DOI:
10.1039/C6OB01731B
(Paper)
Org. Biomol. Chem., 2016,
14, 10593-10598
Synthesis of HIV-1 capsid protein assembly inhibitor (CAP-1) and its analogues based on a biomass approach†
Received
10th August 2016
, Accepted 16th September 2016
First published on 30th September 2016
Abstract
A biomass-derived platform chemical was utilized to access a demanded pharmaceutical substance with anti-HIV activity (HIV, human immunodeficiency virus) and a variety of structural analogues. Step economy in the synthesis of the drug core (single stage from cellulose) is studied including flexible variability of four structural units. The first synthesis and X-ray structure of the inhibitor of HIV-1 capsid protein assembly (CAP-1) is described.
Introduction
State-of-the-art healthcare urges for the on-demand production of pharmaceuticals1 and access to personalized medicine.2 The long term practical implementation of these goals is challenging to achieve due to unsustainable procedures of drugs synthesis, which are typically characterized by large overall production of toxic waste.3 In fact, the synthesis of drugs is accompanied by the largest amount of waste by-products among all chemical industry processes (25–100 kg of waste per 1 kg of drug product).3 A breakthrough concept in this area is to connect improved synthesis and step-economy4 with sustainable natural sources of chemicals.5 The use of biomass-derived chemicals has opened up new possibilities in the sustainable synthesis of bulk and fine chemicals.6 In this article, we describe a complete synthetic procedure to promising anti-HIV pharmaceutical substances starting from a low cost natural biomass (cellulose) and using a special optimized procedure for efficient fine organic synthesis applications.7
The search for effective anti-HIV drugs is one of the most important challenges in modern science and medicine.8 AIDS causes more than a million deaths annually.9 There is no cure or vaccine against AIDS, but antiretroviral therapy can slow the course of the disease and increase the survival time after infection.10,11 In this field, the rapid synthesis of candidate compounds with the possibility of synthesizing close structural analogues is of crucial importance.12
Currently available drugs against HIV are focused on the inhibition of the five stages of the HIV life cycle (Fig. 1) and include CCR5 coreceptor antagonists (maraviroc), which prevent HIV from binding to CD4 cells; fusion inhibitors (enfuvirtide), which block the HIV envelope from merging with the host CD4 cell membrane; nucleoside (lamivudine, zidovudine, emtricitabine, abacavir, azidothymidine, didanosine, etc.) and non-nucleoside (rilpivirine, etravirine, delavirdine, efavirenz, etc.) reverse transcriptase inhibitors, which block the conversion of HIV RNA into DNA; integrase strand transfer inhibitors (raltegravir, dolutegravir), which block the insertion of HIV DNA into CD4 cell DNA; and viral protease inhibitors (tipranavir, indinavir, lopinavir, ritonavir, darunavir, etc.), which prevent the cleavage of newly synthesized viral polyproteins at appropriate places for creating mature protein components of the infectious HIV virion.13
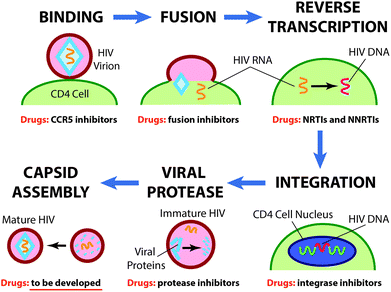 |
| Fig. 1 Therapeutic target stages of HIV life cycle and the corresponding drugs. | |
Recently, a novel type of anti-HIV activity – inhibition of HIV-1 capsid protein assembly, which is the last stage of HIV maturation – was described.14N-(3-Chloro-4-methylphenyl)-N′-2-[(5-[(dimethylamino)-methyl]-2-furylmethyl)sulfanyl]ethyl-urea (CAP-1) was one of the first compounds with such activity.14g,h The structure of CAP-1 was disclosed in patents,15 however, to the best of our knowledge, the methods of synthesis have not been described and a 3D molecular structure was not determined.
