DOI:
10.1039/C6MD00042H
(Research Article)
Med. Chem. Commun., 2016,
7, 1209-1216
Design and synthesis of fused soluble epoxide hydrolase/peroxisome proliferator-activated receptor modulators†
Received
19th January 2016
, Accepted 10th April 2016
First published on 22nd April 2016
Abstract
Metabolic syndrome (MetS) is a widespread, complex disease cluster which consists of hypertension, atherosclerosis, dyslipidaemia and type II diabetes. The treatment of MetS requires multiple pharmaceutical agents leading to complex polypharmacy. Multi-target compounds might reduce the number of required drugs in MetS patients. In this study we fused three different pharmacophores of soluble epoxide hydrolase (sEH) inhibitors and peroxisome proliferator-activated receptor (PPAR) agonists. The most promising fused scaffold exhibits multi-target activity and represents a valuable starting point for design and evaluation of fused sEH/PPAR modulators.
Introduction
Metabolic syndrome (MetS) is a complex disease cluster with high prevalence in the western society.1 Central obesity is regarded as the most relevant risk factor which is accompanied by dyslipidaemia, type II diabetes, and hypertension.2 This so-called “deadly quartet” is accompanied by diverse complications leading to treatment with multiple pharmacological agents.3 Complex treatment regimen, also referred as polypharmacy, cause adverse effects, drug–drug interactions, and ultimately, failure of the therapy.4 In this context, multi-target approaches offer a promising strategy to deliver safe and efficient drugs for the treatment of complex disease clusters.5 Simultaneous modulation of soluble epoxide hydrolase (sEH) and peroxisome proliferator-activated receptor γ (PPARγ) was shown to exhibit synergistic effects in spontaneously hypertensive obese (SHROB) rats.6 In this setting, a combination of the sEH inhibitor tAUCB and the PPARγ agonist rosiglitazone efficiently restored insulin sensitivity, lowered blood pressure, and protected the animals from nephropathy. Hypothetically, beneficial effects of dual sEH/PPARγ modulation might be enhanced by effective control of plasma lipids, which can be achieved by activation of PPARα.7 Thus, a simultaneous modulation of sEH, PPARγ, and PPARα might be a valuable profile of a multi-target agent for effective and safe MetS therapy.
PPARs are ligand-activated transcription factors which are involved in glucose and lipid homeostasis. PPARγ is targeted by thiazolidinediones (TZDs), also known as insulin sensitizers. Fibrates, which are clinically used for treatment of dyslipidemia are addressing all PPAR subtypes with slight preference for PPARα.8 The role of sEH in cardiovascular disease associated with MetS is well known and has been excessively reviewed.9 sEH converts epoxyeicosatrienoic acids (EETs) to the corresponding dihydroxyeicosatrienoic acids (DHETs) and sEH inhibition increases levels of EETs while decreasing levels of DHETs. The resulting increase in the EET/DHET ratio has multiple beneficial effects on the cardiovascular system. EETs are described as agonists of PPARα and PPARγ10 which subsequently promotes the expression of sEH.11 Thus, there is a substantial crosstalk between sEH and PPAR activity supporting the assumption of synergistic targeting of the sEH/PPAR axis.12,13
We have previously reported that it is possible to combine the pharmacophores of an sEH inhibitor and PPAR agonist using a combinatorial approach.14 In the present study we have explored three different scaffolds on their potential for dual sEH/PPAR modulation.
Results and discussion
Our first attempt to obtain dual sEH/PPAR modulators was the incorporation of the simplified sEH pharmacophore N-cyclohexyl-N′-(iodophenyl)urea (CIU)15 into bezafibrate (Fig. 1). Bezafibrate is a pan-PPAR agonist exhibiting EC50 values in the micromolar range.16 We decided to remove one of the methyl groups in α-position of the carboxylic acid and replaced the amide moiety by a urea residue. The resulting starting point 3c of our SAR study comprises a linker length similar to bezafibrate while 3d has an additional methylene unit in the linker region.
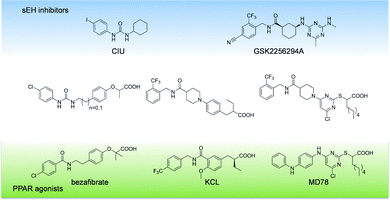 |
| Fig. 1 Scaffolds used for design of fused dual sEH/PPAR modulators. | |
The synthesis of compounds 3a–d was accomplished as shown in Scheme 1. Initially, ethyl 2-bromopropanoate was coupled with the corresponding phenol under Williamson conditions. Then, the nitrile residue was reduced with palladium on carbon as catalyst. The resulting primary amines 2a and 2b were coupled to 4-chlorophenyl isocyanate to yield ureas 3a and 3b.
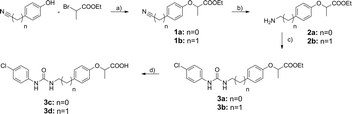 |
| Scheme 1 a) K2CO3, acetone (abs.), reflux, 12 h, b) Pd/C, AcOH, EtOH (abs.), H2, 12 h, c) 4-chlorophenyl isocyanate, DIPEA, DCM (abs.), Ar, 12 h, d) KOH, THF/H2O/MeOH (1/2/1), 90 °C, μw, 30 min. | |
Compounds 3a–d were evaluated in a fluorescence-based assay with recombinant sEH and all are able to inhibit sEH activity with submicromolar potency. Esters 3a and 3b were almost one order of magnitude more potent than the corresponding acids due to the hydrophobic nature of the sEH binding site. Furthermore, the ethyl linker was slightly preferred. Unfortunately, none of the compounds was able to activate any of the PPAR subtypes at a concentration of 30 μM, which forced us to pursue a different scaffold.
We decided to exchange the urea by an amide residue as epoxide mimetic for sEH targeting and chose the N-benzyl amide of isonipecotic acid moiety present in the clinical candidate GSK2256294A that targets sEH.17N-Benzylamides are also known as privileged structures in the design of PPAR agonists with different subtype preferences, represented by KCL.18,19 The synthesis of the chimera compounds 5a–d was accomplished by isobutyl chloroformate (IBCF) mediated amide coupling of 2-trifluoromethylbenzylamine and 1-(4-formylphenyl)piperidine-4-carboxylic acid. The resulting ethyl cinnamate derivatives 5a and 5d were obtained under Wittig–Horner conditions followed by reduction with magnesium in methanol and saponification under microwave conditions as described before (Scheme 2).
