DOI:
10.1039/C6OB00108D
(Communication)
Org. Biomol. Chem., 2016,
14, 5525-5528
3-Component synthesis of α-substituted sulfonamides via Brønsted acid-catalyzed C(sp3)–H bond functionalization of 2-alkylazaarenes†
Received
14th January 2016
, Accepted 9th February 2016
First published on 9th February 2016
Abstract
A Brønsted acid-catalyzed addition of 2-alkylazaarenes to in situ generated N-sulfonylimines through selective C(sp3)–H bond functionalization has been developed. This protocol provides an atom- and step-economic approach to α-substituted sulfonamides.
The selective functionalization of C–H bonds plays a key role in the development of more efficient and sustainable bond forming reactions.1 In the past two decades metal-catalyzed activations of C–H bonds have emerged as a valuable and efficient tool for the atom-economic2 construction of carbon–carbon and carbon–heteroatom bonds.3 In this context, the metal-catalyzed addition of C–H bonds to imines provides a powerful method for the synthesis of α-branched amines, a prevalent structural motif in drugs and natural products. So far transition-metal catalyzed addition reactions of aromatic and vinylic C(sp2)–H bonds to imines4,5 as well as transition metal- and Lewis acid-catalyzed benzylic additions of azaarenes via activation of C(sp3)–H bonds6 have been reported (Scheme 1). Although concise and atom-economic, the overall synthetic utility of these methods is hampered by the additional step required for the preparation of the imine. Based on our continued interest in acyl- and sulfonylimine-based multicomponent reactions,7 we considered a possible in situ generation of the reactive imine in such C–H functionalization reactions.8 This approach would combine all the advantages of C–H functionalization reactions and multicomponent synthesis9 and would lead to a more atom- as well as step-economic10 synthesis of α-branched sulfonamides. Herein we report, to our knowledge, the first example of an addition of a C(sp3)–H bond to an in situ generated N-sulfonylimine via Brønsted acid catalyzed C–H functionalization.
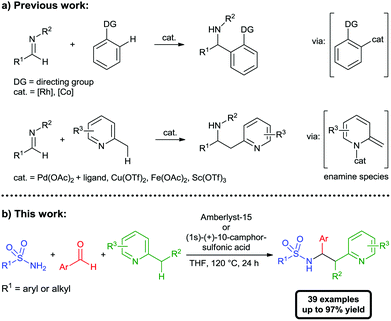 |
| Scheme 1 Direct C–H bond functionalization: general view of previous work and this report. | |
As starting point to investigate a possible combination of C–H functionalization and in situ imine formation we chose reactions with 2-alkylazaarenes. We envisioned, that the reported Lewis or Brønsted acids11 used for functionalization of the benzylic C(sp3)–H bond could simultaneously catalyze the generation of an reactive imine species via condensation of an amide and an aldehyde. To identify an appropriate catalyst and to optimize the reaction conditions, we chose the reaction between p-toluenesulfonamide (1a), benzaldehyde (2a) and 2,6-dimethylpyridine (3a) (Table 1).
Table 1 3-Component synthesis of α-substituted sulfonamides via Brønsted acid-catalyzed C(sp3)–H bond functionalization of 2-alkylazaarenes: influence of reaction parameters
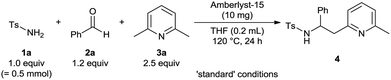
|
Entry |
Variation from the ‘standard’ conditions |
Yielda (%) |
Yields of isolated products. Reactions were performed on a 0.5 mmol scale without exclusion of air or moisture.
No asymmetric induction was observed.