Results and discussion
Here we report an efficient synthesis of the CAP-1 molecule which allows versatile structural variation (Fig. 2). The structure of CAP-1 can be subdivided into five fragments, which should be introduced into the target molecule as individual subunits. Retrosynthetic analysis of CAP-1 shows that this approach can be easily realized on the basis of the 2,5-disubstituted furan core (shown in red, Fig. 2), which is available from natural biomass involving the 5-HMF (5-(hydroxymethyl)furfural) platform-chemical7,16 pathway (Fig. 2). The first site, which can be varied easily, is an amine group (drug unit 1, Fig. 2). Variation at this position is possible via reductive amination of the 5-HMF aldehyde group with various primary and secondary amines. The second site is a spacer of variable length and flexibility (drug unit 2, Fig. 2). The third site (drug unit 3, Fig. 2) is a linkage group, which connects the spacer to an aromatic, aliphatic or heteroaromatic substituent (drug unit 4, Fig. 2) and can also be involved in hydrogen bonding with the substrate. The linkage can be composed of such important groups as urea, amide, ester, carbonate, carbamate, sulphonamide, etc. Each of these four sites can be varied independently, and the final molecule can be assembled using efficient synthetic procedures. Thus, a library of CAP-1 structural analogues can be synthesized on the basis of this biomass-derived approach.
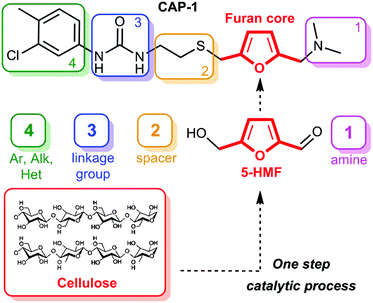 |
| Fig. 2 Retrosynthetic analysis of CAP-1 and its partitioning into sites of structural variation: red – furan core, magenta – amine, orange – spacer, blue – linkage group, green – aromatic, heteroaromatic or aliphatic substituent. | |
A practical synthesis procedure is shown in Scheme 1. The overall synthesis includes the following six steps: (1) CrCl3-catalysed conversion of cellulose into 5-HMF in an ionic liquid;7,17 (2) preparation of 5-(chloromethyl)furfural6b,c,18 (5-CMF) upon treatment of 5-HMF with HCl; (3) S-alkylation of N-acetylcysteamine with 5-CMF; (4) reductive amination of the formyl group by using dimethylamine; (5) alkaline hydrolysis of the amide; (6) synthesis of the urea group by using arylisocyanate. Each step was conducted smoothly, and compound purification via chromatography was required only once, at the final step. The target product was isolated in 57% overall yield in a crystal form with >99.9% purity (see the ESI† for analysis). The critical point of the synthetic procedure was to ensure the use of purified 5-HMF (free from dimer and oligomer impurities) to avoid formation of by-products, simplify separation and to increase the yield.7 The solid-state structure of the synthesized CAP-1 molecule was determined by single crystal X-ray diffraction for the first time and revealed a twisted linear geometry (Fig. 3). The determined molecular structure (see the ESI† for complete details) gives key information for performing docking experiments, understanding the mechanism of action and the analysis of biological activity.
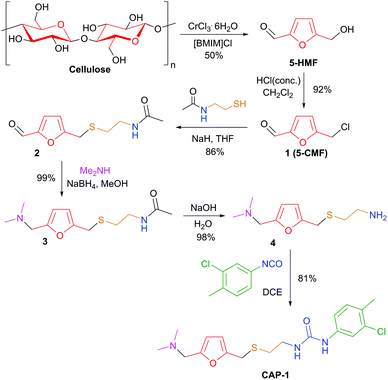 |
| Scheme 1 Synthesis of CAP-1 from cellulose as the starting material (similar color encoding of fragments was used, see Fig. 2). | |
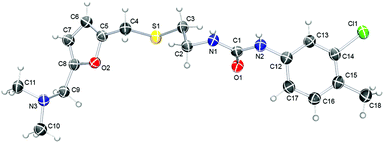 |
| Fig. 3 Molecular view of CAP-1 determined by X-ray analysis. Selected geometry parameters: N(3)–C(9) = 1.475 Å, C(9)–C(8) = 1.497 Å, ∠N(3)C(9)C(8) = 113.26°, C(5)–C(4) = 1.487 Å, C(4)–S(1) = 1.843 Å, ∠C(5)C(4)S(1) = 107.61°, ∠C(4)S(1)C(3) = 102.25°, ∠S(1)C(3)C(2)N(1) = 168.33°, ∠C(1)N(2)C(12)C(17) = 53.48°, and ∠N(1)C(1)N(2) = 115.17° (see the ESI† for complete description). | |
To demonstrate the applicability of the developed approach several structural analogues of CAP-1 were synthesized (Table 1). Variation in the substituents and in the nature of the linkage were successfully carried out giving the products in 46–79% yield (from 5-HMF). The developed biomass-derived approach was fully applicable for rapid access to various CAP-1 functionalized derivatives.
