DOI:
10.1039/C6GC01099G
(Communication)
Green Chem., 2016,
18, 4531-4535
Tin-free visible light photoredox catalysed cyclisation of enamides as a mild procedure for the synthesis of γ-lactams†
Received
18th April 2016
, Accepted 24th June 2016
First published on 13th July 2016
Abstract
The first visible light mediated tin-free cyclisation of α-chloroenamides leading to the synthesis of substituted γ-lactams with excellent stereoselectivity is reported. The protocol employs the single-electron reduction of activated C–Cl bonds, which are typically inert towards reduction.
The opportunity to realise unique transformations has attracted the attention of the chemistry community and stimulated, among others, the development of protocols involving the formation of free radicals by single-electron transfer (SET) processes.1 In particular, free-radical generation from organohalides represents a straightforward way to achieve reactive, open-shell carbon-centred radicals, which has found outstanding applications in natural product synthesis.2
However, the requirement of highly toxic radical reducing agents and initiators such as tin hydrides or AIBN, as well as specific and hard to manage equipment stimulated the development of new methods for the generation of free-radicals under benign conditions.3–6 An alternative is represented by visible light photocatalysis7,8 that, by means of transition-metal polypyridyl complexes or metal free photosensitizers, provides a valuable and green methodology for the single-electron reduction of carbon–halogen bonds. Yet, this approach seems to be limited to activated carbon–bromine/iodine bonds9 while only a few examples are reported when the less reactive organochlorides are involved, due to a more negative reduction potential as a consequence of higher carbon–halide bond dissociation energy (BDE).10 Specifically in the latter case, it is known that the BDE for the carbon–chlorine bond is significantly higher than those measured for the carbon–bromine/iodine bonds (CH3–Cl, 83 kcal mol−1; CH3–Br, 71 kcal mol−1; CH3–I, 57 kcal mol−1),11 and therefore the mesolysis by SET often requires prohibitive energies for most of the common visible light photocatalysts.
Nevertheless, the stability of organochloride derivatives, often along with their low cost compared to the corresponding bromide/iodide precursors, stresses the importance of available methods able to overcome the energetic and selectivity issues associated with the functionalisation of this bond. In this context, we wondered if visible light photoredox catalysis can be applied as an alternative to the less favorable agent Bu3SnH in the synthesis of γ-lactams through a formal 5-endo-trig cyclisation.12,13 This is initiated by the reduction of the carbon–halogen bond of α-haloenamide 1 to form the corresponding N-alkenyl α-carbamoylmethyl radical B (Scheme 1).14 Despite the stereoelectronic problems associated with this type of reaction (Baldwin–Beckwith's rule), γ-lactams are mainly formed compared to the directly reduced (non-cyclised) side-product 3.
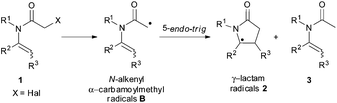 |
| Scheme 1 5-endo cyclisation of α-haloenamides through the formation of N-alkenyl α-carbamoylmethyl radicals. | |
With these considerations in mind we started our investigation with α-chloroenamide 1a as a model substrate. In the course of the optimisation study we first tested a series of amines as suitable stoichiometric reductive quenchers. At a concentration of 0.3 M we noticed the reaction to proceed with good reactivity, with the predominant formation of 3a after irradiation for 22 hours (Table 1, entries 1–3). Subsequently, we analysed the effect of more dilute concentrations on the product distribution, simultaneously increasing the reaction time from 22 to 40 hours. Low concentration mixtures (entries 5–7) encouraged the formation of the desired product 2a in good yields along with the regression of the direct reduction pathway. Surprisingly, increasing the catalyst loading from 1 to 2 mol% did not improve the yield of 2a, but rather enhanced the formation of side-product 3a (entry 8). A limit experiment under highly diluted conditions (0.01 M) with a prolonged reaction time (60 h) showed a general decrease in reactivity (entry 9). Solvent evaluation revealed good reactivity in DMSO (entry 12) and a drastic drop of yield in DMF (entry 10) or apolar solvents like toluene (entry 11).
Table 1 Optimisation of reaction conditionsa
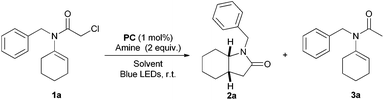
|
Entry |
Amine |
Solvent |
[mmol ml−1] |
2 [%] |
3 [%] |
Reaction conditions: α-chloroenamide 1a (0.2 mmol), amine (0.4 mmol), PC = Ir(ppy)2(dtb-bpy)PF6 (1 mol%), degassed solvent, blue LEDs (450 nm, 11 W), r.t., 22 h, NMR-yield (internal standard: mesitylene); the yield of the isolated product in parenthesis.
40 h reaction time.
48 h reaction time.
2 mol% PC.
60 h reaction time.
α-Chloroenamide 1a (0.13 mmol).
