Selective MS screening reveals a sex pheromone in Caenorhabditis briggsae and species-specificity in indole ascaroside signalling†
Received
6th June 2016
, Accepted 24th June 2016
First published on 28th June 2016
Abstract
The indole ascarosides (icas) represent a highly potent class of nematode-derived modular signalling components that integrate structural inputs from amino acid, carbohydrate, and fatty acid metabolism. Comparative analysis of the crude exo-metabolome of hermaphroditic Caenorhabditis briggsae using a highly sensitive mass spectrometric screen reveals an indole ascaroside blend dominated by two new components. The structures of isolated icas#2 and icas#6.2 were determined by NMR spectroscopy and confirmed by total synthesis and chemical correlation. Low atto- to femtomolar amounts of icas#2 and icas#6.2 act in synergism to attract males indicating a function as sex pheromone. Comparative analysis of 14 Caenorhabditis species further demonstrates that species-specific indole ascaroside biosynthesis is highly conserved in the Elegans group. Functional characterization of the dominating indole ascarosides icas#2, icas#3, and icas#9 reveals a high degree of species-specificity and considerable variability with respect to gender-specificity, thus, confirming that indole ascarosides modulate different biological functions within the Elegans group. Although the nematode response was usually most pronounced towards conspecific signals, Caenorhabditis brenneri, the only species of the Elegans group that does not produce any indole ascarosides, exhibits a robust response to icas#2 suggesting the potential for interspecies interactions.
Introduction
Nematode development and behaviour are regulated via the release of ascarosides (ascr),§ a diverse family of glycolipids based on the 3,6-dideoxysugar L-ascarylose.1 In the model organism Caenorhabditis elegans, the dominating ascarosides ascr#3 (1) and ascr#2 (2) (Fig. 1a) function as a strain-specific population density due to induced formation of dauer larva,2,3 and synergistically attract males.4,5 The diversity of biological functions regulated by ascarosides is paralleled by their structural diversity that depends primarily on the variability of their aglycones, which in turn originates from the co-option of a primary metabolic pathway, the peroxisomal β-oxidation of fatty acids, in ascaroside biosynthesis (Fig. 1b).6,7 Very long chain ascarosides of yet unknown origin undergo consecutive chain shortening reactions catalysed by four key enzymes of the β-oxidation pathway (acox-1, maoc-1, dhs-28, daf-22) to afford a homologous series of ascarosides that differ in side chain length and functionality.6,7 Furthermore, the ascaroside skeletons also serve as scaffolds for the attachment of additional structural moieties originating from primary metabolic pathways to form a complex modular library.6,8 The indole ascarosides (icas)§ incorporate an L-tryptophan-derived indole-3-carboxylic acid group attached to the 4-position of the ascarylose moiety and, thus, integrate metabolic inputs from peroxisomal β-oxidation and amino acid metabolism (Fig. 1c).8 The first indole ascaroside called icas#9 (4) was originally detected using bioactivity guided fractionation and described as a potent dauer-inducing population density signal that exhibits an unusual bell-shaped dose response curve.9 Additional components such as icas#3 (3) (Fig. 1c) were discovered via comparative NMR-based metabolomics and shown to affect nematode behaviour by modulating hermaphrodite attraction and aggregation in femto- to picomolar amounts, which are several orders of magnitude smaller than those required for dauer induction.8
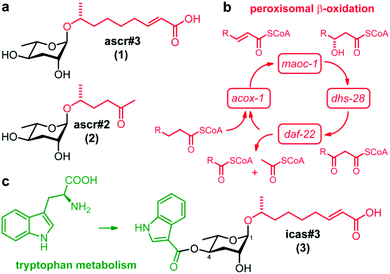 |
| Fig. 1 (a) Dominating ascaroside signals known to modulate C. elegans development and behaviour. (b) Side chain shortening via peroxisomal β-oxidation and the corresponding genes identified in C. elegans. (c) Indole ascarosides such as the highly potent hermaphrodite attractant icas#3 (3) from C. elegans integrate metabolic inputs from peroxisomal β-oxidation and L-tryptophan metabolism. | |
Previous work demonstrated that ascaroside signalling is widely conserved among the nematodes and many basic components are produced by a large diversity of species,10 which raises the question of how species-specific communication is accomplished in sympatric nematodes. Considering the modular assembly of ascaroside signals we hypothesized that species-specific modification of the ascaroside core structures might form the basis for highly dedicated intraspecies signals. In recent years extensive global sampling efforts have resulted in the description of many additional Caenorhabditis species11,12 and expanded our understanding of their ecology and natural history.13–15 Although no direct sister species of C. elegans has yet been described, the Caenorhabditis genus constitutes an invaluable tool for comparative biological studies. Here we utilize this unique resource for a comparative analysis of indole ascaroside signalling in a collection of 14 Caenorhabditis species. Using a highly sensitive mass spectrometric screen that facilitates selective detection of indole ascarosides in crude nematode exo-metabolome extracts we found that their biosynthesis is highly conserved in the Elegans group and displays considerable species-specificity. We identified new indole ascarosides from Caenorhabditis briggsae and characterized their biosynthesis and function in the regulation of nematode behaviour in nine members of the Elegans group. Our results show that indole ascarosides represent species-specific signals that modulate different gender-specific biological functions.
Results and discussion
Selective MS screening reveals C. briggsae-specific indole ascarosides
Considering that behaviour modulating indole ascarosides are active in extremely low amounts8 we aimed to take advantage of the outstanding sensitivity of mass spectrometry to screen for indole ascarosides in crude nematode exo-metabolome extracts. Previous MS/MS analysis using electrospray ionization in negative ion mode (ESI-(−)) demonstrated that fragmentation of indole ascarosides carrying carboxylic acid aglycones such as icas#9 (4) involves loss of the indole-3-carbonyl moiety to give side chain-specific fragments (Fig. 2a).6,8 In contrast, high resolution mass spectrometric (HR-MS) analysis of icas#9 (4) and its methyl ester 5 using electrospray ionization in positive ion mode (ESI-(+)) revealed a characteristic and highly intensive fragment ion signal for C15H16NO4+ (m/z 274.1074) that originates from the loss of the side chain upon in source collision induced dissociation (ISCID) (Fig. 2a, S1 and S2†). ESI-(+)-HR-MS screening of the Caenorhabditis elegans (N2) exo-metabolome, known to contain the dominating indole ascarosides icas#3 (3) and icas#9 (4)8,9 for the [C15H16NO4]+ fragment gave signals corresponding exclusively to indole ascarosides (Fig. 2b), which facilitates their selective detection in crude metabolome extracts.