Table 1 Synthetic scope in the preparation of CAP-1 analogues
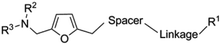
|
Entry |
R1 |
R2 |
R3 |
Linkage |
Spacer |
Yield, % |
1 |
4-Methyl-3-chlorophenyl |
Me |
Me |
Urea |
|
46 |
2 |
Ph |
Me |
Me |
Urea |
–SCH2CH2– |
70 |
3 |
Me |
Me |
Me |
Amide |
–SCH2CH2– |
79 |
4 |
1-Adamantan-methyl |
Me |
Me |
Urea |
–SCH2CH2– |
72 |
5 |
1-Chloro-3-adamantyl |
Me |
Me |
Urea |
–SCH2CH2– |
66 |
7 |
p-Tolyl |
Me |
Me |
Sulfonamide |
–SCH2CH2– |
75 |
8 |
2-Nitrophenyl |
Me |
Me |
Sulfonamide |
–SCH2CH2– |
65 |
9 |
H |
Cyclo-propyl |
H |
Alcohol |
–S–CH2CH2– |
55 |
10 |
t-Bu |
Me |
Me |
Carbamate |
–SCH2CH2– |
73 |
Conclusions
In conclusion, we have evaluated a convenient synthetic procedure for the preparation of the prominent HIV-1 capsid protein assembly inhibitor CAP-1 using cellulose as a renewable starting material. The structure of CAP-1 was determined by X-ray analysis. Using the developed procedure, several structural analogues of CAP-1 were successfully prepared and made available for high demand biochemical applications. Direct connection of the drug core to renewable natural sources of chemicals provides an efficient and green approach for the production of these pharmaceutical substances. Implementation of such methodologies into fine organic synthesis procedures is crucial to achieve long term sustainable drug development.
Conversion of natural carbohydrates into the 5-HMF platform chemical is a sustainable process,7a,16 and nowadays the production of 5-HMF approaches an industrial multi-ton scale.19 Therefore, a variety of efficient and practical implementations of biomass-derived drugs synthesis can be anticipated.
Experimental
5-(Hydroxymethyl)furfural was prepared from cellulose according to our recently published procedure.7a
5-(Chloromethyl)furfural (1)20
A 25 mL Erlenmeyer flask was loaded with 5-HMF (252 mg, 2 mmol), CH2Cl2 (10 mL), and conc. HCl (5 mL). The mixture was stirred at room temperature overnight. The aqueous layer was extracted with CH2Cl2 (3 × 20 mL). The combined organic phase was dried over sodium sulfate. Charcoal (50 mg) was added and the mixture was stirred for 20 minutes and filtered through a Celite pad. The solvent was removed under reduced pressure to give 5-(chloromethyl)furfural (266 mg, 92%) as a yellow liquid. The product should be stored below +5 °C to prevent decomposition. 1H NMR (CDCl3, 400 MHz) δ = 9.61 (s, 1H), 7.18 (d, 1H, J = 3.5 Hz), 6.57 (d, 1H, J = 3.5 Hz), 4.59 (s, 2H). 13C{1H} NMR (CDCl3, 101 MHz) δ = 177.1, 156.0, 152.9, 121.6, 111.9, 36.5; Anal. Calcd for C6H5ClO2: C, 49.85; H, 3.49; Cl, 24.52; Found: C, 49.84; H, 3.50, Cl, 24.49. HRMS (ESI): calcd [M + Na]+ 166.9870, Found: 166.9872.