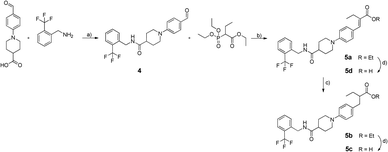 |
| Scheme 2 a) Isobutyl chloroformate (IBCF), trimethylamine (TEA), dichloromethane (DCM) (abs.), Ar, 12 h, b) NaH, tetrahydrofuran (THF) (abs.), 0 °C, 2 h, c) Mg, MeOH (abs.), Ar, 12 h, d) KOH, THF/H2O/MeOH (1/2/1), 90 °C, μw, 30 min. | |
All derivatives 5a–d exhibited sEH nanomolar inhibition. Since acids and the corresponding esters showed less differences in activity, we assumed that the scaffold was very well-tolerated by sEH. However, again no activity on PPARs could be determined. Encouraged by the robust sEH inhibitory activity of N-benzyl amides we fused this element with the 4-chloro-2-thio-pyrimidine scaffold which has been described in various studies as a privileged structure for PPAR activation.20 The synthesis of compounds 8c and 8d is shown in Scheme 3. 2-((4,6-Dichloropyrimidine-2-yl)thio)octanoate was prepared as described previously.21 Boc-protected γ-amino acids were coupled to 2-trifluoromethylbenzylamine mediated by IBCF, followed by Boc cleavage. Scaffold fusion was accomplished under basic conditions in acetonitrile, followed by saponification.
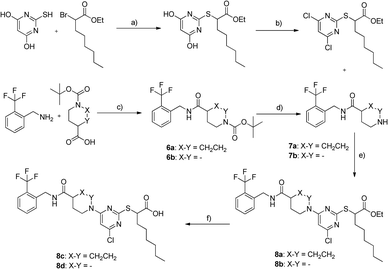 |
| Scheme 3 a) TEA, dimethylformamide (DMF) (abs.), 90 °C, 3 h, b) POCl3, N,N-diethylaniline, 90 °C, 6 h, c) IBCF, TEA, DCM (abs.), Ar, 12 h, d) trifluoroacetic acid (TFA), DCM (abs.), rt, 30 min, e) TEA, MeCN (abs.), rt, 12 h, f) LiOH, H2O/THF, 45 °C, 24 h. | |
Compounds 8a–d showed sEH inhibitory potency in a low micromolar to submicromolar range. As observed for other scaffolds, acidic derivatives suffered a 4–6-fold loss in potency. Rigidified derivatives 8a and 8c were not active on PPARs, while compound 8d partially activated PPARα and PPARγ and finally represented the desired triple active agent.
In order to examine PPAR activation under native conditions, mouse fibroblasts were incubated with 8d and a dose-dependent adipocyte differentiation could be observed (Fig. 2). Notably the absolute extend of adipocyte differentiation was significantly lower compared to the full agonist rosiglitazone, confirming the favourable partial PPAR agonistic properties of 8d.
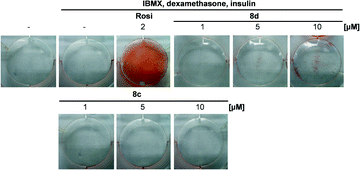 |
| Fig. 2 Oil Red O staining of differentiated murine 3T3-L1 fibroblasts. Staining was performed after 14 days of differentiation. One representative experiment out of 4 is shown. Rosi, rosiglitazone; CIU, sEH inhibitor N-cyclohexyl-N′-iodophenyl urea. | |
An established pharmacophore of dual PPAR agonists consists of an acidic head group, a central aromatic ring, and an additional lipophilic substituent connected to the aromatic ring by a flexible linker.22 It is well-established that PPAR ligands should be able to adopt a conformation wrapped around helix 3.23 We investigated the differences between 8c and 8d considering the conformational space. While the flexible linker of 8d allows such conformation, it is almost impossible for the rigid derivative 8c (Fig. 3). With its combined potency on sEH and PPARs, 8d represents a very promising lead to explore the potential of a combined sEH and PPAR modulation as a novel concept to treat MetS and further studies are warranted (Table 1).
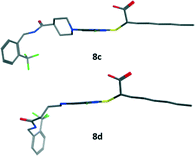 |
| Fig. 3 Low-energy conformation of compounds 8c and 8d, calculated by quantum mechanics energy minimization software implemented in MOE2015.10 (Chemical Computing Group, Montreal, Canada). | |
Table 1
In vitro activity values of dual sEH/PPAR modulators
Compd. |
IC50 sEH [μM] |
EC50 PPARα [μM] (Emax – %) |
EC50 PPARδ [μM] (Emax – %) |
EC50 PPARγ [μM] (Emax – %) |
Bezafibrate |
ia. |
7.1 ± 1.4 μM (62%) |
22.6 ± 1.9 μM (44%) |
15.4 ± 0.2 μM (109%) |
CIU |
0.14 ± 0.02 |
ia. |
ia. |
@10 μM (22%) |
GSK2256294A |
0.11 ± 0.009 |
ia. |
ia. |
ia. |
MD78 |
ia. |
0.067 ± 0.004 μM (95%) |
ia. |
0.69 ± 0.02 μM (126%) |
3a
|
0.064 ± 0.002 |
ia. |
ia. |
ia. |
3b
|
0.027 ± 0.008 |
ia. |
ia. |
ia. |
3c
|
0.85 ± 0.003 |
ia. |
ia. |
ia. |
3d
|
0.57 ± 0.005 |
ia. |
ia. |
ia. |
5a
|
0.04 ± 0.017 |
ia. |
ia. |
ia. |
5b
|
0.023 ± 0.008 |
ia. |
ia. |
ia. |
5c
|
0.075 ± 0.007 |
ia. |
ia. |
ia. |
5d
|
0.027 ± 0.004 |
ia. |
ia. |
ia. |
8a
|
2.5 ± 0.5 |
ia. |
ia. |
ia. |
8b
|
1.3 ± 0.3 |
ia. |
ia. |
ia. |
8c
|
0.3 ± 0.04 |
@10 μM (65%) |
ia. |
ia. |
8d
|
1.2 ± 0.07 |
2.5 ± 0.3 μM (28%) |
ia. |
6 ± 0.1 μM (68%) |
Experimental
General
All starting materials, reagents and solvents were purchased from Alfa-Aesar (Karlsruhe, Germany), Sigma-Aldrich (Hannover, Germany), Apollo Scientific Ltd (Manchester, England), JRD Fluorochemicals, Ltd. (Surrey, England), Axon Medchem BV (Groningen, Netherlands) and used without further purification. TLC was performed using silica coated aluminum foil (particle size 60 μm) purchased from Merck KGaA (Darmstadt, Germany). Purification of synthesized compounds was performed on an Intelli Flash 310 Chromatograph from Varian Medical Systems (Darmstadt, Germany) using SF25-80 g and SF25-60 g columns, both loaded with silica gel (particle size 50 μm) and also purchased from Varian Medical Systems (Darmstadt, Germany). 1H (250/400 MHz) and 13C (64 MHz) were recorded on DPX250 and AV400 nuclear magnetic resonance spectrometers from Bruker (Karlsruhe, Germany). All spectra were analyzed with TopSpin software (Bruker, Karlsruhe, Germany). Tetramethylsilane was used as internal standard. DMSO-d6 and methanol-d4 were used as solvents. HPLC and mass analyses were performed on a LCMS 2020 from Shimadzu (Duisburg, Germany), under the use of a MultoHigh 100 RP 18, 3 μ, 100 × 2 mm column from CS Chromatography-Service GmbH (Langerwehe, Germany) with an acetonitrile/water gradient from 20–75%. Electron spray ionization spectra were recorded in positive (+) as well as negative (−) mode and UV was measured at two wavelengths (λ = 254 and 280 nm). High resolution mass spectroscopy was performed by a Thermo Scientific MALDI LTQ ORBITRAP XL. All final compounds had a purity ≥95% as determined by HPLC.