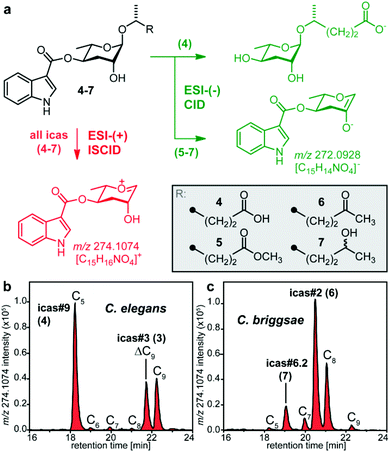 |
| Fig. 2 (a) Fragmentation of indole ascarosides upon ESI-(−) (green) depends on the functionality of the side chain whereas those upon ESI-(+) (red) indiscriminately results in the loss of the aglycone to give a characteristic [C15H16NO4]+ fragment (red); (b) HPLC-ESI-(+)-HR-MS screening of the crude C. elegans (N2) exo-metabolome extract for the [C15H16NO4]+ fragment gives signals for indole ascarosides only; (c) HPLC-ESI-(+)-HR-MS screening of the C. briggsae (AF16) exo-metabolome extract for the [C15H16NO4]+ fragment reveals a species-specific indole ascaroside blend including two new components identified as icas#2 (6) and icas#6.2 (7). | |
ESI-(+)-HR-MS screening of the previously unexplored exo-metabolome from the closely related hermaphroditic Caenorhabditis briggsae strain AF16 from India (Fig. 2c) revealed an indole ascaroside profile considerably different from that of C. elegans. Comparative analysis indicated that two of the dominating indole ascarosides produced by C. briggsae (icas#2 (6), icas#6.2 (7)) do not correspond to any of the components previously identified in C. elegans, demonstrating that selective ESI-(+)-MS screening enables detection of novel species-specific indole ascarosides. Targeted LC-MS analysis of 13 wild-type isolates including tropical and temperate strains16,17 demonstrated that production of icas#2 (6) and icas#6.2 (7) is highly conserved in cosmopolitan C. briggsae (Fig. S5†). The structure assignment of these potential signalling molecules was pursued by complementing the ESI-(+)-MS screen with an ESI-(−)-HR-MS/MS analysis that indicated the molecular formulas of C21H27NO6 (m/z 388.1775 [M − H]−, Δ 2.3 ppm) and C21H29NO6 (m/z 390.1915 [M − H]−, Δ 1.8 ppm) along with fragment ion signals for [C15H14NO4]− from loss of the side chains (Fig. 2a, S3 and S4†), which suggested indole ascaroside structures carrying six carbon side-chains with a carbonyl (6) or hydroxyl group (7), respectively. To corroborate these assignments approximately 150 μg icas#2 (6) and 170 μg icas#6.2 (7) were isolated from 1.5 litres of C. briggsae liquid culture medium using a combination of reverse phase C18 solid phase extraction (SPE) and HPLC. Analysis of two-dimensional NMR spectra (see the ESI†) confirmed a 4-substituted ascarylose unit, an indole-3-carboxylate moiety, and (ω-1)-oxygenated side chains with an additional methyl ketone moiety in the case of icas#2 (6) or a 2-hydroxyalkyl moiety in the case of icas#6.2 (7).
The structures of the natural compounds were finally established by synthesis as shown in Fig. 3. Starting from 8, which is available in seven steps from L-rhamnose,5 icas#2 (6) was obtained in four steps as previously described.18 Icas#6.1 (14) was prepared via the TBS ether 11. Hydrolysis of the benzoyl groups to 12 and reaction with indole-3-carbonyl chloride was followed by separation of the resulting 2- and 4-substituted monoesters (13) and TBS-deprotection to afford (R)-icas#6.1 (14). Comparative analysis of the 1H NMR spectra of synthetic (R)-icas#6.1 (14) and the material isolated from C. briggsae indicated small differences in chemical shifts that were most pronounced for the terminal methyl group of the side chain, thus, suggesting an (S)-configuration for natural icas#6.2 (7). The absolute stereochemistry of icas#6.2 (7) was finally established by chemical correlation with the diastereoisomeric mixture of the NaBH4 reduction products of synthetic icas#2 (6) as well as icas#6.1 (14) for comparison (Fig. 3 and S6†). The corresponding non-indole derivatives, the dauer-inducing ascr#2 (2)2 and diastereoisomeric ascr#6.1 and ascr#6.2 of unknown function5 in C. elegans have previously been described and were now also detected in C. briggsae (Fig. S7†). Furthermore, icas#2 (6) has previously been described as a synthetic compound that mimics icas#9 (4) in exhibiting dauer-inducing activity in C. elegans.18
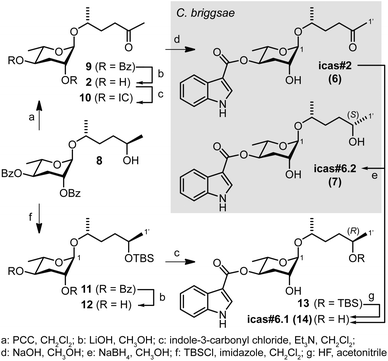 |
| Fig. 3 Synthesis of indole ascarosides detected in C. briggsae. | |
Indole ascarosides icas#2 (6) and icas#6.2 (7) synergistically attract C. briggsae males
The behavioural activities of the species-specific indole ascarosides from C. briggsae were evaluated using a holding assay by measuring the time nematodes spent within a circular scoring region conditioned with indole ascarosides in comparison to the control regions.19 We found that amounts as low as 1–25 fmol icas#2 (6) (Fig. 4a) or 1–2.5 fmol icas#6.2 (7) (Fig. 4b) result in significantly increased holding times for young adult males of the tropical C. briggsae strain AF16 from India, whereas hermaphrodites are not affected over a wide range of concentrations (0.01–10
000 fmol). A similar male-specific response to icas#2 (6) or icas#6.2 (7) was also observed for the temperate C. briggsae strain HK104 from Japan (Fig. S8†). In contrast to (S)-icas#6.2 (7) nematodes are not retained by the diastereoisomeric (R)-icas#6.1 (14) (Fig. S9†), demonstrating that behavioural activity depends on the (S)-configuration of the side chain.