5-[[(2-Acetamidoethyl)thio]methyl]furfural (2)6b
A Schlenk flask was loaded with N-acetylcysteamine (203 mg, 1.70 mmol) and a magnetic stirrer bar. The flask was filled with argon via three vacuum–argon cycles. 10 mL of absolute THF (distilled over sodium-benzophenone ketyl) was added. Sodium hydride (95%, 52 mg, 2.17 mmol) was added and the reaction mixture was stirred for 30 minutes. After that the solution of 5-(chloromethyl)furfural (256 mg, 1.77 mmol) in 5 mL of absolute THF was added dropwise. The reaction mixture was stirred overnight at room temperature. The solvent was removed under reduced pressure and brine (55 mL) was added. At this moment the color changed from light yellow to red-orange. The mixture was extracted with CH2Cl2 (4 × 40 mL), and the combined organic phase was washed with brine (100 mL) and dried over anhydrous Na2SO4. Charcoal (70 mg) was added and the mixture was stirred for another 20 minutes and filtered through a 15 mm Celite® pad. The solvent was removed under reduced pressure to give 5-[[(2-acetamidoethyl)thio]methyl]furfural (332 mg, 86%) as a yellow oil. 1H NMR (CDCl3, 400 MHz) δ = 9.57 (s, 1H), 7.19 (d, 1H, J = 3.5 Hz), 6.46 (d, 1H, J = 3.5 Hz), 6.17 (brs, 1H), 3.79 (s, 2H), 3.45 (q, 2H, J = 6.2 Hz), 2.73 (t, 2H, J = 6.5 Hz), 2.03 (s, 3H). 13C{1H} NMR (CDCl3, 101 MHz) δ = 177.2, 170.3, 158.8, 152.4, 122.7, 110.5, 38.3, 32.1, 28.0, 23.2. Anal. Calcd for C10H13NO3S: C, 52.85; H, 5.77; N, 6.16; Found: C, 52.81; H, 5.80, N, 6.14. HRMS (ESI): calcd [M + Na]+ 250.0508, Found: 250.0515.
5-[[(2-Acetamidoethyl)thio]methyl]-N,N-dimethyl-2-furanmethanamine (3)6b
The liquid dimethylamine (1 mL, prepared from dimethylamine hydrochloride and solid NaOH) was added to a solution of 5-[[(2-acetamidoethyl)thio]methyl]furfural (410 mg, 1.804 mmol) in 60 mL of absolute methanol and the resulting mixture was stirred at room temperature for 40 minutes. The solution became an intense orange. The reaction mixture was cooled to 0 °C and sodium borohydride (103 mg, 2.706 mmol) was added portionwise. The mixture was stirred at 0 °C for 20 minutes and then warmed up to room temperature. The solvent was removed under reduced pressure and the residue was dissolved in CH2Cl2, filtered from inorganic impurities, and evaporated to dryness to give 5-[[(2-acetamidoethyl)thio]methyl]-N,N-dimethyl-2-furanmethanamine (459 mg, 99%) as a yellow oil. 1H NMR (CDCl3, 400 MHz) δ = 6.27 (brs, 1H), 6.11 (s, 2H), 3.69 (s, 2H), 3.41 (s, 2H), 3.30 (q, 2H, J = 6.2 Hz), 2.65 (t, 2H, J = 6.5 Hz), 2.24 (s, 6H), 1.95 (s, 3H). 13C{1H} NMR (CDCl3, 101 MHz) δ = 170.2, 151.9, 151.4, 109.8, 108.2, 56.0, 45.1, 38.5, 31.8, 28.3, 23.3. Anal. Calcd for C12H20N2O2S: C, 56.61; H, 6.33; N, 11.00; Found: C, 56.21; H, 7.91, N, 10.90. HRMS (ESI): calcd [M + H]+ 257.1318, Found: 257.1321.
5-[[(2-Aminoethyl)thio]methyl]-N,N-dimethyl-2-furanmethanamine (4)
5-[[(2-Acetamidoethyl)-thio]methyl]-N,N-dimethyl-2-furanmethanamine (44 mg, 0.172 mmol) was added to 2 mL of 2N NaOH. The mixture was heated at reflux for 2.5 hours, then cooled down to room temperature and extracted with CH2Cl2 (3 × 5 mL). The combined organic phase was washed with brine (15 mL) and dried over anhydrous Na2SO4. The solvent was removed under reduced pressure, and the residue was dried in vacuo to give 5-[[(2-aminoethyl)thio]methyl]-N,N-dimethyl-2-furanmethanamine (36 mg, 98%) as a pale yellow oil. 1H NMR (CDCl3, 400 MHz) δ = 6.09 (s, 2H), 3.67 (s, 2H), 3.40 (s, 2H), 2.81 (t, 2H, J = 6.4 Hz), 2.59 (t, 2H, J = 6.4 Hz), 2.23 (s, 6H), 1.90 (brs, 2H). 13C{1H} NMR (CDCl3, 101 MHz) δ = 151.9, 151.3, 109.4, 108.0, 55.9, 45.0, 40.8, 35.7, 28.1. Anal. Calcd for C12H18N2OS: C, 56.04; H, 8.47; N, 13.07; Found: C, 56.01; H, 8.48, N, 13.02. HRMS (ESI): calcd [M + H]+ 239.1213, Found: 239.1217.