Murine embryonic 3T3-L1 fibroblasts were purchased from the American Type Culture Collection (ATCC, Manassas, VA, USA). COS-7 african green monkey kidney cells originated from Deutsche Sammlung für Mikroorgansimen und Zellkulturen (DSMZ, Braunschweig, Germany).
General procedure for preparation of compounds 1a–b, using the example of ethyl 2-(4-cyanophenoxy)propanoate (1a).
5 g (25.2 mmol) 4-hydroxybenzonitrile, 20.9 g (151 mmol) potassium carbonate, 2.1 g (12.6 mmol) potassium iodide and 3.3 ml (25.2 mmol) ethyl 2-bromopropanoate were refluxed in 50 ml acetone for 72 h. After cooling to room temperature, the mixture was filtered and the solvent was removed under reduced pressure. The crude residue was dissolved in 30 ml ethyl acetate, washed three times with 2 M sodium hydroxide solution and twice with 30 ml brine. The organic layer was dried over magnesium sulfate and the solvent was removed under reduced pressure. After purification by flash chromatography, using pure dichloromethane, compound 1a remained as a white powder (2.2 g, 40%); 1H NMR (250 MHz, DMSO-d6): δ = 7.75 (d, J = 8.9 Hz, 2H), 7.03 (d, J = 8.8 Hz, 2H), 5.13 (q, J = 7.2 Hz, 1H), 4.13 (q, J = 8.01 Hz, 2H), 1.52 (d, J = 6.9 Hz, 3H), 1.15 ppm (t, J = 7.3 Hz, 3H); MS-ESI+: m/z 242 [M + Na]+.
Ethyl 2-(4-(cyanomethyl)phenoxy)propanoate (1b).
Remained as a white powder (2 g, 38%); 1H NMR (250 MHz, DMSO-d6): δ = 7.29–6.88 (m, 4H), 4.69 (q, J = 5.7 Hz, 1H), 4.15 (q, J = 6 Hz, 2H), 3.94 (s, 2H), 1.51 (d, J = 6.8 Hz, 3H), 1.18 ppm (t, J = 6.8 Hz, 3H); MS-ESI+: m/z 256 [M + Na]+.
General procedure for preparation of compounds 2a–b, using the example of ethyl 2-(4-(aminomethyl)phenoxy)propanoate (2a).
1 g (4.6 mmol) ethyl 2-(4-cyanophenoxy)propanoate (1a), 0.1 g (0.21 mmol) palladium on carbon 10% and 0.78 ml (13.68 mmol) glacial acetic acid were stirred in 10 ml dry ethanol under argon atmosphere for 24 h. The catalyst was segregated over Celite® and the solvent was removed under reduced pressure. The crude product was dissolved in 25 ml of 2 M hydrochloric acid and washed with 20 ml cyclohexane. To the aqueous solution was cooled to 5 °C and by adding 1 M potassium hydroxide solution the pH was adjusted to a basic level. The aqueous layer was extracted with 25 ml ethyl acetate. The organic layer was dried over magnesium sulfate and the solvent was removed under reduced pressure. Without further purification, 2a remained as clear oil (0.69 g, 68%); 1H NMR (250 MHz, DMSO-d6): δ = 7.21–6.6 (m, 4H), 4.77 (q, J = 6.8 Hz, 1H), 4.12 (q, J = 6.7 Hz, 2H), 2.65 (s, 2H), 1.5 (d, J = 6.8 Hz, 3H), 1.17 ppm (t, J = 6.9 Hz, 3H); MS-ESI+: m/z 246 [M + Na]+.
Ethyl 2-(4-(aminoethyl)phenoxy)propanoat (2b).
Remained as clear oil (0.67 g, 66%); 1H NMR (250 MHz, DMSO-d6): δ = 7.13–6.75 (m, 4H,), 4.87 (q, J = 6.9 Hz, 1H), 4.14 (q, J = 7 Hz, 2H), 2.7 (t, J = 6.1, 2H), 2.6 (t, J = 6.5, 2H), 1.49 (d, J = 6.8 Hz, 3H), 1.18 ppm (t, J = 6.8 Hz); MS-ESI+: m/z 260 [M + Na]+.
General procedure for preparation of compounds 3a–b, using the example of ethyl 2-(4-((3-(4-chlorophenyl)ureido)methyl)phenoxy)propanoate (3a).
0.5 g (2.24 mmol) ethyl 2-(4-(aminomethyl)phenoxy)propanoate (2a), 0.59 ml (3.36 mmol) N,N-diisopropylethylamine and 0.29 ml (2.24 mmol) 4-chlorophenyl isocyanate were stirred in 5 ml dry dichloromethane under argon for 24 h. Then, the reaction mixture was diluted with further 10 ml dichloromethane and washed with 1 M hydrochloric acid. The organic layer was dried over magnesium sulfate and the solvent was removed under reduced pressure. The crude product was purified by flash chromatography with hexane/ethyl acetate 3
:
1. Compound 3a remained as a white powder (0.17 g, 20%); 1H NMR (250 MHz, DMSO-d6): δ = 8.7 (s, 1H), 7.5–6.6 (m, 8H), 5.78 (t, J = 6.3 Hz, 1H), 4.92 (q, J = 6.7 Hz, 1H), 4.22 (d, J = 5.7 Hz, 2H), 4.14 (q, J = 6.8 Hz, 2H), 1.5 (d, J = 6.7 Hz, 3H), 1.18 ppm (t, J = 7.6 Hz, 3H); 13C NMR (75.4 MHz, DMSO-d6): 172, 159.1, 154.1, 134.9, 132.3, 129.4, 129.3, 129.1, 128.6, 128.4, 122, 119.1, 114.3, 114.6, 79, 61.3, 50.9, 17.2, 12.3 ppm; HRMS-MALDI m/z [M + H]+ calcd for C19H21ClN2O4: 376.1193, found 376.1192.
Ethyl 2-(4-((3-(4-chlorophenyl)ureido)ethyl)phenoxy)propanoate (3b).