|
1 |
None |
80
|
2 |
(1s)-(+)-10-Camphorsulfonic acid (5 mol%), instead of Amberlyst-15 |
75b |
3 |
2,6-Dinitrobenzenesulfonic acid (5 mol%), instead of Amberlyst-15 |
69 |
4 |
Mg(OTf)2 (5 mol%), instead of Amberlyst-15 |
68 |
5 |
Zn(OTf)2 (5 mol%), instead of Amberlyst-15 |
75 |
6 |
Yb(OTf)3 (5 mol%), instead of Amberlyst-15 |
78 |
7 |
No catalyst |
20–30 |
8 |
0.3 mL THF, instead of 0.2 mL |
66 |
9 |
0.5 mL THF, instead of 0.2 mL |
65 |
10 |
100 °C, instead of 120 °C |
42–77 |
11 |
1.5 equiv. 2,6-lutidine, instead of 2.5 equiv. |
39 |
12 |
3.5 equiv. 2,6-lutidine, instead of 2.5 equiv. |
79 |
To our delight, several Brønsted as well as Lewis acids could catalyze this reaction and provided the desired product 4a in 69–80% yield (entries 1–6). Best results were obtained with Amberlyst-15, a commercial available heterogeneous sulfonic acid catalyst12 (entry 1). Although (1s)-(+)-10-camphorsulfonic acid (entry 2) as well as several metal triflates, for example Zn(OTf)2 or Yb(OTf)3 (entries 5 and 6), displayed almost identical catalytic activities, we chose Amberlyst-15 due to its additional advantages such as low cost, simple catalyst separation by filtration and potential catalyst recycling. Without catalyst product 4a was obtained in 20–30% yield (entry 7). Generation of the enamine species by C–H cleavage of the benzylic C–H bond of 2-substituted alkylazaarenes at high temperatures is known from literature.6e Hence, it is expected that the Brønsted acid is mainly required for the in situ generation of the sulfonylimine. Initial studies revealed that THF constituted the best solvent for this transformation. Performing the reaction at high temperatures (100–120 °C), using high concentrations (2.5 M) and at least 2.5 equivalents of the 2-alkylazaarene proved to be crucial for obtaining high yields (compare entries 1 and 8–12). A reaction temperature of 120 °C provided the highest and most reproducible yields.
With the optimized conditions at hand, we investigated the scope and limitations of our method. The 3-component reaction with various electron-rich and -poor as well as halogenated aryl sulfonamides or the heterocyclic thiophene-2-sulfonamide proceeded smoothly and afforded the corresponding products 4a–4j in good yields (Table 2). Moreover, alkyl sulfonamides can be used as amide component, furnishing the desired α-branched sulfonamides in 54 and 70% yields (4k and 4l). In some cases (4c, 4f, 4k) better yields were obtained with (1s)-(+)-10-camphorsulfonic acid as catalyst. This might be due to the fact, that the reaction temperature of 120 °C corresponds to the maximum operating temperature for Amberlyst-15 and catalyst decomposition might occur.12,13
Table 2 Variation of sulfonamidesa
The reported yields are those of the isolated products. Reactions were performed on a 0.5 mmol scale without exclusion of air or moisture. Reaction times were not optimized.
(1s)-(+)-10-Camphorsulfonic acid (5 mol%), instead of Amberlyst-15.
|
|
Next we investigated the scope of the reaction in terms of the aldehyde component. As shown in Table 3, a broad range of aryl aldehydes are suitable substrates for the 3-component synthesis. Substituents at various positions on the aryl ring were tolerated. Reactions of electron-donating or -withdrawing as well as halogen substituted aryl aldehydes gave the corresponding products 4m–4ac in good to high yields. Heterocyclic aldehydes displayed a similar reactivity and the α-substituted sulfonamides 4ad–4af were isolated in 46–67% yields. As shown before, in some cases the use of 5 mol% (1s)-(+)-10-camphorsulfonic acid as catalyst provided approximately 20% higher yields (4m, 4p, 4z, 4aa and 4ae). Reactions with other aldehyde components, such as alkyl aldehydes or glyoxalates were unsuccessful.
Table 3 Variation of aryl aldehydesa
The reported yields are those of the isolated products. Reactions were performed on a 0.5 mmol scale without exclusion of air or moisture. Reaction times were not optimized.
(1s)-(+)-10-Camphorsulfonic acid (5 mol%), instead of Amberlyst-15.
|
|
Subsequently, we examined reactions with various 2-alkylazaarenes (Table 4). Unfortunately, the substrate scope in terms of the 2-alkylazaarenes component is not as broad as that for the other two reactants. Compared to 2,6-lutidine (4a), reactions of other substituted pyridine or quinoline derivatives, such as 2,3-lutidine or 8-hydroxyquinoline, gave the desired α-branched sulfonamides in only moderate yields (4ag, 4ai–4al). Only in the case of 2-picoline a substantial increase in yield could be achieved with (1s)-(+)-10-camphorsulfonic acid as catalyst. Interestingly, 4-methylpyridine did not react at all under our reaction conditions. Contrary to literature reports on Lewis acid catalyzed reactions6b,c we did not observe γ-functionalization. 5-Membered 2-alkyl-substituted nitrogen heterocycles, such as 2-methylbenzo[d]oxazole, or various diazines did not react under the standard reaction conditions. Only in the case of quinoxaline the desired product was isolated in low yield (4al). 2-Ethylpyridine proved to be an excellent substrate for this 3-component synthesis, furnishing the α-substituted amine 4an in 97% yield as a 2.4
:
1 mixture of diastereomers.