1-(3-Chloro-4-methylphenyl)-3-(2-(((5-((dimethylamino)methyl)furan-2-yl)methyl)thio)ethyl)urea (CAP-1)
To a solution of 5-[[(2-aminoethyl)thio]methyl]-N,N-dimethyl-2-furanmethanamine (110 mg, 0.513 mmol) in 5 ml of 1,2-dichloroethane, 3-chloro-4-methylphenylisocyanate (90.3 mg, 0.539 mmol) was added and the mixture was heated at 50 °C overnight. The solvent was removed under reduced pressure and the residue was purified by column chromatography (eluent CHCl3
:
MeOH = 8
:
1 (v/v)). The product was dried in vacuo to obtain CAP-1 (159 mg, 81%) as a white solid. 1H NMR (CDCl3, 500 MHz) δ = 7.51 (s, 1H), 7.38 (d, 1H, J = 1.9 Hz), 7.13 (dd, 1H, J = 8.2, 1.9 Hz), 7.07 (d, 1H, J = 8.2 Hz), 6.15 (d, 1H, J = 3.0 Hz), 6.11 (d, 1H, J = 3.0 Hz), 5.82 (t, 1H, J = 5.8 Hz), 3.70 (s, 2H), 3.49 (s, 2H), 3.84 (q, 2H, J = 6.0 Hz), 2.69 (t, 2H, J = 6.0 Hz), 2.31 (s, 6H), 2.28 (s, 3H). 13C{1H} NMR (CDCl3, 126 MHz) δ = 155.9, 152.2, 150.6, 138.2, 134.5, 131.1, 130.3, 120.6, 118.4, 110.7, 108.3, 55.9, 45.0, 39.4, 33.0, 28.7, 19.4. Anal. Calcd for C18H24ClN3O2S: C, 56.61; H, 6.33; N, 11.00; Found: C, 56.33; H, 6.36, N, 10.72. HRMS (ESI): calcd [M + H]+ 382.1351, Found: 382.1350. Crystals suitable for X-ray analysis were grown using a vapor diffusion method in a chloroform/hexane system .
Synthesis of CAP-1 derivatives
The detailed description of the synthesis and characterization of structural analogues of CAP-1 is provided in the ESI.† Adamatane-containing isocyanates for the synthesis of adamantyl derivatives were obtained according to the literature.21
Acknowledgements
This work was supported by the Russian Science Foundation (RSF Grant 14-13-01030). The authors thank Dr Victor Khrustalev for carrying out X-ray analysis and Dr Viktor Burmistrov and Prof. Gennady Butov for precursors of adamantyl derivatives.
Notes and references
-
(a) A. Adamo, R. L. Beingessner, M. Behnam, J. Chen, T. F. Jamison, K. F. Jensen, J.-C. M. Monbaliu, A. S. Myerson, E. M. Revalor, D. R. Snead, T. Stelzer, N. Weeranoppanant, S. Y. Wong and P. Zhang, Science, 2016, 352, 61–67 CrossRef CAS PubMed;
(b) R. E. Martin, Science, 2016, 352, 44–45 CrossRef CAS PubMed.
-
(a) M. A. Hamburg and F. S. Collins, N. Engl. J. Med., 2010, 363, 301–304 CrossRef CAS PubMed;
(b) A. D. Weston and L. Hood, J. Proteome Res., 2004, 3, 179–196 CrossRef CAS PubMed;
(c) P. C. Ng, S. S. Murray, S. Levy and J. C. Venter, Nature, 2009, 461, 724–726 CrossRef CAS PubMed;
(d) L. Chin, J. N. Andersen and P. A. Futreal, Nat. Med., 2011, 17, 297–303 CrossRef CAS PubMed;
(e) R. L. Schilsky, Nat. Rev. Drug Discovery, 2010, 9, 363–366 CrossRef CAS PubMed;
(f) G. S. Ginsburg and J. J. McCarthy, Trends Biotechnol., 2001, 19, 491–496 CrossRef CAS PubMed.
-
(a) R. A. Sheldon, Green Chem., 2007, 9, 1273–1283 RSC;
(b) R. A. Sheldon, Chem. Commun., 2008, 3352–3365 RSC.
-
(a) P. A. Wender, V. A. Verma, T. J. Paxton and T. H. Pillow, Acc. Chem. Res., 2008, 41, 40–49 CrossRef CAS PubMed;
(b) P. A. Wender and B. L. Miller, Nature, 2009, 460, 197–201 CrossRef CAS PubMed;
(c) P. A. Wender, Nat. Prod. Rep., 2014, 31, 433–440 RSC.