Remained as a white powder (0.15 g, 18%); 1H NMR (250 MHz, DMSO-d6): δ = 8.62 (s, 1H), 7.44–6.8 (m, 8H), 6.13 (t, J = 5.3 Hz, 1H), 4.9 (q, J = 6.7 Hz, 1H), 4.15 (q, J = 8 Hz, 2H), 2.68 (t, J = 8.1 Hz, 2H), 1.5 (d, J = 6.1 Hz, 3H), 1.18 ppm (t, J = 6.6 Hz, 3H); 13C NMR (75.4 MHz, DMSO-d6): 173, 162.1, 149.1, 136.9, 134.3, 130.4, 129.7, 129.2, 128.9, 128.6, 120, 119.5, 114.9, 114, 80, 61.4, 48.5, 36.7, 18.2, 11.3 ppm; HRMS-MALDI m/z [M + H]+ calcd for C20H23ClN2O4: 390.1351, found 390.1352.
General procedure for synthesis of compounds 3c–d, 5c, 5d using the example of 2-(4-((3-(4-chlorophenyl)ureido)methyl)phenoxy)propionic acid (3c).
100 mg (0.27 mmol) ethyl 2-(4-((3-(4-chlorophenyl)ureido)methyl)phenoxy)propanoate (3a) and 74 mg (1.33 mmol) potassium hydroxide were dissolved in 2 ml of a solvent mixture containing tetrahydrofuran, water and methanol in a ratio 1/2/1 and stirred under microwave irradiation in a Initiator Microwave Synthesizer from Biotage (Uppsala, Sweden) at 70 °C for 30 minutes. The organic solvents were removed under reduced pressure. With the addition of a few drops 12 M hydrochloric acid 3c precipitated in the aqueous phase. After filtration the product was dried by lyophilization and 3c remained as white powder (42 mg, 45%); 1H NMR (250 MHz, DMSO-d6): δ = 8.65 (s, 1H), 7.45–6.8 (m, 8H), 6.58 (t, J = 5.75 Hz, 1H), 4.8 (q, J = 6.5 Hz, 1H), 4.21 (d, J = 6.1 Hz, 2H), 1.49 ppm (d, J = 7.2 Hz, 3H); 13C NMR (75.4 MHz, DMSO-d6): 178, 159.1, 147.1, 135.9, 133.3, 131.4, 129.9, 129.7, 129, 128.7, 119, 118.1, 114.5, 113, 76, 49.5, 19.2 ppm, HRMS-MALDI m/z [M + H]+ calcd for C17H17ClN2O4: 348.0885, found 348.0883.
2-(4-((3-(4-Chlorophenyl)ureido)ethyl)phenoxy)propionic acid (3d).
Remained as a white powder (39 mg, 42%); 1H NMR (250 MHz, DMSO-d6): δ = 8.63 (s, 1H), 7.44–6.79 (m, 8H), 6.14 (t, J = 6 Hz, 1H), 4.78 (q, J = 6.5 Hz, 1H), 3.2 (q, J = 6.9 Hz, 2H), 1.49 ppm (d, J = 6.5 Hz, 3H); 13C NMR (75.4 MHz, DMSO-d6): 180, 159.1, 148.1, 137.2, 135.3, 129.9, 129.6, 129.2, 128.9, 128.5, 118, 117.5, 114.8, 114.1, 79.1, 49.5, 36.5, 18.1 ppm; HRMS-MALDI m/z [M + H]+ calcd for C18H19ClN2O4: 362.1032, found 362.1031.
General procedure for amide synthesis of compounds 4, 6a and 6b, described with the synthesis of 1-(4-formylphenyl)-N-(2-(trifluoromethyl)benzyl)piperidine-4-carboxamide (4).
1 g (4.29 mmol) 1-(4-formylphenyl)piperidine-4-carbonic acid, 0.66 ml (4.72 mmol) triethylamine and 0.61 ml (4.72 mmol) isobutyl chloroformiate were stirred in 40 ml dry chloroform at 0 °C under argon atmosphere for 1 h. Subsequently 0.66 ml (4.29 mmol) 2-(trifluoromethyl)benzylamine were added and the mixture was further stirred at room temperature for 12 h. The reaction mixture was washed three times with 20 ml of 2 M hydrochloric acid, three times with 20 ml of 2 M sodium hydroxide solution and two times with 20 ml brine. The organic layer was dried over magnesium sulfate and the solvent was removed under reduced pressure. The product was recrystallized from ethyl acetate/hexane. Compound 4 remained as a white powder (0.9 g, 54%); 1H NMR (250 MHz, DMSO-d6): δ = 9.8 (s, 1H), 8.56 (t, J = 6.4 Hz, 1H), 7.82–7.12 (m, 8H), 4.53 (d, J = 6.1 Hz, 2H), 3.93–2.9 (m, 4H), 2.5 (m, 1H), 1.88–1.74 ppm (m, 4H); MS-ESI−: m/z 425 [M + Cl]−.
Preparation of (E)-2-(4-(4-((2-(trifluoromethyl)benzyl)carbamoyl)piperidine-1-yl)benzylidene)-alpha-ethylcinnamate (5a).
200 mg (5.12 mmol) sodium hydride 63% and 9.1 ml (3.84 mmol) triethyl 2-phosphonobutyrate were stirred in 5 ml dry tetrahydrofuran at 0 °C under argon atmosphere for 30 minutes. Then, 1 g (2.56 mmol) 1-(4-formylphenyl)-N-(2-(trifluoromethyl)benzyl)piperidine-4-carboxamide (4) was dissolved in 10 ml dry tetrahydrofuran and added to the reaction mixture. After 2 h the reaction was stopped with the addition of 25 ml water. The mixture was diluted with 10 ml ethyl acetate and washed three times with brine. The organic layer was dried over magnesium sulfate and the solvent was removed under reduced pressure. The product was recrystallized from ethyl acetate/hexane. Compound 5a remained as a white powder (901 mg, 72%); 1H NMR (250 MHz, DMSO-d6): δ = 8.5 (t, J = 6 Hz, 1H), 7.75–7.05 (m, 9H), 4.47 (d, J = 7.4 Hz, 2H), 4.2 (q, J = 6.3 Hz, 2H), 3.92–2.8 (m, 4H), 2.9 (q, J = 12 Hz, 2H), 2.5 (m, 1H), 1.92–1.68 (m, 4H), 1.29 (t, J = 7.3 Hz, 3H), 1.14 ppm (t, J = 8.3 Hz, 3H); 13C NMR (75.4 MHz, DMSO-d6): 178.9, 165, 142.9, 139.5, 135, 132.1, 132, 128, 129.1, 127.9, 127.8, 126.5, 125.2, 124.5, 113, 113.2, 112, 60, 51.6, 51, 39.3, 25.9, 25.8, 19.6, 13.9, 11.1; HRMS-MALDI m/z [M + H]+ calcd for C27H31F3N2O3: 488.2295, found 488.2295.
(E)-2-(4-(4-((2-(Trifluoromethyl)benzyl)carbamoyl)piperidine-1-yl)benzylidene)-alpha-ethylcinnamic acid (5d).