Table 4 Variation of 2-alkylazaarenesa
The reported yields are those of the isolated products. Reactions were performed on a 0.5 mmol scale without exclusion of air or moisture. Reaction times were not optimized.
(1s)-(+)-10-Camphorsulfonic acid (5 mol%), instead of Amberlyst-15.
|
|
Conclusions
In summary, we have developed a Brønsted acid-catalyzed 3-component synthesis of α-substituted sulfonamides from 2-aklyazaarenes, aryl aldehydes and sulfonamides. This method is based on the combination of an in situ imine generation and an acid-catalyzed selective C(sp3)–H bond activation. The reaction has a broad scope and is simple to perform. With water as only byproduct this protocol provides an atom- and step-economic, sustainable approach to various heterocyclic α-substituted sulfonamides. In addition, this method represents an example for the successful merger of two synthetically very useful transformations, the selective functionalization of C–H bonds and multicomponent reactions. Further extensions of this concept to various metal-catalyzed C–H activation reactions are currently investigated in our laboratory.
Acknowledgements
This work was financially supported by the Fonds der Chemischen Industrie (Liebig fellowship to G. Manolikakes) and Stiftung Polytechnische Gesellschaft Frankfurt am Main (Ph.D. fellowship to T. Beisel). We would like to thank Professor Michael Göbel (Goethe-University Frankfurt) for his support, and BASF SE, Evonik Industries AG, and Rockwood Lithium GmbH for generous donations of chemicals.
Notes and references
-
(a) J. Yamaguchi, K. Itami and A. D. Yamaguchi, Angew. Chem., Int. Ed., 2012, 51, 8960 CrossRef CAS PubMed;
(b) W. R. Gutekunst and P. S. Baran, Chem. Soc. Rev., 2011, 40, 1976 RSC;
(c) L. Ackermann, Chem. Rev., 2011, 111, 1315 CrossRef CAS PubMed;
(d) J. Wencel-Delord, T. Dröge, F. Liu and F. Glorius, Chem. Soc. Rev., 2011, 40, 4740 RSC;
(e) R. Jazzar, J. Hitce, A. Renaudat, J. Sofack-Kreutzer and O. Baudoin, Chem. – Eur. J., 2010, 16, 2654 CrossRef CAS PubMed;
(f) R. H. Crabtree, Chem. Rev., 2010, 110, 575 CrossRef CAS PubMed;
(g) R. G. Bergman, Nature, 2007, 446, 391 CrossRef CAS PubMed;
(h) K. Godula and D. Sames, Science, 2006, 312, 67 CrossRef CAS PubMed;
(i)
G. Dyker, Handbook of C-H Transformations, Wiley-VCH, Weinheim, 2005 Search PubMed;
(j)
K. I. Goldberga and A. S. Goldman, Activation and Functionalization of C-H Bonds (ACS Symposium), Oxford University Press, Oxford, 2004 Search PubMed;
(k) F. Kakiuchi and N. Chatani, Adv. Synth. Catal., 2003, 345, 1077 CrossRef CAS;
(l) J. A. Labinger and J. E. Bercaw, Nature, 2002, 417, 507 CrossRef CAS PubMed;
(m) C. Jia, T. Kitamura and Y. Fujiwara, Acc. Chem. Res., 2001, 34, 633 CrossRef CAS PubMed;
(n) G. Dyker, Angew. Chem., Int. Ed., 1999, 38, 1698 CrossRef.
-
(a) B. M. Trost, Acc. Chem. Res., 2002, 35, 695 CrossRef CAS PubMed;
(b) B. M. Trost, Science, 1991, 254, 1471 CAS.