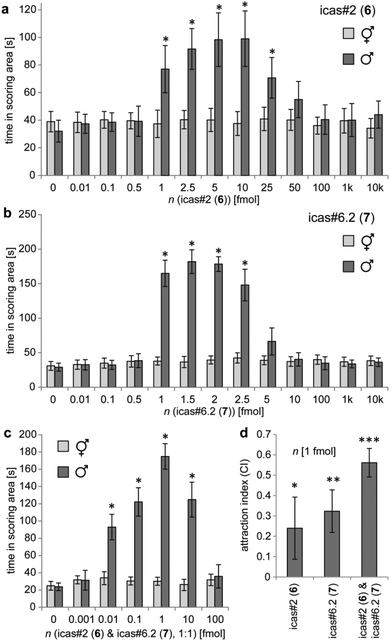 |
| Fig. 4 Indole ascarosides icas#2 (6) and icas#6.2 (7) act as a synergistic male-attractant in C. briggsae. (a) C. briggsae AF16 males are retained in holding assays with 1–25 fmol icas#2 (6); (b) C. briggsae AF16 males are retained in holding assays with 1–2.5 fmol icas#6.2 (7); (c) equimolar mixtures of atto- to femtomolar amounts of icas#2 (6) and icas#6.2 (7) synergistically retain C. briggsae AF16 males in holding assays; (d) femtomolar amounts of icas#2 (6), icas#6.2 (7), and their synergistic blend attract C. briggsae AF16 males in the 4-spot attraction assay (a–c: one-way ANOVA with Dunett's post-test, *p < 0.001; d: students’ t test with Welch's correction, *p < 0.05, **p < 0.01, ***p < 0.001). | |
Because hermaphroditic C. briggsae release a blend of indole ascarosides (Fig. S5†) we asked how the combinations of the dominating components icas#2 (6) and icas#6.2 (7) modulate male behaviour. Holding assays using equimolar mixtures of 6 and 7 indicate significant retention by amounts as small as 10 attomol each (Fig. 4c), which do not display any activity on their own (Fig. 4a and b), demonstrating that indole ascarosides exhibit synergistic activities. Furthermore, C. briggsae males are retained by this equimolar blend in amounts covering several orders of magnitude (0.01–10 fmol) (Fig. 4c), whereas the preferred concentration ranges for the single components are much more restricted (Fig. 4a and b). To confirm that the observed increase in male holding times in regions conditioned with icas#2 (6) and icas#6.2 (7) corresponds to nematode attraction, we evaluated C. briggsae male behaviour in a 4-spot attraction assay,19 which showed significant attraction that was most pronounced for the equimolar mixture (Fig. 4d). Taken together these results demonstrate that in C. briggsae a hermaphrodite-produced species-specific blend of icas#2 (6) and icas#6.2 (7) acts in synergism to attract males, suggesting its function as a sex pheromone in this facultative outcrossing species.
Species-specific indole ascaroside biosynthesis is highly conserved within the Elegans group
Having discovered that behavioural signalling in C. briggsae and C. elegans involves species-specific components, we aimed for a comparative analysis of indole ascaroside biosynthesis in a collection of 14 Caenorhabditis species with special emphasis on the Elegans group that harbours the model organisms C. elegans and C. briggsae (Fig. 5a). Targeted metabolomics using the indole ascaroside-selective ESI-(+)-HR-MS screen demonstrated that their production is highly conserved within the Elegans group. Of the nine Elegans group members that were analysed, all but C. brenneri were found to produce indole ascarosides. In contrast, indole ascarosides were not detected in any of the representative members of the non-Elegans group such as C. japonica, C. afra, C. portoensis, C. virilis, or the most basal Caenorhabditis species 1, indicating that indole ascaroside signalling is much less prominent outside of the Elegans group.
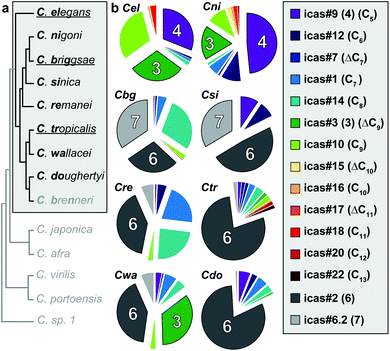 |
| Fig. 5 Species-specific indole ascaroside biosynthesis is highly conserved in the Elegans group. (a) Phylogeny of the analysed Caenorhabditis species11 with the Elegans group highlighted, hermaphroditic species underlined, 3-letter identifier in bold,12 and indole ascaroside producing species shown in black; (b) species-specific compositions of indole ascaroside blends in 8 representative members of the Elegans group with icas#3 (3), icas#9 (4), icas#2 (6), and icas#6.2 (7) as dominating components (Cx = side chain length of the aglycone). | |
Comparative analysis of indole ascaroside compositions further demonstrated that their biosynthesis displays a high degree of species-specificity (Fig. 5b). Indole ascaroside profiles of C. elegans and C. nigoni are dominated by icas#3 (3) and icas#9 (4), whereas those of C. briggsae, C. sinica, C. remanei, C. tropicalis, and C. doughertyi are dominated by icas#2 (6). C. wallacei produces a blend of icas#3 (3) and icas#2 (6). In contrast to the species-specific indole ascarosides icas#3 (3) and icas#2 (6) their biosynthetic precursors ascr#3 (1) and ascr#2 (2) were detected in almost all members of the Elegans group (ascr#2 was absent in C. nigoni and only traces of ascr#3 were detectable in C. briggsae) indicating that indole ascaroside biosynthesis through attachment of tryptophan-derived indole-3-carboxylic acid is tightly regulated.
Quantitative LC-MS analysis of high density cultures grown for 15 days indicated that the most prominent components accumulated in concentrations around 100 nM, whereas extraordinarily high concentrations of 750 nM icas#2 (6) or 1.6 μM icas#9 (4) were detected in C. tropicalis and C. nigoni cultures, respectively (Fig. S10†). Furthermore, ascaroside selective precursor ion screening6 revealed the presence of many known as well as several yet undescribed ascaroside derivatives in the various analysed Caenorhabditis species. Some of these putative signalling molecules, whose molecular structures and biological functions are currently being investigated, display high levels of species-specificity, whereas others are more widely distributed.
Behavioural response to indole ascaroside signals is highly species-specific
Having discovered that indole ascarosides modulate different functions in hermaphroditic C. briggsae (exclusively male attractant) and C. elegans (predominantly hermaphrodite attractant and aggregation signal),8 we characterized the behavioural activity of the three dominating components, icas#2 (6), icas#3 (3), and icas#9 (4), in nine members of the Caenorhabditis elegans group using the holding assay (Fig. 6).19 These analyses revealed a high degree of species-specificity and considerable variability with respect to gender-specificity, thus, confirming that indole ascarosides modulate different biological functions within the Elegans group. Icas#2 (6) exhibits male-specific activity in the closely related C. briggsae and C. sinica as well as distantly related hermaphroditic C. tropicalis, suggesting its function as a sex pheromone. In contrast icas#2 (6) affects both males and females in gonochoristic C. remanei, C. doughertyi, and C. wallacei. Similarly, we found that icas#3 (3) and icas#9 (4) affect both genders of C. elegans and C. wallacei but only males of C. nigoni. Characterizing the behavioural response over a wide range of concentrations (1–10
000 fmol μl−1) revealed almost exclusively bell-shaped dose response curves suggesting nematode preferences to well defined concentration ranges. Comparison of the responses to conspecific signals (that are produced by the responding species) versus heterospecific signals (that are only produced by organisms other than the responding species) indicated considerable self-preference in most species. For example, C. briggsae and C. tropicalis were not attracted by heterospecific icas#3 (3). In C. elegans and C. nigoni as well as C. doughertyi and C. remanei, the response to heterospecific indole ascarosides required considerably larger amounts. However, we found that males of C. brenneri, the only species of the Elegans group that does not produce any indole ascarosides, exhibit a robust response to low femtomolar amounts of icas#2 (6) and icas#9 (4), suggesting an ability to eavesdrop on heterospecific communication.