-
(a) C. H. Christensen, J. Rass-Hansen, C. C. Marsden, E. Taarning and K. Egeblad, ChemSusChem, 2008, 1, 283–289 CrossRef CAS PubMed;
(b) P. Anastas and N. Eghbali, Chem. Soc. Rev., 2010, 39, 301–312 RSC;
(c) P. J. Dunn, Chem. Soc. Rev., 2012, 41, 1452–1461 RSC;
(d) J. A. Geboers, S. Van de Vyver, R. Ooms, B. Op de Beeck, P. A. Jacobs and B. F. Sels, Catal. Sci. Technol., 2011, 1, 714–726 RSC;
(e) S. Van de Vyver, J. Geboers, P. A. Jacobs and B. F. Sels, ChemCatChem, 2011, 3, 82–94 CrossRef CAS.
-
(a) M. E. Zakrzewska, E. Bogel-Łukasik and R. Bogel-Łukasik, Chem. Rev., 2011, 111, 397–417 CrossRef CAS PubMed;
(b) M. Mascal and S. Dutta, Green Chem., 2011, 13, 3101–3102 RSC;
(c) M. Mascal and E. B. Nikitin, Green Chem., 2010, 12, 370–373 RSC;
(d) F. Chang, W.-H. Hsu and M. Mascal, Sustainable Chem. Pharm., 2015, 1, 14–18 CrossRef;
(e) F. Chang, S. Dutta, J. J. Becnel, A. S. Estep and M. Mascal, J. Agric. Food Chem., 2014, 62, 476–480 CrossRef CAS PubMed.
-
(a) K. I. Galkin, E. A. Krivodaeva, L. V. Romashov, S. S. Zalesskiy, V. V. Kachala, J. V. Burykina and V. P. Ananikov, Angew. Chem., Int. Ed., 2016, 55, 8338–8342 CrossRef CAS PubMed;
(b) A. S. Kashin, K. I. Galkin, E. A. Khokhlova and V. P. Ananikov, Angew. Chem., Int. Ed., 2016, 55, 2161–2166 CrossRef CAS PubMed.
-
(a) L. O. Kallings, J. Intern. Med., 2008, 263, 218–243 CrossRef CAS PubMed;
(b) G. H. Friedland and R. S. Klein, N. Engl. J. Med., 1987, 317, 1125–1135 CrossRef CAS PubMed;
(c) C. Flexner, Nat. Rev. Drug Discovery, 2007, 6, 959–966 CrossRef CAS PubMed;
(d) E. D. Clercq, Nat. Rev. Drug Discovery, 2007, 6, 1001–1018 CrossRef PubMed;
(e) P. Piot, M. Bartos, P. D. Ghys, N. Walker and B. Schwartlander, Nature, 2001, 410, 968–973 CrossRef CAS PubMed;
(f) D. D. Richman, Nature, 2001, 410, 995–1001 CrossRef CAS PubMed;
(g) V. Hughes, Nature, 2010, 466, S11–S13 CrossRef CAS PubMed;
(h) P. M. Sharp and B. H. Hahn, Cold Spring Harbor. Perspect. Med., 2011, 1, a006841 Search PubMed.
-
(a) MDG 6: 15 YEARS, 15 LESSONS OF HOPE FROM THE AIDS RESPONSE, UNAID http://www.unaids.org;
(b) U. Weiss, Nature, 2001, 410, 961–961 CrossRef CAS.
-
(a) M. P. Girard, S. Osmanov, O. M. Assossou and M.-P. Kieny, Vaccine, 2011, 29, 6191–6218 CrossRef CAS PubMed;
(b) L. Menendez-Arias, Antiviral Res., 2010, 85, 210–231 CrossRef CAS PubMed;
(c) J. N. Blankson, D. Persaud and R. F. Siliciano, Annu. Rev. Med., 2002, 53, 557–593 CrossRef CAS PubMed;
(d) C. C. J. Carpenter, D. A. Cooper, M. A. Fischl, J. M. Gatell, B. G. Gazzard, S. M. Hammer, M. S. Hirsch, D. M. Jacobsen, D. A. Katzenstein, J. S. G. Montaner, D. D. Richman, M. S. Saag, M. Schechter, R. T. Schooley, S. Vella, P. G. Yeni and P. A. Volberding, J. Am. Med. Assoc., 2000, 283, 381–390 CrossRef CAS;
(e) M. A. Fischl, D. D. Richman, M. H. Grieco, M. S. Gottlieb, P. A. Volberding, O. L. Laskin, J. M. Leedom, J. E. Groopman, D. Mildvan, R. T. Schooley, G. G. Jackson, D. T. Durack and D. King, N. Engl. J. Med., 1987, 317, 185–191 CrossRef CAS PubMed.
