzynski, Analysis of photodegradation process of Pinus sylvestris L. wood after treatment with acid and alkaline buffers and light irradiation, BioResources, 2015, 10, 2057–2066 CrossRef CAS.
- G. Bonifazia, C. Luca, G. Capobiancoa, A. L. Monacob, C. Pelosic, R. Picchiob and S. Serrantia, Effect of profiling and preservative treatments on the weathering characteristics of Southern pine deck boards, Polym. Degrad. Stab., 2015, 113, 10–21 CrossRef.
- L. Calienno, C. Pelosi, R. Picchio, G. Agresti, U. Santamaria, F. Balletti and A. L. Monaco, Light-induced color changes and chemical modification of treated and untreated chestnut wood surface, Stud. Conserv., 2015, 60, 131–139 CrossRef CAS.
- Y. Liu, L. Shao, J. Gao, Y. Chen, Q. Cheng and B. K. Via, Surface photo-discoloration and degradation of dyed wood veneer exposed to different wavelengths of artificial light, Appl. Surf. Sci., 2015, 331, 353–361 CrossRef CAS.
- C. Wan, Y. Jiao and J. Li, In situ deposition of graphene nanosheets on wood surface by one-pot hydrothermal method for enhanced UV-resistant ability, Appl. Surf. Sci., 2015, 347, 891–897 CrossRef CAS.
- W. Gan, L. Gao, Q. Sun, C. Jin, Y. Lu and L. Li, Multifunctional wood materials with magnetic, superhydrophobic and anti-ultraviolet properties, Appl. Surf. Sci., 2014, 332, 565–572 CrossRef.
- X. Wang, S. Liu, H. Chang and J. Liu, Sol-gel deposition of TIO2 nanocoatings on wood surfaces with enhanced hydrophobicity and photostability, Wood Fiber Sci., 2014, 46, 109–117 CrossRef CAS.
- Y. Lu, S. Xiao, R. Gao, J. Li and Q. Sun, Improved weathering performance and wettability of wood protected by CeO2 coating deposited onto the surface, Holzforschung, 2014, 68, 345–351 CrossRef CAS.
- V. Vardanyan, T. Galstian and B. Riedl, Effect of additon of cellulose nanocrystals to wood coating s on color changes and surfacee roughenss due to accelerated weathering, Col. Res. Appl., 2015, 39, 519–529 Search PubMed.
- D. Rosu, R. Bodirlau, C. A. Teaca, L. Rosu and C. D. Varganici, Epoxy and succinic anhydride functionalized soybean oil for wood protection against UV light action, J. Cleaner Prod., 2015, 112, 1175–1183 CrossRef.
-
D. Cirule, A. Meija-Feldmane and E. Kuka, Colour stability of thermally modified hardwood, in 20th International Scientific Conference on Research for Rural Development, Vol. 2, Latvia University of Agriculture, Jelgava, Latvia, 2014, pp. 103–108 Search PubMed.
- L. Tolvaj, R. Nemeth, Z. Pasztory, L. Bej and P. Takats, Colour stability of thermally modified wood during short-term photodegradation, BioResources, 2014, 9, 6644–6651 CrossRef CAS.
- D. Todaro, M. D'Auria, F. Langerame, A. M. Salvi and A. Scopa, Surface characterization of untreated and hydro-thermally pre-treated turkey oak woods after UV-C irradiation., Surf. Interface Anal., 2015, 47, 206–215 CrossRef.
- E. D. Tomak, D. Ustaomer and S. Yildiz, Changes in surface and mechanical properties of heat treated wood during natural weathering, Measurement, 2014, 53, 30–39 CrossRef.
- Y.-S. Jang, T. Amna, M. S. Hassan, H.-C. Kim, J.-H. Kim, S.-H. Baik and M.-S. Khil, Nanotitania/mulberry fibers as novel textile with anti-yellowing and intrinsic antimicrobial properties, Ceram. Interfaces, 2015, 41, 6274–6280 CrossRef CAS.
- M. Rehan, H. M. Mashaly, A. Salwa Mowafi, Abou El-Kheir and E. E. Emam, Multi-functional textile design using in situ Ag NPs incorporation into natural fabric matrix, Dyes Pigm., 2015, 118, 9–17 CrossRef CAS.
- B. Tang, L. Suna, J. Li, J. Kaur, H. Zhu, S. Qin, Y. Yao, W. Chen and W. X. Xungai, Functionalization of bamboo pulp fabrics with noble metal nanoparticle, Dyes Pigm., 2015, 113, 289–298 CrossRef CAS.
- P. S. Sobolewskia, J. W. Krzyścina, J. Jarosławskia, J. Winka, A. Lesiakb and J. Narbuttb, Controlling adverse and beneficial effects of solar UV radiation by wearing suitable clothes – Spectral transmission of different kinds of fabrics, J. Photochem. Photobiol., B, 2014, 140, 105–110 CrossRef PubMed.
- L. Qu, M. Tian, X. Hu, Y. Wang, S. Zhu, X. Guo, G. Han, X. Zhang, K. Sun and X. Tang, Functionalization of cotton fabric at low graphene nanoplate content for ultra-strong ultraviolet blocking, Carbon, 2014, 80, 565–574 CrossRef CAS.
- M. Tian, X. Tang, L. Qu, S. Zhu, X. Guo and G. Han, Robust ultraviolet blocking cotton fabric modified with chitosan/graphene nanocomposites, Mater. Lett., 2015, 145, 340–343 CrossRef CAS.
Footnote |
† List of contributing authors in alphabetical order: Anthony Andrady, Pieter J. Aucamp, Amy T. Austin, Alkiviadis F. Bais, Carlos L. Ballaré, Paul W. Barnes, Germar H. Bernhard, Lars Olof Björn, *Janet F. Bornman (Co-Chair), David J. Erickson (passed away, 16th November 2015), Frank R. de Gruijl, Donat-P. Häder, Mohammad Ilyas, Janice Longstreth, Robyn M. Lucas, Sasha Madronich, Richard L. McKenzie, Rachel Neale, Mary Norval, Krishna K. Pandey, Nigel Paul (Co-Chair), Halim Hamid Redhwi, Sharon A. Robinson, Kevin Rose, Min Shao (Co-Chair), Rajeshwar P. Sinha, Keith R. Solomon (Secretary), Barbara Sulzberger, Yukio Takizawa, Ayako Torikai, Kleareti Tourpali, Craig E. Williamson, Stephen R. Wilson, Sten-Åke Wängberg, Robert C. Worrest, Antony R. Young, and Richard G. Zepp. *Corresponding author: E-mail: janet.bornman@curtin.edu.au |
|
This journal is © The Royal Society of Chemistry and Owner Societies 2016 |