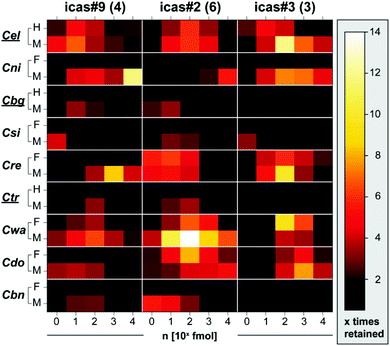 |
| Fig. 6 Response to indole ascarosides is species and gender-specific. Heatmap showing nematode retention with an increase of holding times in regions conditioned with 1–10 000 fmol of three indole ascarosides (icas#3 (3), icas#9 (4), or icas#2 (6)) in comparison to solvent control for both genders of nine Caenorhabditis species (H: hermaphrodite, F: female, M: male; see Fig. S11a–c† for details). | |
Conclusions
In conclusion we have established a highly selective and sensitive MS screen to analyse indole ascaroside biosynthesis in crude nematode exo-metabolome extracts which revealed significant species-specificity in the members of the Caenorhabditis elegans group. Using comparative metabolomics, traditional isolation techniques, and total synthesis, we identified two species-specific indole ascarosides, icas#2 (6) and icas#6.2 (7), which are produced by C. briggsae hermaphrodites and synergistically attract conspecific males in attomolar amounts indicating their function as a sex pheromone in this facultative outcrossing species. Comparative analysis of several Caenorhabditis species further demonstrated that indole ascaroside signalling is highly conserved among the Elegans group and that icas#2 (6) constitutes an abundant signal which, however, elicits different responses in different species. The high degree of species-specificity in production and the response to indole ascarosides within the members of the Elegans group do not correlate with their phylogenetic relationship suggesting that indole ascaroside signalling within the Caenorhabditis evolved rapidly. Taken together, our results demonstrate that the Caenorhabditis genus constitutes a highly suited resource for comparative metabolomics. Results from these studies when correlated with comparative genomics and transcriptomics might facilitate the characterization of novel signalling pathways and finally lead to a holistic understanding of these model organisms in an ecological and evolutionary context.
Experimental
Nematode strains and general culture methods
Thirteen wild-type isolates of Caenorhabditis briggsae including the five tropical strains: AF16 (India), JU725 (China), ED3034 (Taiwan), ED3083 (South Africa), VT847 (Hawaii), the five temperate strains: HK104 (Japan), PB800 (Ohio, US), EG4181 (Utah, US), JU516 (France), JU439 (Iceland), and three outgroup strains: QR24 (Canada), ED3101 (Kenya), JU1341 (India);16,17 as well as 13 wild-type isolates of related Caenorhabditis species:11C. elegans N2 (Bristol), C. remanei PB4641 (USA), C. wallacei (sp. 16) JU1904 (Indonesia), C. sinica (sp. 5) JU727 (China), C. brenneri (sp. 4) PB2801 (Costa Rica), C. nigoni (sp. 9) JU1422 (India), C. doughertyi (sp. 10) JU1771 (India), C. tropicalis (sp. 11) JU1373 (La Reunion), C. japonica DF5081 (Japan), C. afra (sp. 7) JU1286 (Ghana), C. portoensis (sp. 6) EG4788 (Portugal), C. virilis (sp. 13) JU1968 (France), and C. sp. 1 JU1667 (Germany) were cultivated at 23 °C on solidified nematode growth medium (NGM agar) seeded with E. coli OP50.20 Androdioecious populations of hermaphroditic C. briggsae (AF16, HK104), C. elegans (N2), and C. tropicalis (JU1373) were generated by heat shock of late L4 hermaphrodites at 30 °C for 6 h and subsequently maintained by mating.
Preparation of exo-metabolome extracts
Cultures were established using mixed stage nematodes from five 10 cm plates collected in M9 buffer as inoculums and grown in 100 ml S complete medium at 23 °C and 150 rpm.20 Concentrated E. coli OP50 bacteria pellets from an overnight culture in LB medium at 37 °C and 170 rpm were provided as food on day 1 (3 ml), day 2 (3 ml), day 3 (3 ml), day 4 (5 ml), and day 5 (10 ml). After 15 days nematodes were separated by centrifugation (5 min at 500g). The filtered supernatant representing the exo-metabolome was frozen at −80 °C, lyophilized, and extracted with 3 × 100 ml methanol for 12 h each. The combined extract was filtered and concentrated to dryness at 40 °C under reduced pressure. The metabolome extract was further concentrated by subsequent extraction of the residues with decreasing amounts of methanol and concentration of the supernatants at 40 °C under reduced pressure. The resulting material was dissolved in 1 ml methanol for HPLC-MS analysis. All experiments were performed in triplicate.
HPLC-HR-MS/MS analysis and indole ascaroside screening
HPLC-ESI-HR-MS/MS analysis was performed using a Dionex UltiMate 3000 HPLC instrument coupled to a Bruker Maxis ultrahigh resolution (UHR) qTOF mass spectrometer equipped with an electrospray ionization (ESI) unit operated in positive or negative mode. Chromatographic separations were achieved using an Agilent ZORBAX Eclipse XDB-C18 column (250 × 3 mm, 5 μm) with a flow rate of 400 μl min−1 and gradient elution starting at 3% acetonitrile in 0.5% aqueous acetic acid for 5 minutes followed by a linear increase to 100% acetonitrile with 0.5% acetic acid within 35 minutes. Quantification of indole ascarosides was performed by comparison with authentic standards of known concentration using three independent replicates.
Isolation of indole ascarosides from C. briggsae strain AF16
The combined exo-metabolome extract from fifteen 100 ml liquid cultures was adsorbed on 1 g of Celite and fractionated by reverse phase solid phase extraction using 5 g of C18 modified silica as the stationary phase (Chromabond, Macherey-Nagel) and a serial stepwise increase of methanol in water as the eluent. The fraction eluted with 70% methanol containing the indole ascarosides (Fig. S12†) was further fractionated by semi-preparative HPLC using an Agilent HP-1100 HPLC instrument equipped with a Grom-Sil 120 ODS-4 HE column (250 × 8 mm, 5 μm) coupled to a Gilson 206 Abimed fraction collector. A flow rate of 2 ml min−1 with gradient elution was used starting at 3% acetonitrile in 0.5% aqueous acetic acid for 3 minutes, followed by a linear increase to 100% acetonitrile with 0.5% acetic acid within 30 minutes. Fractions were analyzed by HPLC-MS, concentrated under reduced pressure, and dried under vacuum to afford 150 μg icas#2 (6) (Fig. S13–S16†) and 170 μg icas#6.2 (7) (Fig. S17–S19†).