-
(a) L. Yang and H. Huang, Chem. Rev., 2015, 115, 3468 CrossRef CAS PubMed;
(b) K. Gao and N. Yoshikai, Acc. Chem. Res., 2014, 47, 1208 CrossRef CAS PubMed;
(c) G. Yan, X. Wu and M. Yang, Org. Biomol. Chem., 2013, 11, 5558 RSC;
(d) P. B. Arockiam, C. Bruneau and P. H. Dixneuf, Chem. Rev., 2012, 112, 5879 CrossRef CAS PubMed;
(e) C.-L. Sun, B.-J. Li and Z.-J. Shi, Chem. Rev., 2011, 111, 1293 CrossRef CAS PubMed;
(f) C. S. Yeung and V. M. Dong, Chem. Rev., 2011, 111, 1215 CrossRef CAS PubMed;
(g) T. W. Lyons and M. S. Sanford, Chem. Rev., 2010, 110, 1147 CrossRef CAS PubMed;
(h) D. A. Colby, R. G. Bergman and J. A. Ellman, Chem. Rev., 2010, 110, 624 CrossRef CAS PubMed;
(i) P. Thansandote and M. Lautens, Chem. – Eur. J., 2009, 15, 5874 CrossRef CAS PubMed;
(j) D. Alberico, M. S. Scott and M. Lautens, Chem. Rev., 2007, 107, 174 CrossRef CAS PubMed;
(k) V. Ritleng, C. Sirlin and M. Pfeffer, Chem. Rev., 2002, 102, 1731 CrossRef CAS PubMed;
(l) Y. Guari, S. Sabo-Etienne and B. Chaudret, Eur. J. Inorg. Chem., 1999, 1047 CrossRef CAS.
- For the rhodium-catalyzed intermolecular addition of aromatic and vinylic C(sp2)–H bonds to imines, see:
(a) A. Wangweerawong, R. G. Bergman and J. A. Ellman, J. Am. Chem. Soc., 2014, 136, 8520 CrossRef CAS PubMed;
(b) K. Parthasarathy, A. R. Azcargorta, Y. Cheng and C. Bolm, Org. Lett., 2014, 16, 2538 CrossRef CAS PubMed;
(c) B. Zhou, Y. Yang, S. Lin and Y. Li, Adv. Synth. Catal., 2013, 355, 360 CAS;
(d) M. E. Tauchert, C. D. Incarvito, A. L. Rheingold, R. G. Bergman and J. A. Ellman, J. Am. Chem. Soc., 2012, 134, 1482 CrossRef CAS PubMed;
(e) Y. Li, X.-S. Zhang, H. Li, W.-H. Wang, K. Chen, B.-J. Li and Z.-J. Shi, Chem. Sci., 2012, 3, 1634 RSC;
(f) Y. Li, X.-S. Zhang, Q.-L. Zhu and Z.-J. Shi, Org. Lett., 2012, 14, 4498 CrossRef CAS PubMed;
(g) K. D. Hesp, R. G. Bergman and J. A. Ellman, Org. Lett., 2012, 14, 2304 CrossRef CAS PubMed;
(h) Y. Li, B.-J. Li, W.-H. Wang, W.-P. Huang, X.-S. Zhang, K. Chen and Z.-J. Shi, Angew. Chem., Int. Ed., 2011, 50, 2115 CrossRef CAS PubMed;
(i) A. S. Tsai, M. E. Tauchert, R. G. Bergman and J. A. Ellman, J. Am. Chem. Soc., 2011, 133, 1248 CrossRef CAS PubMed.
- For cobalt-catalyzed intermolecular addition of aromatic and vinylic C(sp2)–H bonds to imines, see:
(a) T. Yoshino, H. Ikemoto, S. Matsunaga and M. Kanai, Chem. – Eur. J., 2013, 19, 9142 CrossRef CAS PubMed;
(b) T. Yoshino, H. Ikemoto, S. Matsunaga and M. Kanai, Angew. Chem., Int. Ed., 2013, 52, 2207 CrossRef CAS PubMed;
(c) K. Gao and N. Yoshikai, Chem. Commun., 2012, 48, 4305 RSC.