5d remained as a white powder (57 mg, 60%); 1H NMR (250 MHz, DMSO-d6): δ = 8.49 (t, J = 5.7 Hz, 1H), 7.72–7.1 (m, 9H), 4.44 (d, J = 5.4 Hz, 2H), 3.93–2.86 (m, 4H), 2.8 (q, J = 9 Hz, 2H), 2.49 (m, 1H), 1.9–1.7 (m, 4H), 1.09 ppm (t, J = 7.1 Hz, 3H); 13C NMR (75.4 MHz, DMSO-d6): 179, 170.1, 142.7, 136.5, 134, 132.5, 132.1, 127, 129.5, 127.1, 126.9, 126, 125.1, 124.4, 113.1, 113, 111, 51.7, 52, 38.3, 26.1, 25.7, 19.3, 12.5; HRMS-MALDI m/z [M + H]+ calcd for C25H27F3N2O3: 460.2200, found 460.2201.
Preparation of ethyl 2-(4-(4-((2-(trifluoromethyl)benzyl)carbamoyl)piperidine-1-yl)benzyl)butanoate (5b).
200 mg (0.41 mmol) (E)-2-(4-(4-((2-(trifluoromethyl)benzyl)carbamoyl)piperidine-1-yl)benzylidene)-alpha-ethylcinnamate (5a) and 100 mg magnesium chips were stirred with 4 ml dry methanol under argon atmosphere. After 12 h the reaction was stopped by addition of 4 ml 2 M hydrochloric acid. The product was extracted with 10 ml ethyl acetate. The organic layer was dried over magnesium sulfate and the solvent was removed under reduced pressure. For purification a flash chromatography was used with ethyl acetate and hexane (1
:
1) as mobile phase. 5d remained as white powder (161 mg, 80%); 1H NMR (250 MHz, DMSO-d6): δ = 8.56 (t, J = 5.6 Hz, 1H), 7.81–7.04 (m, 8H), 4.52 (d, J = 6.2 Hz, 2H), 4.07 (q, J = 7.2 Hz, 2H), 3.92–2.65 (m, 4H), 2.74 (q, J = 8 Hz), 2.4 (m, 1H), 2.3–2.29 (m, 1H), 1.98–1.72 (m, 4H), 1.23 (t, J = 6.3 Hz, 3H), 1.14 ppm (t, J = 7.9 Hz, 3H); 13C-NMR (75.4 MHz, DMSO-d6): 178.1, 174.2, 142.8, 138.5, 131, 129.2, 129.1, 128, 127.9, 126.4, 125.1, 124.1, 113, 112.9, 112, 59.8, 51.7, 51, 48, 39.1, 35, 26.1, 25.8, 19.6, 14.1, 13.1; HRMS-FAB m/z [M + H]+ calcd for C27H32F3N2O3: 490.2442, found 490.2441.
2-(4-(4-((2-(Trifluoromethyl)benzyl)carbamoyl)piperidine-1-yl)benzyl)butanoic acid (5c).
5c remained as a white powder (58 mg, 61%); 1H NMR (250 Hz, DMSO-d6): δ = 6.64 (t, J = 6.1 Hz, 1H), 7.81–7.29 (m, 8H), 4.53 (d, J = 6.1 Hz, 2H), 3.77–2.7 (m, 4H), 2.6 (q, J = 7 Hz, 2H), 2.5 (m, 1H), 2.31–2.28 (m, 1H), 1.98–2.11 (m, 4H), 0.94 ppm (t, J = 7.4 Hz, 3H); 13C-NMR (75.4 MHz, DMSO-d6): 179.5, 178.1, 142.1, 137.5, 132, 129, 128.9, 128, 127.8, 125.9, 125.1, 124.6, 114, 113.9, 112, 51.9, 50.1, 49, 39.4, 34, 26.1, 25.7, 18.1, 12.1; HRMS-FAB m/z [M + H]+ calcd for C25H29F3N2O3: 462.2133, found 462.2131.
tert-Butyl 4-((2-(trifluoromethyl)benzyl)carbamoyl)piperidine-1-carboxylate (6a).
6a remained as an unclear oil (1.146 g, 68%); 1H NMR (250 MHz, DMSO-d6): δ = 8.49 (t, J = 5.4 Hz, 1H), 7.78–7.49 (m, 4H), 4.49 (d, J = 5.4 Hz, 2H), 4.06–3.96 (m, 2H), 2.88–2.72 (m, 2H), 2.51–2.44 (m, 1H), 1.83–1.73 (m, 2H), 1.51–1.5 (m, 2H), 1.45 ppm (s, 9H). MS-ESI−: m/z 410 [M + Cl−].
tert-Butyl (4-oxo-4-((2-(trifluoromethyl)benzyl)amino)butyl)carbamate (6b).
6b remained as an unclear oil (0.975 g, 55%); 1H NMR (250 MHz, DMSO-d6): δ = 8.45 (t, J = 5.9 Hz, 1H), 7.79–7.5 (m, 4H), 6.88 (t, J = 9.1 Hz, 1H), 4.49 (d, J = 6 Hz, 2H), 2.98 (q, J = 6.6 Hz, 2H), 2.24 (t, J = 7.2 Hz, 2H), 1.7 (q, J = 7.3 Hz, 2H), 1.44 ppm (s, 9H). MS-ESI+: m/z 383 [M + Na]+.
General procedure of Boc-protecting group cleavage for compounds 7a and 7b, described with the synthesis of N-(2-(trifluoromethyl)benzyl)piperidine-4-carboxamide (7a).
230 mg (0.6 mmol) tert-butyl 4-((2-(trifluoromethyl)benzyl)carbamoyl)piperidine-1-carboxylate (6a) and 0.92 ml (11.9 mmol) trifluoroacetic acid were stirred in 4 ml dichloromethane for 60 minutes. The organic solvent was removed under reduced pressure and the crude residue was dissolved in 10 ml of 2 M hydrochloric acid. The acidic solution was washed twice with 10 ml ethyl acetate. By the addition of 8 M sodium hydroxide solution the pH was adjusted to a basic level and the product was extracted with ethyl acetate (3 × 15 mL). The organic layer was dried over magnesium sulfate and the solvent was removed under reduced pressure. Without further purification, 7a remained as a colorless oil (0.13 g, 76%); 1H NMR (250 MHz, DMSO-d6): δ = 8.42 (t, J = 5.6 Hz, 1H), 7.79–7.48 (m, 4H), 4.48 (d, J = 6.2 Hz, 2H), 3.08–2.99 (m, 2H), 2.61–2.5 (m, 2H), 2.44–2.33 (m, 1H), 1.78–1.68 ppm (m, 2H), 1.46–1.6 (m, 2H). MS-ESI+: m/z 287 [M + H+].
4-Amino-N-(2-(trifluoromethyl)benzyl)butanamide (7b).
7b remained as a colorless oil (71 mg, 50%); 1H NMR (250 MHz, DMSO-d6): δ = 8.4 (t, J = 5.4 Hz, 1H), 7.74–7.45 (m, 4H), 4.44 (d, J = 5.6 Hz, 2H), 2.66 (t, J = 7.4 Hz, 2H), 2.23 (t, J = 7.1 Hz, 2H), 1.62 ppm (q, J = 7.1 Hz, 2H). MS-ESI+: m/z 261 [M + H+].