NMR spectroscopy
NMR spectra were recorded in CD3OD or CDCl3 at 400 MHz for 1H and 100 MHz for 13C using a Bruker AMX400 instrument. Residual solvent signals were used as internal standard with 1H at 3.31 ppm and 13C at 49.05 ppm for CD3OD or 1H at 7.26 ppm and 13C at 77.16 ppm for CDCl3. Two-dimensional homonuclear double quantum filtered (dqf)-COSY spectra were recorded using phase cycling for coherence selection. A total of 32 scans were acquired using a time domain of 8k in F2 (acquisition time of 1.2 s) and 512 increments in F1. For two dimensional heteronuclear HSQC spectra, 96 scans were acquired using a time domain of 1k in F2 and 256 increments in F1. Spectra were zero-filled to 8k × 4k (COSY) or 4k × 2k (HSQC), Fourier transformed, and manually phased using the Bruker Topspin software.
Indole ascaroside icas#2 (6) from C. briggsae.
1H NMR (400 MHz, CD3OD) δ: 1.18 (3H, d, J = 6.1 Hz, 6′-H), 1.24 (3H, d, J = 6.2 Hz, 6-H), 1.83 (2H, m, 4′-H), 2.01 (1H, ddd, 2J = 13.8 Hz, J = 11.2 Hz, J = 3.1 Hz, 3-Hax), 2.20 (3H, s, 1′-H), 2.21 (1H, dt, 2J = 13.7, J = 4.0 Hz, 3-Heq), 2.69 (2H, m, 3′-H), 3.79 (1H, s.br, 2-H), 3.87 (1H, m, 5′-H), 4.00 (1H, dq, J = 9.4 Hz, J = 6.2 Hz, 5-H), 4.74 (1H, s, 1-H), 5.12 (1H, ddd, J = 10.7 Hz, J = 9.6 Hz, J = 4.7 Hz, 4-H), 7.19 (1H, m, 5′′-H), 7.21 (1H, m, 6′′-H), 7.45 (1H, d, J = 7.2 Hz, 7′′-H), 7.98 (1H, s, 2′′-H), 8.04 (1H, d, J = 6.9 Hz, 4′′-H); 13C NMR (100 MHz, CD3OD) δ: 17.9 (6-C), 18.9 (6′-C), 29.6 (1′-C), 31.9 (4′-C), 33.1 (3-C), 40.3 (3′-C), 68.8 (5-C), 69.3 (2-C), 70.5 (4-C), 71.9 (5′-C), 97.7 (1-C), 112.7 (7′′-C), 121.7 (4′′-C), 122.3 (5′′-C), 123.5 (6′′-C), 133.1 (2′′-C); ESI-(−)-HR-MS: obs. m/z 388.1775 [M − H]−, calc. m/z 388.1766 for C21H26NO6, Δ 2.3 ppm.
Indole ascaroside (S)-icas#6.2 (7) from C. briggsae.
1H NMR (400 MHz, CD3OD) δ: 1.19 (3H, d, J = 6.1 Hz, 6′-H), 1.22 (3H, d, J = 6.2 Hz, 1′-H), 1.24 (3H, d, J = 6.3 Hz, 6-H), 1.55 (1H, m, 3′-Ha), 1.62 (1H, m, 4′-Ha), 1.68 (2H, m, 3′-Hb, 4′-Hb), 2.02 (1H, ddd, 2J = 13.2 Hz, J = 11.1 Hz, J = 3.2 Hz, 3-Hax), 2.22 (1H, dt, 2J = 13.3 Hz, J = 4.1 Hz, 3-Heq), 3.78 (1H, m, 2′-H), 3.80 (1H, s.br, 2-H), 3.85 (1H, m, 5′-H), 4.08 (1H, dq, J = 9.6 Hz, J = 6.4 Hz, 5-H), 4.76 (1H, s, 1-H), 5.13 (1H, ddd, J = 10.7 Hz, J = 9.7 Hz, J = 4.7 Hz, 4-H), 7.18 (1H, m, 5′′-H), 7.21 (1H, m, 6′′-H), 7.45 (1H, d, J = 7.4 Hz, 7′′-H), 7.96 (1H, s, 2′′-H), 8.02 (1H, d, J = 6.9 Hz, 4′′-H); 13C NMR (100 MHz, CD3OD) δ: 18.0 (6-C), 19.1 (6′-C), 23.3 (1′-C), 33.3 (3-C), 34.4 (4′-C), 36.3 (3′-C), 68.5 (2′-C), 68.7 (5-C), 69.5 (2-C), 70.5 (4-C), 73.3 (5′-C), 97.8 (1-C), 112.9 (7′′-C), 121.7 (4′′-C), 122.4 (5′′-C), 123.6 (6′′-C), 133.2 (2′′-C); ESI-(−)-HR-MS: obs. m/z 390.1915 [M − H]−, calc. m/z 390.1922 for C21H28NO6, Δ 1.8 ppm.
Synthesis of indole ascarosides
Known indole ascarosides such as icas#3 (3),8 icas#9 (4),8,9 and icas#2 (6)18 were synthesized as previously described.
Synthesis of icas#9 methyl ester (5).
20 μl of a 50 μM icas#9 (4) solution (1 nmol) in methanol was diluted with 500 μl methanol and treated with 5 μl of a 2 M trimethylsilyl diazomethane solution (10 μmol) in diethyl ether. After stirring for 30 min, the excess reagent was quenched by addition of 5 μl acetic acid (87 μmol) and the solution concentrated to dryness under reduced pressure. The residue was taken up in 20 μl methanol and analyzed by HPLC-ESI-HR-MS/MS (see Fig. S2†). ESI-(−)-HR-MS: obs. m/z 404.1722 [M − H]−, calc. m/z 404.1715 for C21H26NO7, Δ 1.7 ppm.
Synthesis of 2,4-di-O-benzoyl-2′-O-TBS-ascr#6.1 (11).