-
(a) L. S. Rocha and I. P. Beletskaya, Russ. Chem. Bull., Int. Ed., 2014, 63, 2686 CrossRef;
(b) D. Best, S. Kujawa and H. Wai Lam, J. Am. Chem. Soc., 2012, 134, 18193 CrossRef CAS PubMed;
(c) H. Komai, T. Yoshino, S. Matsunaga and M. Kanai, Synthesis, 2012, 2185 CAS;
(d) M. Rueping and N. Tolstoluzhsky, Org. Lett., 2011, 13, 1095 CrossRef CAS PubMed;
(e) Y. Yan, K. Xu, Y. Fang and Z. Wang, J. Org. Chem., 2011, 76, 6849 CrossRef CAS PubMed;
(f) B. Qian, P. Xie, Y. Xie and H. Huang, Org. Lett., 2011, 13, 2580 CrossRef CAS PubMed;
(g) B. Qian, S. Guo, C. Xia and H. Huang, Adv. Synth. Catal., 2010, 352, 3195 CrossRef CAS;
(h) B. Qian, S. Guo, J. Shao, Q. Zhu, L. Yang, C. Xia and H. Huang, J. Am. Chem. Soc., 2010, 132, 3650 CrossRef CAS PubMed.
-
(a) T. Beisel and G. Manolikakes, Synthesis, 2015, A–H Search PubMed;
(b) T. Beisel and G. Manolikakes, Org. Lett., 2015, 17, 3162 CrossRef CAS PubMed;
(c) T. Beisel and G. Manolikakes, Org. Lett., 2013, 15, 6046 CrossRef CAS PubMed;
(d) J. Halli and G. Manolikakes, Eur. J. Org. Chem., 2013, 7471 CrossRef CAS;
(e) A. E. Schneider and G. Manolikakes, Synlett, 2013, 2057 CAS;
(f) A. E. Schneider, T. Beisel, A. Shemet and G. Manolikakes, Org. Biomol. Chem., 2014, 12, 2356 RSC.
- A different approach for the oxidative in situ imine formation from the corresponding amino acid has been reported by Huang, see: Z.-Q. Zhu, P. Bai and Z.-Z. Huang, Org. Lett., 2014, 16, 4881 CrossRef CAS PubMed.
-
(a)
J. Zhu and H. Bienaymé, Multicomponent Reactions, Wiley-VCH, Weinheim, 2005 Search PubMed;
(b) H. Bienaymé, C. Hulme, G. Oddon and P. Schmidt, Chem. – Eur. J., 2000, 6, 3321 CrossRef;
(c) R. W. Armstrong, A. P. Combs, P. A. Tempest, S. D. Brown and T. A. Keating, Acc. Chem. Res., 1996, 29, 123 CrossRef CAS.
- P. A. Wender, V. A. Verma, T. J. Paxton and T. H. Pillow, Acc. Chem. Res., 2008, 41, 40 CrossRef CAS PubMed.
- The use of Brønsted acid catalysts for the benzylic addition of 2-azaarenes has been reported for reactions with carbonyl and nitroso compounds but not with imines, see:
(a) X. Gao, F. Zhang, G. Deng and L. Yang, Org. Lett., 2014, 16, 3664 CrossRef CAS PubMed;
(b) A. I. Lansakara, D. P. Farrell and F. C. Pigge, Org. Biomol. Chem., 2014, 12, 1090 RSC;
(c) J.-J. Jin, D.-C. Wang, H.-Y. Niu, S. Wu, G.-R. Qu, Z.-B. Zhang and H.-M. Guo, Tetrahedron, 2013, 69, 6579 CrossRef CAS;
(d) R. Niu, J. Xiao, T. Liang and X. Li, Org. Lett., 2012, 14, 676 CrossRef CAS PubMed;
(e) F.-F. Wang, C.-P. Luo, Y. Wang, G. Deng and L. Yang, Org. Biomol. Chem., 2012, 10, 8605 RSC.
- R. Pal, T. Sarkar and S. Khasnobis, ARKIVOC, 2012, I, 570 Search PubMed.
- Due to this fact all attempts to recycle the Amberlyst-15 catalyst were unsuccessful. A sharp decrease in yield was observed even in the 2nd cycle.
Footnotes |
† Electronic supplementary information (ESI) available: Full experimental procedures and characterization data (including 1H and 13C NMR spectra). See DOI: 10.1039/c6ob00108d |
‡ These authors contributed equally. |
|
This journal is © The Royal Society of Chemistry 2016 |