-
(a) M. S. Cohen, Y. Q. Chen, M. McCauley, T. Gamble, M. C. Hosseinipour, N. Kumarasamy, J. G. Hakim, J. Kumwenda, B. Grinsztejn, J. H. S. Pilotto, S. V. Godbole, S. Mehendale, S. Chariyalertsak, B. R. Santos, K. H. Mayer, I. F. Hoffman, S. H. Eshleman, E. Piwowar-Manning, L. Wang, J. Makhema, L. A. Mills, G. de Bruyn, I. Sanne, J. Eron, J. Gallant, D. Havlir, S. Swindells, H. Ribaudo, V. Elharrar, D. Burns, T. E. Taha, K. Nielsen-Saines, D. Celentano, M. Essex, T. R. Fleming and H. S. Team, N. Engl. J. Med., 2011, 365, 493–505 CrossRef CAS PubMed;
(b) J. K. Rockstroh, J. L. Lennox, E. DeJesus, M. S. Saag, A. Lazzarin, H. Wan, M. L. Walker, X. Xu, J. Zhao, H. Teppler, M. J. DiNubile, A. J. Rodgers, B.-Y. Nguyen, R. Leavitt, P. Sklar and S. Investigators, Clin. Infect. Dis., 2011, 53, 807–816 CrossRef CAS PubMed;
(c) E. M. Connor, R. S. Sperling, R. Gelber, P. Kiselev, G. Scott, M. J. Osullivan, R. Vandyke, M. Bey, W. Shearer, R. L. Jacobson, E. Jimenez, E. Oneill, B. Bazin, J. F. Delfraissy, M. Culnane, R. Coombs, M. Elkins, J. Moye, P. Stratton and J. Balsley, N. Engl. J. Med., 1994, 331, 1173–1180 CrossRef CAS PubMed.
-
(a) A. Persidis, Nat. Biotechnol., 2000, 18, 466–467 CrossRef CAS PubMed;
(b) M. J. Dapp, L. Bonnac, S. E. Patterson and L. M. Mansky, J. Virol., 2014, 88, 354–363 CrossRef PubMed.
-
(a) Overview of HIV Treatments, https://www.aids.gov/hiv-aids-basics/just-diagnosed-with-hiv-aids/treatment-options/overview-of-hiv-treatments/;
(b) Antiretroviral drugs used in the treatment of HIV infection, FDA http://www.fda.gov/ForPatients/Illness/HIVAIDS/Treatment/ucm118915.htm.
-
(a) T. Dorfman, A. Bukovsky, A. Ohagen, S. Hoglund and H. G. Gottlinger, J. Virol., 1994, 68, 8180–8187 CAS;
(b) L. S. Ehrlich, B. E. Agresta and C. A. Carter, J. Virol., 1992, 66, 4874–4883 CAS;
(c) B. M. Forshey, U. von Schwedler, W. I. Sundquist and C. Aiken, J. Virol., 2002, 76, 5667–5677 CrossRef CAS PubMed;
(d) T. Fricke, A. Brandariz-Nunez, X. Wang, A. B. Smith, III and F. Diaz-Griffero, J. Virol., 2013, 87, 10587–10597 CrossRef CAS PubMed;
(e) N. Goudreau, C. T. Lemke, A.-M. Faucher, C. Grand-Maitre, S. Goulet, J.-E. Lacoste, J. Rancourt, E. Malenfant, J.-F. Mercier, S. Titolo and S. W. Mason, ACS Chem. Biol., 2013, 8, 1074–1082 CrossRef CAS PubMed;
(f) Y. Jin, Z. Tan, M. He, B. Tian, S. Tang, I. Hewlett and M. Yang, Bioorg. Med. Chem., 2010, 18, 2135–2140 CrossRef CAS PubMed;
(g) B. N. Kelly, S. Kyere, I. Kinde, C. Tang, B. R. Howard, H. Robinson, W. I. Sundquist, M. F. Summers and C. P. Hill, J. Mol. Biol., 2007, 373, 355–366 CrossRef CAS PubMed;
(h) C. Tang, E. Loeliger, I. Kinde, S. Kyere, K. Mayo, E. Barklis, Y. N. Sun, M. J. Huang and M. F. Summers, J. Mol. Biol., 2003, 327, 1013–1020 CrossRef CAS PubMed;
(i) U. K. von Schwedler, K. M. Stray, J. E. Garrus and W. I. Sundquist, J. Virol., 2003, 77, 5439–5450 CrossRef CAS PubMed.