Under an argon atmosphere a solution of 50 mg 2,4-di-O-benzoyl-ascr#6.1 (8)5 (109 μmol) and 41 mg imidazole (602 μmol) in 1 ml dry dichloromethane was treated with 90 mg of tert-butyldimethylsilyl chloride (597 μmol). After stirring at room temperature for 20 h the reaction was washed with 3 × 1 ml water, dried over Na2SO4, and concentrated under reduced pressure to afford crude 2,4-di-O-benzoyl-2′-O-TBS-ascr#6.1 (11) that was used without further purification. 1H NMR (400 MHz, CDCl3) δ: 0.08 (6H, s, Si(CH3)2), 0.90 (9H, s, t-Bu), 1.18 (3H, d, J = 6.1 Hz, 6′-CH3), 1.20 (3H, d, J = 6.1 Hz, 1′-CH3), 1.28 (3H, d, J = 6.3 Hz, 6-CH3), 1.51 (2H, m, 3′,4′-H), 1.67 (2H, m, 3′,4′-H), 2.21 (1H, ddd, 2J = 13.6 Hz, J = 11.5 Hz, J = 3.1 Hz, 3-Hax), 2.42 (1H, dt, 2J = 13.5 Hz, J = 3.6 Hz, 3-Heq), 3.85 (2H, m, 2′,5′-H), 4.13 (1H, dq, J = 9.8 Hz, J = 6.3 Hz, 5-H), 4.96 (1H, s.br, 1-H), 5.15 (1H, s.br, 2-H), 5.19 (1H, ddd, J = 11.3 Hz, J = 9.8 Hz, J = 4.6 Hz, 4-H), 7.46 (4H, m, 3′′,5′′-H, 3′′′,5′′′-H), 7.58 (2H, m, 4′′,4′′′-H), 8.04 (2H, m, 2′′,6′′-H), 8.12 (2H, m, 2′′′,6′′′-H); 13C NMR (100 MHz, CDCl3) δ: −4.5, −4.2, 18.0, 18.3, 19.3, 23.9, 26.1, 29.9, 33.3, 35.8, 67.1, 68.5, 70.8, 71.4, 72.6, 93.9, 128.6, 128.6, 129.8, 130.0, 130.1, 130.2, 133.3, 133.4, 165.8, 165.9; ESI-(+)-HR-MS: obs. m/z 593.2919 [M + Na]+, calc. m/z 593.2905 for C32H46NaO7Si, Δ +2.4 ppm.
Synthesis of 2′-O-TBS-ascr#6.1 (12).
A solution of crude 2,4-di-O-benzoyl-2′-O-TBS-ascr#6.1 (11) in 500 μl methanol was treated with 30 μl of a saturated aqueous lithium hydroxide solution. After stirring at room temperature for 12 h the reaction was concentrated under reduced pressure and purified by column chromatography on silica gel using a mixture of 5% methanol in dichloromethane as the eluent to afford 16.6 mg 2′-O-TBS-ascr#6.1 (12) as a colourless oil (45.8 μmol, 42% yield over two steps). 1H NMR (400 MHz, CD3OD) δ: 0.07 (6H, s, Si(CH3)2), 0.90 (9H, s, t-Bu), 1.13 (3H, d, J = 6.0 Hz, 6′-CH3), 1.15 (3H, d, J = 6.1 Hz, 1′-CH3), 1.21 (3H, d, J = 6.2 Hz, 6-CH3), 1.50 (1H, m, 3′-Ha), 1.51 (1H, m, 4′-Ha), 1.56 (1H, m, 3′-Hb), 1.64 (1H, m, 4′-Hb), 1.76 (1H, ddd, 2J = 13.0 Hz, J = 11.3 Hz, J = 3.0 Hz, 3-Hax), 1.95 (1H, dt, 2J = 13.1 Hz, J = 3.8 Hz, 3-Heq), 3.52 (ddd, J = 11.1 Hz, J = 9.5 Hz, J = 4.5 Hz, 4-H), 3.62 (dq, J = 9.3 Hz, J = 6.2 Hz, 5-H), 3.72 (1H, s.br, 2-H), 3.78 (1H, m, 5′-H), 3.87 (1H, m, 2′-H), 4.64 (1H, s.br, 1-H); 13C NMR (100 MHz, CD3OD) δ: −4.6 (Si(CH3)2), 17.8 (6-C), 19.0 (t-Bu), 19.1 (6′-C), 24.0 (1′-C), 26.1 (t-Bu), 34.2 (4′-C), 35.7 (3-C), 36.4 (3′-C), 68.1 (4-C), 69.4 (2′-C), 69.8 (2-C), 71.0 (5-C), 72.5 (5′-C), 97.6 (1-C); obs. m/z 385.2378 [M + Na]+, calc. m/z 385.2381 for C18H38NaO5Si, Δ +0.8 ppm.
Synthesis of 4-O-(indole-3-carbonyl)-2′-O-TBS-ascr#6.1 (13).
A well-stirred suspension of 44.5 mg indole-3-carboxylic acid (275 μmol) in 1 ml dry DCM at 0 °C was treated with 20 ml dry DMF and 40 μl thionyl chloride (550 μmol). The mixture was stirred for 30 min at room temperature to yield an intensive red and clear solution that was concentrated to dryness under reduced pressure, dried under high vacuum, and was used without further purification. Under an argon atmosphere a well stirred solution of 10 mg 2′-O-TBS-ascr#6.1 (12) (28 μmol) and 140 μl diisopropylethylamine (106.9 mg, 827 μmol) in 1 ml dry dichloromethane at −20 °C was treated dropwise with the crude indole-3-carbonyl chloride in 1 ml dry DCM over the course of 10 min. After stirring at room temperature for 2 h the reaction was quenched by addition of 200 μl saturated aqueous NaHCO3 solution, dried over Na2SO4, and concentrated to dryness under reduced pressure. The residue was purified by reverse phase solid phase extraction on the 1 g C18ec phase (Chromabond, Macherey-Nagel) using a stepwise gradient of methanol in water as the eluent. The fraction eluted with 90% methanol was further purified by column chromatography on silica gel using a mixture of 2% ethyl acetate in hexane as the eluent to afford 1.29 mg 4-O-(indole-3-carbonyl)-2′-O-TBS-ascr#6.1 (13) as a colourless oil (2.6 μmol; yield 9%). 1H NMR (400 MHz, CD3OD) δ: 0.10 (3H, s, SiCH3), 0.11 (3H, s, SiCH3), 0.91 (9H, s, t-Bu), 1.17 (3H, d, J = 6.2 Hz, 6′-CH3), 1.19 (3H, d, J = 6.1 Hz, 1′-CH3), 1.24 (3H, d, J = 6.2 Hz, 6-CH3), 1.54 (1H, m, 4′-Ha), 1.55 (1H, m, 3′-Ha), 1.66 (1H, m, 3′-Hb), 1.70 (1H, m, 4′-Hb), 2.02 (1H, ddd, 2J = 13.1 Hz, J = 11.2 Hz, J = 3.0 Hz, 3-Hax), 2.22 (1H, dt, 2J = 13.0 Hz, J = 3.7 Hz, 3-Heq), 3.80 (1H, s.br, 2-H), 3.84 (1H, m, 5′-H), 3.91 (1H, m, 2′-H), 4.06 (1H, dq, J = 9.3 Hz, J = 6.3 Hz, 5-H), 4.75 (1H, s.br, 1-H), 5.13 (1H, ddd, J = 11.6 Hz, J = 9.4 Hz, J = 4.6 Hz, 4-H), 7.17 (1H, m, 5′′-H), 7.21 (1H, m, 6′′-H), 7.45 (1H, d, J = 7.9 Hz, 7′′-H), 7.94 (1H, s, 2′′-H), 8.01 (1H, d, J = 7.8 Hz, 4′′-H); 13C NMR (100 MHz, CD3OD) δ: −4.6 (Si(CH3)2), 17.9 (6-C), 18.9 (t-Bu), 19.2 (6′-C), 23.9 (1′-C), 26.1 (t-Bu), 33.3 (3-C), 34.3 (4′-C), 36.5 (3′-C), 68.7 (5-C), 69.5 (2-C), 69.6 (2′-C), 70.6 (4-C), 72.9 (5′-C), 98.0 (1-C), 108.4 (3′′-C), 112.7 (7′′-C), 121.7 (4′′-C), 122.3 (5′′-C), 123.4 (6′′-C), 127.2 (3a′′-C), 133.0 (2′′-C), 137.8 (7a′′-C); ESI-(+)-HR-MS: obs. m/z 528.2752 [M + Na]+, calc. m/z 528.2746 for C27H43NNaO6Si, Δ +1.1 ppm.