General procedure of compounds 8a and 8b is described by the synthesis of ethyl 2-((4-chloro-6-(4-((2-(trifluoromethyl)benzyl)carbamoyl)piperidine-1-yl)pyrimidine-2-yl)thio)octanoate (8a).
100 mg ethyl 2-((4,6-dichloropyrimidine-2-yl)thio)octanoate were dissolved in 2 ml dry acetonitrile under argon atmosphere. A mixture of 0.04 ml (0.285 mmol) triethylamine and 81 mg (0.285 mmol) N-(2-(trifluoromethyl)benzyl)piperidine-4-carboxamide (7a) were added slowly and the mixture was stirred for 12 h. With the addition of water, the product precipitated, was filtered off and dried by lyophilization. Without further purification, 8a remained as a white powder (0.116 g, 68%); 1H NMR (250 MHz, methanol-d4: δ = 7.62–7.31 (m, 4H), 6.44 (s, 1H), 4.47 (s, 2H), 4.22 (t, J = 7.2 Hz, 1H), 4.07 (q, J = 7.3 Hz, 2H), 2.99–2.87 (m, 2H), 2.62–2.47 (m, 1H), 1.9–1.48 (m, 6H), 1.47–1.11 (m, 13H), 0.8 ppm (t, J = 7.6 Hz, 3H); 13C NMR (75.4 MHz, methanol-d4): 178.8, 174.6, 171.2, 167.2, 160.6, 139.3, 131.7, 129.2, 127.4, 126.7, 125.1, 113.1, 102.8, 60.1, 52, 51.2, 44.7, 39.1, 35.2, 30.1, 29.8, 27.5, 25.7, 25.8, 32.6, 23.1, 14, 13.6; HRMS-MALDI m/z [M + H]+ calcd for C27H35ClF3N2O3: 600.2154, found 600.2153.
Ethyl 2-((4-chloro-6-((4-oxo-4-((2-(trifluoromethyl)benzyl)amino)butyl)amino)pyrimidin-2-yl)thio)octanoat (8b).
8b remained as a white powder (0.508 g, 50%); 1H NMR (250 MHz, methanol-d4): δ = 7.62–7.3 (m, 4H), 6.07 (s, 1H), 4.47 (s, 2H), 4.29 (t, J = 7.3 Hz, 1H), 4.04 (q, J = 7.1 Hz, 2H), 3.49–3.27 (m, 2H), 2.27 (t, J = 7.2 Hz, 2H), 1.82 (q, J = 7.8 Hz, 2H), 1.38–1.11 (m, 13H), 0.79 (t, J = 7.1 Hz, 3H) ppm; 13C NMR (75.4 MHz, methanol-d4): 178.9, 175.6, 170.2, 166.2, 158.6, 137.3, 134.7, 129.2, 128.4, 128.7, 126.1, 114.1, 103.8, 58.1, 50.6, 43.7, 34.2, 32.6, 31.7, 29.1, 28.8, 27.5, 27.4, 23.1, 14.7, 12.6; HRMS-MALDI m/z [M + H]+ calcd for C25H33ClF3N2O3: 574.1997, found 574.1998.
2-((4-Chloro-6-(4-((2-(trifluoromethyl)benzyl)carbamoyl)piperidine-1-yl)pyrimidine-2-yl)thio)octanoic acid (8c).
To a solution of 8b (0.3 g, 0.5 mmol) in THF (10 mL) was added LiOH·H2O (0.06 g, 1.5 mmol) dissolved in water (2 mL). The reaction was stirred for 24 h at 45 °C. The organic solvent was removed under reduced pressure, and the residue was diluted with 3 ml of water. The aqueous layer was acidified by addition of few drops of 12 M HCl solution. The resulting precipitate was separated by filtration and without further purification, 8c remained as a white powder (70 mg, 57%); 1H NMR (250 MHz, methanol-d4): δ = 7.63–7.31 (m, 4H), 6.39 (s, 1H), 4.47 (s, 2H), 4.19 (t, J = 7.2 Hz, 1H), 2.98–2.86 (m, 2H), 2.61–2.48 (m, 1H), 1.9–1.53 (m, 6H), 1.29–1.11 ppm (m, 13H). 13C NMR (75.4 MHz, methanol-d4): 179.5, 178.1, 171.1, 165.2, 159.6, 137.3, 132.7, 128.2, 128.1, 126.6, 125, 112.1, 102.9, 48.1, 48, 43.7, 40, 33.2, 32.1, 31.6, 28.8, 27.1, 25.1, 25, 21.1, 12, 11.6; HRMS-FAB m/z [M + H]+ calcd for C25H31ClF3N2O3: 572.1850, found 572.1849.
2-((4-Chloro-6-((4-oxo-4-((2-(trifluoromethyl)benzyl)amino)butyl)amino)pyrimidine-2-yl)thio)octanoic acid (8d) was synthesized according to the procedure described with the synthesis of compound 8c.
8d remained as a white powder (31 mg, 33%); 1H NMR (250 MHz, methanol-d4): δ = 7.62–7.32 (m, 4H), 6.03 (s, 1H), 4.47 (s, 2H), 4.24 (t, J = 7.3 Hz, 1H), 3.51–3.33 (m, 2H), 2.25–2.35 (m, 2H), 1.96–1.73 (m, 2H), 1.45–1.12 ppm (m, 13H); 13C NMR (75.4 MHz, methanol-d4): 181.1, 177.9, 171.2, 165.2, 157.6, 138.3, 133.7, 128.2, 128.1, 127.7, 126.9, 113.1, 101.8, 51.6, 42.7, 33.2, 31.6, 30, 29.5, 28.7, 27, 26.1, 21.1, 13.7; HRMS-MALDI m/z [M + H]+ calcd for C23H29ClF3N2O3: 546.1688, found 546.1683.