-
(a)
A. Agarwal, X. Chen, M. Deshpande and M. Summers, WO2006053323, 2006 Search PubMed;
(b)
A. Agarwal, X. Chen, M. Deshpande and M. Summers, US20060100232, 2006 Search PubMed;
(c)
A. Agarwal, X. Chen, M. Deshpande and M. Summers, EP1809380, 2007 Search PubMed.
-
(a) F. S. Asghari and H. Yoshida, Ind. Eng. Chem. Res., 2006, 45, 2163–2173 CrossRef CAS;
(b) J. N. Chheda, Y. Roman-Leshkov and J. A. Dumesic, Green Chem., 2007, 9, 342–350 RSC;
(c) R. Karinen, K. Vilonen and M. Niemela, ChemSusChem, 2011, 4, 1002–1016 CrossRef CAS PubMed;
(d) E. A. Khokhlova, V. V. Kachala and V. P. Ananikov, ChemSusChem, 2012, 5, 783–789 CrossRef CAS PubMed;
(e) S. Lima, M. M. Antunes, M. Pillinger and A. A. Valente, ChemCatChem, 2011, 3, 1686–1706 CrossRef CAS;
(f) J. Lewkowski, ARKIVOC, 2001, 2, 17–54 Search PubMed;
(g) R. A. Sheldon, J. Mol. Catal. A: Chem., 2016, 422, 3–12 CrossRef CAS;
(h) M. Besson, P. Gallezot and C. Pinel, Chem. Rev., 2014, 114, 1827–1870 CrossRef CAS PubMed;
(i) J. D. Lewis, S. Van de Vyver, A. J. Crisci, W. R. Gunther, V. K. Michaelis, R. G. Griffin and Y. Román-Leshkov, ChemSusChem, 2014, 7, 2255–2265 CrossRef CAS PubMed;
(j) P. C. A. Bruijnincx and Y. Roman-Leshkov, Catal. Sci. Technol., 2014, 4, 2180–2181 RSC.
- K. I. Galkin, E. A. Krivodaeva and V. P. Ananikov, Russ. Chem. Bull., 2015, 2954–2957 CrossRef CAS.
- S. Dutta, L. Wu and M. Mascal, Green Chem., 2015, 17, 3737–3739 RSC.
-
(a) T. Kläusli, Green Process. Synth., 2014, 3, 235 Search PubMed;
(b) S. Krawielitzki and T. M. Kläusli, Ind. Biotechnol., 2015, 11, 6–8 CrossRef;
(c)
E. de Jong, M. A. Dam, L. Sipos and G.-J. M. Gruter, in Biobased Monomers, Polymers, and Materials, ed. P. B. Smith and R. A. Gross, American Chemical Society, Washington, DC, 2012, pp. 1–13 Search PubMed;
(d) A. Scott, Chem. Eng. News, 2016, 94, 15 Search PubMed.
- A. Bredihhin, U. Mäeorg and L. Vares, Carbohydr. Res., 2013, 375, 63–67 CrossRef CAS PubMed.
-
(a) V. Burmistrov, C. Morisseau, K. S. S. Lee, D. S. Shihadih, T. R. Harris, G. M. Butov and B. D. Hammock, Bioorg. Med. Chem. Lett., 2014, 24, 2193–2197 CrossRef CAS PubMed;
(b) V. Burmistrov, C. Morisseau, D. Danilov, T. R. Harris, I. Dalinger, I. Vatsadze, T. Shkineva, G. M. Butov and B. D. Hammock, Bioorg. Med. Chem. Lett., 2015, 25, 5514–5519 CrossRef CAS PubMed.
Footnote |
† Electronic supplementary information (ESI) available: Experimental procedures and characterization data. CCDC 1497315. For crystallographic data in CIF or other electronic format see DOI: 10.1039/c6ob01731b |
|
This journal is © The Royal Society of Chemistry 2016 |
Click here to see how this site uses Cookies. View our privacy policy here.