Synthesis of 4-O-(indole-3-carbonyl)-ascr#6.1 ((R)-icas#6.1) (14).
A solution of 1.29 mg 4-O-(indole-3-carbonyl)-2′-O-TBS-ascr#6.1 (13) (2.6 μmol) in 500 μl acetonitrile was treated with 10 μl of 1 M hydrofluoric acid. After stirring at RT for 1 h the solution was concentrated under reduced pressure. The product was isolated by reverse phase solid phase extraction on the 1 g C18ec phase (Chromabond, Macherey-Nagel) using a stepwise gradient of methanol in water as the eluent to afford 0.95 mg 4-O-(indole-3-carbonyl)-ascr#6.1 ((R)-icas#6.1) (14) as a colourless oil (2.4 μmol, 92% yield). 1H NMR (400 MHz, CD3OD) δ: 1.19 (3H, d, J = 6.1 Hz, 6′-H), 1.22 (3H, d, J = 6.2 Hz, 1′-H), 1.24 (3H, d, J = 6.3 Hz, 6-H), 1.56 (1H, m, 3′-Ha), 1.58 (1H, m, 4′-Ha), 1.67 (1H, m, 3′-Hb), 1.74 (1H, m, 4′-Hb), 2.03 (1H, ddd, J = 12.9 Hz, J = 11.0 Hz, J = 3.0 Hz, 3-Hax), 2.22 (1H, dt, J = 12.9 Hz, J = 4.0 Hz, 3-Heq), 3.79 (1H, m, 2′-H), 3.80 (1H, s.br, 2-H), 3.88 (1H, m, 5′-H), 4.06 (1H, dq, J = 9.5 Hz, J = 6.3 Hz, 5-H), 4.76 (1H, s, 1-H), 5.13 (1H, ddd, J = 11.2 Hz, J = 9.6 Hz, J = 4.8 Hz, 4-H), 7.18 (1H, m, 5′′-H), 7.21 (1H, m, 6′′-H), 7.45 (1H, d, J = 7.3 Hz, 7′′-H), 7.96 (1H, s, 2′′-H), 8.01 (1H, d, J = 7.0 Hz, 4′′-H); 13C NMR (100 MHz, CD3OD) δ: 18.0 (6-C), 19.2 (6′-C), 23.5 (1′-C), 33.3 (3-C), 34.3 (4′-C), 36.2 (3′-C), 68.1 (2′-C), 68.7 (5-C), 69.5 (2-C), 70.6 (4-C), 72.7 (5′-C), 97.8 (1-C), 112.9 (7′′-C), 121.7 (4′′-C), 122.4 (5′′-C), 123.6 (6′′-C), 133.2 (2′′-C); ESI-(−)-HR-MS: obs. m/z 390.1927 [M − H]−, calc. m/z 390.1922 for C21H28NO6, Δ +1.3 ppm.
Synthesis of a diastereoisomeric mixture of (R)-icas#6.1 (7) and (S)-icas#6.2 (14).
A solution of 34 μg synthetic icas#2 (6) (87 nmol) in 1 ml methanol was treated with 1 mg NaBH4 (26 mmol, 300 equivalents) and stirred for 30 min until mass spectrometry indicated the complete consumption of the starting material. The reaction was quenched by addition of 10 μl formic acid, stirred for 30 min, and the solution was concentrated to dryness under reduced pressure. The reaction products were purified by reverse phase solid phase extraction on the 500 mg C18ec phase (Chromabond, Macherey-Nagel) using a stepwise gradient of methanol in water as the eluent. The 60% fraction containing the products, an equimolar mixture of diastereoisomeric (R)-icas#6.1 (7) and (S)-icas#6.2 (14), was concentrated under reduced pressure, dried under high vacuum, and analyzed by 1H NMR spectroscopy (Fig. S6†).
Retention assay to evaluate nematode behavioural response
Nematode preference for environments conditioned with known amounts of indole ascarosides was measured using a modified retention assay.19 On a 6 cm Petri dish filled with 6 ml peptone-free NGM agar, circular scoring regions of 9 mm diameter were marked. Next, 1 μl of 10% aqueous methanol (as negative control) or indole ascaroside solutions, e.g. icas#3 (3), icas#9 (4), icas#2 (6), (S)-icas#6.2 (7), (R)-icas#6.1 (14), or their mixtures in 10% aqueous methanol were placed in the centre of the scoring areas onto the agar and left to dry for 5 minutes. Young adult nematodes from non-starved and non-crowded 6 cm NGM plates seeded with E. coli OP50 were transferred to peptone-free NGM agar without any food for ca. 15 min before being used for the assay to minimize the amount of concomitant bacteria. Individual worms were placed into the centre of the conditioned scoring region and the time required for the nematode to leave the scoring region was measured. Nematodes were defined to have left the scoring area when no part of the nematode was still within the circular boundary. A total number of 10 worms per condition were analysed. A one-way ANOVA with Dunett's post-test was performed between subjects using the SPSS software to evaluate the effect of indole ascarosides on mean times nematode spent in scoring regions.