PPAR activity assay
Evaluation of PPAR activity was performed as published before.24 In brief, COS-7 cells were grown in DMEM high glucose, supplemented with 10% fetal calf serum (FCS), 1% sodium pyruvate (SP) and 1% penicillin/streptomycin (PS) at 37 °C and 5% CO2. The day before transfection, COS-7 cells were seeded in 96-well plates with a density of 30
000 cells per well. Transient transfection was carried out using Lipofectamine LTX reagent (Invitrogen, Carlsbad, CA, USA) according to the manufacturer's protocol with pFR-Luc (Stratagene), pRL-SV40 (Promega) and the Gal4-fusion receptor plasmids (pFA-CMV-hPPAR-LBD) of the respective PPAR subtype. 5 h after transfection, medium was changed to DMEM without phenol red and 10% FCS, supplemented with 1% SP, 1% PS and 1% L-glutamine, now additionally containing 0.1% DMSO and the respective test compound or 0.1% DMSO alone as untreated control. Each concentration was tested in triplicate wells and each experiment was repeated independently at least three times. Following overnight incubation with the test compounds, cells were assayed for luciferase activity using Dual-Glo™ Luciferase Assay System (Promega) according to the manufacturer's protocol. Luminescence was measured using a microplate reader (Infinite M200, Tecan Group Ltd., Crailsheim, Germany). Normalization for transfection efficacy and cell growth was done by division of the Firefly luciferase data by Renilla luciferase data resulting in relative light units. Activation factors were obtained by dividing by DMSO control. EC50 and standard deviation values were calculated by mean values of at least three determinations by SigmaPlot 2001 (Systat Software GmbH, Erkrath, Germany) using a four-parameter logistic regression. All compounds were evaluated by comparison of the achieved maximum effect to that of the reference compound (pioglitazone for PPARγ, GW7647 for PPARα,25 and L165041 for PPARδ26 each at 1 μM). Data are expressed as mean ± SE; n ≥ 3.
sEH activity assay
The IC50 values of the compounds were determined by a fluorescence-based assay system of 96-well format. As substrate non-fluorescent PHOME (3-phenyl-cyano-(6-methoxy-2-naphthalenyl)methyl ester-2-oxirane-acetic acid, Cayman Chemicals) was used, which can be hydrolyzed by the sEH to the fluorescent 6-methoxynaphtaldehyde.27 The formation of the product was measured (λem = 330 nm, λex = 465 nm) by a Tecan Infinite F200 Pro plate reader. Therefore, recombinant human sEH (2 μg per well) in Bis-Tris buffer pH 7 with 0.1 mg ml−1 BSA containing a final concentration of 0.01% Triton-X 100. 100 μl of protein were incubated with different concentrations of compounds (DMSO with final concentration of 1%) for 30 min. at room temperature. After that 10 μl of substrate were added (final concentration 50 μM). The hydrolysed substrate was measured for 30 min (one point every minute). A blank control (no protein and no compound) as well as a positive control (no compound) was executed. All measurements were performed in triplicates.
Differentiation of murine 3T3-L1 cells
3T3-L1 cells were subcultured in DMEM containing 10% newborn calf serum in a humidified atmosphere at 37 °C, 5% CO2. Cells were differentiated into adipocytes for 14 days according to the method of Zebisch et al.28 Briefly, cells were seeded in 6-well plates (2.5 × 106 per well). Differentiation was started at day 3 by addition of 1 μg ml−1 insulin, 0.25 μM dexamethasone and 0.5 mM isobutylmethylxanthine in DMEM supplemented with 10% fetal calf serum. At day 5 medium was replaced by medium containing only insulin for 2 more days. After this, cells were kept for lipid droplet accumulation in basal medium without additions until day 15. Rosiglitazone (2 μM) and N-cyclohexyl-N′-(iodophenyl)urea (CIU) (10 μM) were used as PPARγ and sEH positive controls, respectively. Differentiation of 3T3-L1 cells was confirmed by Oil Red O staining. Cells were washed with PBS and subsequently fixed for 60 minutes with a formaldehyde solution (4% in PBS). After this, cells were rinsed with 60% isopropanol and incubated with Oil Red O solution (0.3%) for 120 minutes.
Conclusions
In this study diverse scaffolds of sEH inhibitors and PPAR agonists were fused in order to obtain a dual sEH/PPAR modulator. All three designed compound classes displayed potent inhibitory activity against sEH. Obviously, inhibitory activity against sEH can be easily introduced in a multi-target compound by incorporating a secondary urea or N-benzyl benzamide fragment, as it was previously shown by Hwang et al.29 and Meirer et al.30 However, this does not hold true for PPAR activation. Although all compounds corresponded to the general pharmacophore of PPAR agonists,31 only 8d displayed the desired PPAR modulatory activity. Even small changes in the linker region of bezafibrate displayed in 3c and 3d led to complete loss of PPAR agonistic properties. This observation is in line with our previous observation on dual sEH/PPAR modulators, where almost all members of a combinatorial library displayed sEH inhibitory activity, whereas only few compounds were indeed able to activate PPARγ and PPARα.14 Small N-benzyl benzamides were previously identified as a merged pharmacophores of dual sEH/PPARγ modulators with oral activity, however without impact on PPARα.32 Thus, it seems to be difficult to design-in sEH inhibitory activity in a dual PPARα/γ agonist.
The triple active agent 8d resulting from this study is an interesting tool for the investigation of multi-target compounds for MetS treatment. Due to the fact that it comprises sEH inhibitory and PPARα/γ partial agonistic properties, it might simultaneously reduce dyslipidaemia, hyperglycaemia, and hypertension. With its partial agonistic profile on both PPAR subtypes, 8d should also be less prone of the typical TZD side effects including weight-gain and edema causing fluid retention. Further in vivo evaluation of 8d will provide valuable insights into multi-target treatment options for MetS.
Acknowledgements
This work was supported by the Else-Kröner-Fresenius Foundation graduate school Translational Research Innovation Pharma (TRIP) and Deutsche Forschungsgemeinschaft (DFG; Sachbeihilfe PR1405/1-2; SFB 1039 Teilprojekt A07). R. B. and O. D. thank the graduate school Translational Research Innovation Pharma (TRIP) for PhD scholarships.
Notes and references
- E. S. Ford, W. H. Giles and W. H. Dietz, JAMA, 2002, 287, 356–359 CrossRef PubMed.
- R. H. Eckel, S. M. Grundy and P. Z. Zimmet, Lancet, 2005, 365, 1415–1428 CrossRef CAS.
- S. M. Grundy, Nat. Rev. Drug Discovery, 2006, 5, 295–309 CrossRef CAS PubMed.
- D. V. Rollason and N. Vogt, Drugs Aging, 2012, 20, 817–832 Search PubMed.
- M. G. Katselou, A. N. Matralis and A. P. Kourounakis, Curr. Med. Chem., 2014, 21, 2743–2787 CrossRef CAS PubMed.
- J. D. Imig, K. A. Walsh, M. A. Hye Khan, T. Nagasawa, M. Cherian-Shaw, S. M. Shaw and B. D. Hammock, Exp. Biol. Med., 2012, 237, 1402–1412 CrossRef CAS PubMed.
- C. Duval, M. Müller and S. Kersten, Biochim. Biophys. Acta, Mol. Cell Biol. Lipids, 2007, 1771, 961–971 CrossRef CAS PubMed.
- C. Lamers, M. Schubert-Zsilavecz and D. Merk, Expert Opin. Ther. Pat., 2012, 22, 803–841 CrossRef CAS PubMed.
- I. Fleming, Pharmacol. Rev., 2014, 66, 1106–1140 CrossRef CAS PubMed.
- J. Wray and D. Bishop-Bailey, Exp. Physiol., 2008, 93, 148–154 CrossRef CAS PubMed.
- B. M. De Taeye, C. Morisseau, J. Coyle, J. W. Covington, A. Luria, J. Yang, S. B. Murphy, D. B. Friedman, B. B. Hammock and D. E. Vaughan, Obesity, 2010, 18, 489–498 CrossRef CAS PubMed.
- Y. Liu, Y. Zhang, K. Schmelzer, T.-S. Lee, X. Fang, Y. Zhu, A. A. Spector, S. Gill, C. Morisseau, B. D. Hammock and J. Y.-J. Shyy, Proc. Natl. Acad. Sci. U. S. A., 2005, 102, 16747–16752 CrossRef CAS PubMed.
- D. Xu, B. B. Davis, Z. Wang, S. Zhao, B. Wasti, Z. Liu, N. Li, C. Morisseau, N. Chiamvimonvat and B. D. Hammock, Int. J. Cardiol., 2013, 167, 1298–1304 CrossRef PubMed.
- E. Buscató, R. Blöcher, C. Lamers, F.-M. Klingler, S. Hahn, D. Steinhilber, M. Schubert-Zsilavecz and E. Proschak, J. Med. Chem., 2012, 55, 10771–10775 CrossRef PubMed.
- G. A. Gomez, C. Morisseau, B. D. Hammock and D. W. Christianson, Biochemistry (Moscow), 2004, 43, 4716–4723 CrossRef CAS PubMed.
- T. Asaki, T. Aoki, T. Hamamoto, Y. Sugiyama, S. Ohmachi, K. Kuwabara, K. Murakami and M. Todo, Bioorg. Med. Chem., 2008, 16, 981–994 CrossRef CAS PubMed.
- P. L. Podolin, B. J. Bolognese, J. F. Foley, E. Long, B. Peck, S. Umbrecht, X. Zhang, P. Zhu, B. Schwartz, W. Xie, C. Quinn, H. Qi, S. Sweitzer, S. Chen, M. Galop, Y. Ding, S. L. Belyanskaya, D. I. Israel, B. A. Morgan, D. J. Behm, J. P. Marino, E. Kurali, M. S. Barnette, R. J. Mayer, C. L. Booth-Genthe and J. F. Callahan, Prostaglandins Other Lipid Mediators, 2013, 104-105, 25–31 CrossRef CAS PubMed.
- M. Nomura, T. Tanase, T. Ide, M. Tsunoda, M. Suzuki, H. Uchiki, K. Murakami and H. Miyachi, J. Med. Chem., 2003, 46, 3581–3599 CrossRef CAS PubMed.
- M. Ohashi, I. Nakagome, J.-I. Kasuga, H. Nobusada, K. Matsuno, M. Makishima, S. Hirono, Y. Hashimoto and H. Miyachi, Bioorg. Med. Chem., 2012, 20, 6375–6383 CrossRef CAS PubMed.
- D. Merk, M. Zettl, D. Steinhilber, O. Werz and M. Schubert-Zsilavecz, Future Med. Chem., 2015, 7, 1597–1616 CrossRef CAS PubMed.
- C. Lamers, M. Dittrich, R. Steri, E. Proschak and M. Schubert-Zsilavecz, Bioorg. Med. Chem. Lett., 2014, 24, 4048–4052 CrossRef CAS PubMed.
- G. Navarrete-Vázquez, H. Torres-Gómez, S. Hidalgo-Figueroa, J. J. Ramírez-Espinosa, S. Estrada-Soto, J. L. Medina-Franco, I. León-Rivera, F. J. Alarcón-Aguilar and J. C. Almanza-Pérez, Bioorg. Med. Chem. Lett., 2014, 24, 4575–4579 CrossRef PubMed.
- H. E. Xu, M. H. Lambert, V. G. Montana, K. D. Plunket, L. B. Moore, J. L. Collins, J. A. Oplinger, S. A. Kliewer, R. T. Gampe, D. D. McKee, J. T. Moore and T. M. Willson, Proc. Natl. Acad. Sci. U. S. A., 2001, 98, 13919–13924 CrossRef CAS PubMed.
- O. Rau, M. Wurglics, A. Paulke, J. Zitzkowski, N. Meindl, A. Bock, T. Dingermann, M. Abdel-Tawab and M. Schubert-Zsilavecz, Planta Med., 2006, 72, 881–887 CrossRef CAS PubMed.
- P. J. Brown, L. W. Stuart, K. P. Hurley, M. C. Lewis, D. A. Winegar, J. G. Wilson, W. O. Wilkison, O. R. Ittoop and T. M. Willson, Bioorg. Med. Chem. Lett., 2001, 11, 1225–1227 CrossRef CAS PubMed.
- J. Berger, M. D. Leibowitz, T. W. Doebber, A. Elbrecht, B. Zhang, G. Zhou, C. Biswas, C. A. Cullinan, N. S. Hayes, Y. Li, M. Tanen, J. Ventre, M. S. Wu, G. D. Berger, R. Mosley, R. Marquis, C. Santini, S. P. Sahoo, R. L. Tolman, R. G. Smith and D. E. Moller, J. Biol. Chem., 1999, 274, 6718–6725 CrossRef CAS PubMed.
- N. M. Wolf, C. Morisseau, P. D. Jones, B. Hock and B. D. Hammock, Anal. Biochem., 2006, 355, 71–80 CrossRef CAS PubMed.
- K. Zebisch, V. Voigt, M. Wabitsch and M. Brandsch, Anal. Biochem., 2012, 425, 88–90 CrossRef CAS PubMed.
- S. H. Hwang, K. M. Wagner, C. Morisseau, J.-Y. Liu, H. Dong, A. T. Wecksler and B. D. Hammock, J. Med. Chem., 2011, 54, 3037–3050 CrossRef CAS PubMed.
- K. Meirer, C. B. Rödl, J. M. Wisniewska, S. George, A.-K. Häfner, E. Buscató, F.-M. Klingler, S. Hahn, D. Berressem, S. K. Wittmann, D. Steinhilber, B. Hofmann and E. Proschak, J. Med. Chem., 2013, 56, 1777–1781 CrossRef CAS PubMed.
- H. Zettl, R. Steri, M. Lämmerhofer and M. Schubert-Zsilavecz, Bioorg. Med. Chem. Lett., 2009, 19, 4421–4426 CrossRef CAS PubMed.
- R. Blöcher, C. Lamers, S. K. Wittmann, D. Merk, M. Hartmann, L. Weizel, O. Diehl, A. Brüggerhoff, M. Boß, A. Kaiser, T. Schader, T. Göbel, M. Grundmann, C. Angioni, J. Heering, G. Geisslinger, M. Wurglics, E. Kostenis, B. Brüne, D. Steinhilber, M. Schubert-Zsilavecz, A. S. Kahnt and E. Proschak, J. Med. Chem., 2016, 59, 61–81 CrossRef PubMed.
Footnote |
† The authors declare no competing interests. |
|
This journal is © The Royal Society of Chemistry 2016 |