Four spot attraction assay to evaluate nematode behavioural response
Nematode attraction to known amounts of indole ascarosides was measured using a modified 4 spot attraction assay.19 On a 6 cm Petri dish filled with 6 ml peptone-free NGM agar four circular scoring regions of 9 mm diameter were marked in a square of 3 × 3 cm. Next, 2 μl of 1 M sodium azide solution was placed in the centre of all four scoring areas and left to dry for 5 minutes. Next, 1 μl of 10% aqueous methanol (as negative control) or indole ascaroside test solutions (1 nM icas#2 (6), 1 nM (S)-icas#6.2 (7), or a blend of 1 nM icas#2 (6) and 1 nM (S)-icas#6.2 (7)) in 10% aqueous methanol were placed in the centre of the two opposing scoring areas onto the agar and left to dry for 5 minutes. Young adult male nematodes of C. briggsae strain AF16 from non-starved and non-crowded 6 cm NGM plates seeded with E. coli OP50 were transferred to peptone-free NGM agar without any food for ca. 15 min before being used for the assay to minimize the amount of concomitant bacteria. A total number of 50 worms were placed into the centre of the plate between the 4 scoring regions. After 30 minutes immobilized nematodes in the scoring regions were counted and the attraction index was calculated according to: CI = (n[icas] − n[control])/(n[icas] + n[control]). A total number of 4 × 50 worms per condition were analyzed and the experiments were performed in duplicate on two separate days. Student's t test with Welch's correction was performed using the SPSS software to evaluate the attraction of nematodes to indole ascarosides in the 4 spot attraction assays.
Author information
CD performed nematode cultivation, natural product isolation, and chemical analysis. FD performed total synthesis. SHvR designed the research, developed the MS screen, and performed the bioassays. SHvR, CD, and FD wrote the manuscript.
Conflict of interests
The authors declare no competing financial interests.
Acknowledgements
Financial support by the Max Planck Society and the Jena School for Microbial Communication (JSMC) is gratefully acknowledged. Authentic standards of icas#3 and icas#9 were kindly provided by F. C. Schroeder. All nematode strains were provided by the Caenorhabditis Genetics Center, which is funded by the NIH Office of Research Infrastructure Programs (P40 OD010440).
Notes and references
- S. H. von Reuss and F. C. Schroeder, Nat. Prod. Rep., 2015, 32, 994–1006 RSC.
- R. A. Butcher, M. Fujita, F. C. Schroeder and J. Clardy, Nat. Chem. Biol., 2007, 3, 420–422 CrossRef CAS PubMed.
- S. A. Diaz, V. Brunet, G. C. Lloyd-Jones, W. Spinner, B. Wharam and M. Viney, BMC Evol. Biol., 2014, 14, 46 CrossRef PubMed.
- J. Srinivasan, F. Kaplan, R. Ajredini, C. Zachariah, H. T. Alborn, P. E. A. Teal, R. U. Malik, A. S. Edison, P. W. Sternberg and F. C. Schroeder, Nature, 2008, 454, 1115–1118 CrossRef CAS PubMed.
- C. Pungaliya, J. Srinivasan, B. W. Fox, R. U. Malik, A. H. Ludewig, P. W. Sternberg and F. C. Schroeder, Proc. Natl. Acad. Sci. U. S. A., 2009, 106, 7708–7713 CrossRef CAS PubMed.
- S. H. von Reuss, N. Bose, J. Srinivasan, J. J. Yim, J. C. Judkins, P. W. Sternberg and F. C. Schroeder, J. Am. Chem. Soc., 2012, 134, 1817–1824 CrossRef CAS PubMed.
- X. Zhang, L. Feng, S. Chinta, P. Singh, Y. Wang, J. K. Nunnery and R. A. Butcher, Proc. Natl. Acad. Sci. U. S. A., 2015, 112, 3955–3960 CrossRef CAS PubMed.
- J. Srinivasan, S. H. von Reuss, N. Bose, A. Zaslaver, P. Mahanti, M. C. Ho, O. G. O'Doherty, A. S. Edison, P. W. Sternberg and F. C. Schroeder, PLoS Biol., 2012, 10, e1001237 CAS.
- R. A. Butcher, J. R. Ragains and J. Clardy, Org. Lett., 2009, 11, 3100–3103 CrossRef CAS PubMed.
- A. Choe, S. H. von Reuss, D. Kogan, R. B. Gasser, E. G. Platzer, F. C. Schroeder and P. W. Sternberg, Curr. Biol., 2012, 22, 772 CrossRef CAS PubMed.
- K. C. Kiontke, M. A. Félix, M. Ailion, M. V. Rockman, C. Braendle, J. B. Pénigault and D. H. A. Fitch, BMC Evol. Biol., 2011, 11, 339 CrossRef CAS PubMed.
- M.-A. Félix, C. Braendle and A. D. Cutter, PLoS One, 2014, 9, e94723 Search PubMed.
- M.-A. Félix and F. Duveau, BMC Biol., 2012, 10, 59 CrossRef PubMed.
- M.-A. Félix, R. Jovelin, C. Ferrari, S. Han, Y. R. Cho, E. C. Andersen, A. D. Cutter and C. Braendle, BMC Evol. Biol., 2013, 13, 1 CrossRef PubMed.
- C. Petersen, M. Saebelfeld, C. Barbosa, B. Pees, R. J. Hermann, R. Schalkowski, E. A. Strathmann, P. Dirksen and H. Schulenburg, Ecol. Evol., 2015, 5, 3250–3263 CrossRef PubMed.
- A. D. Cutter, M.-A. Félix, A. Barrière and D. Charlesworth, Genetics, 2006, 173, 2021–2031 CrossRef CAS PubMed.
- E. S. Dolgin, M.-A. Félix and A. D. Cutter, Heredity, 2008, 100, 304–315 CrossRef CAS PubMed.
- K. A. Hollister, E. S. Conner, X. X. Zhang, M. Spell, G. M. Bernard, P. Patel, A. C. G. V. de Carvalho, R. A. Butcher and J. R. Ragains, Bioorg. Med. Chem., 2013, 21, 5754–5769 CrossRef CAS PubMed.
- J. M. Simon and P. W. Sternberg, Proc. Natl. Acad. Sci. U. S. A., 2002, 99, 1598–1603 CrossRef CAS PubMed.
-
T. Stiernagle, Maintenance of C. elegans, in WormBook, ed. The C. elegans Research Community, 2006 Search PubMed.
Footnotes |
† Electronic supplementary information (ESI) available: NMR spectra of natural and synthetic compounds, details of nematode retention assays. See DOI: 10.1039/c6ob01230b |
‡ These authors contributed equally. |
§ For nomenclature of ascarosides see: http://www.smid-db.org. |
|
This journal is © The Royal Society of Chemistry 2016 |
Click here to see how this site uses Cookies. View our privacy policy here.