|
1 |
Et3N |
MeCN |
0.3 |
19 |
43 |
2 |
n-Bu3N |
MeCN |
0.3 |
20 |
39 |
3 |
i-PrN(Et)2 |
MeCN |
0.3 |
20 |
41 |
4b |
Et3N |
MeCN |
0.06 |
38 |
n.d. |
5b |
n-Bu3N |
MeCN |
0.06 |
41 |
n.d. |
6b |
n-Bu3N |
MeCN |
0.03 |
48 |
25 |
7c |
n-Bu3N |
MeCN |
0.02 |
592 (48) |
9 |
8b,d |
n-Bu3N |
MeCN |
0.02 |
40 |
11 |
9e |
n-Bu3N |
MeCN |
0.01 |
42 |
7 |
10c,f |
n-Bu3N |
DMF |
0.02 |
11 |
14 |
11c,f |
n-Bu3N |
Toluene |
0.02 |
13 |
5 |
12c,f |
n-Bu3N |
DMSO |
0.02 |
69 (67) |
17 |
As part of the optimisation study, we investigated the role of different halogens in the mesolysis of the C–X bond and in the following cyclisation step (Table 2). As previously reported by Ishibashi15 and Curran,16 the nature of the radical precursor has an effect on the product distribution, and hence we assumed that the analogous mechanism using different catalytic systems could lead to the formation of the same radical intermediates (Scheme 4).17
Table 2 Product distribution as a function of the radical precursora
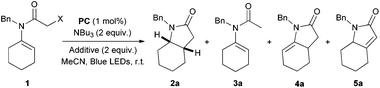
|
Entry |
X |
Additive |
2a [%] |
3a [%] |
4a [%] |
5a [%] |
Reaction conditions: α-haloenamide 1 (0.13 mmol), amine (0.26 mmol), PC (1 mol%), degassed DMSO, blue LEDs (450 nm, 11 W), r.t., 48 h.
|
1 |
Cl |
— |
69 |
17 |
— |
— |
2 |
Br |
— |
54 |
17 |
24 |
n.d. |
3 |
Cl |
NaI |
<5 |
31 |
17 |
22 |
When α-bromoenamide 1a′ was tested under the optimised reaction conditions, a mixture of the expected cyclised product 2a along with the directly reduced side product 3a was obtained, accompanied by 24% of the cyclised-oxidative product 4a (entry 2).
When sodium iodide (2 equiv.) was added to the reaction mixture in order to in situ generate the iodide precursor 1a′′, the reaction provided only traces of the desired product 2a, while a mixture of 3a (major product in 38% yield) and cyclised-oxidative products 4a and 5a was obtained. Taking note of the role of radical precursors in the product distribution, we began the synthesis of various α-chloroenamides in order to investigate the electronic and steric effects on the cyclisation reaction (Scheme 2).
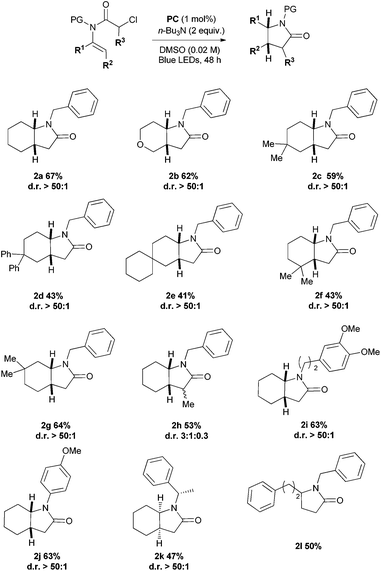 |
| Scheme 2 Scope for the photoredox catalysed 5-endo-trig cyclisation of substituted α-chloroenamides. Reaction conditions: α-chloroenamide 1 (0.13 mmol), n-Bu3N (2 equiv.), PC (1 mol%), degassed DMSO, blue LEDs (450 nm, 11 W), r.t., 48 h; yield of the isolated product. | |
Although the 5-endo-trig pathway is considered disfavoured,18 the reaction generally proceeded in moderate to good yields with a broad range of substrates with different substitution patterns. Remarkably, in almost all cases, only one diasteroisomer was formed.19 The product was obtained in good yield when a heteroatom was introduced as part of the cyclohexane skeleton (1b), but slightly decreased when the substituents were placed in the 4,4′ positions (1c–e); in this particular case, the yield decreased along with the increasing bulkiness (2c, 2d and 2e). It is noteworthy to underline that when two methyl groups were placed in the 5,5′ position (1g) the reaction gave a comparable yield (2g) to the non-substituted one (2a). When the same pattern of substituents was arranged directly next to the olefin bond on the 3,3′ carbon atom (1f), the formation of product 2f dropped to 43% yield. This trend was also observed when a methyl group was placed alpha to the incoming radical (2h). Good yields were observed when different substituents were placed on the nitrogen atom (2i and 2j). Next, we wondered how the diastereoselectivity would be influenced by placing the chiral auxiliary (S)-1-phenethyl group as a removable protecting group in this position (2k).20 Although the yield was modest, only one diastereoisomer was formed (Scheme 2). The cleavage of the phenethyl group upon treatment with Na/NH3 in THF at −78 °C20 led to the optically pure lactam 6. The absolute configuration of lactam 6 was assigned as 3aR,7aR by comparison with the literature reported compound.20,21 The auxiliary mediated asymmetric induction furnishes a smooth access to the basic structural core of the angiotensin converting enzyme (ACE) inhibitor HOE 498 (Scheme 3).22 Furthermore, we also observed comparable reactivity for the opened-chain system giving 50% yield (2l).
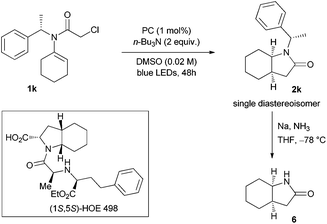 |
| Scheme 3 Influence of the chiral auxiliary (S)-1-phenethyl group on the asymmetric induction. | |
Regarding the mechanism, we assume that irradiation with visible light triggers the photoredox catalytic cycle of Ir3+, whose stable and long-lived (557 ns for Ir(ppy)2(dtbbpy)+)7j excited state Ir3+ acts as an oxidant towards trialkylamines (Ered1/2 = +1.0 V vs. SCE for triethylamine),23 generating the aminium radical cation A+˙ and Ir2+, which can reduce the α-chloroenamide 1 to the α-carbonyl radical B (Scheme 4). The latter species can then partition between the directly reduced product 3 and the 5-endo cyclised product 2.
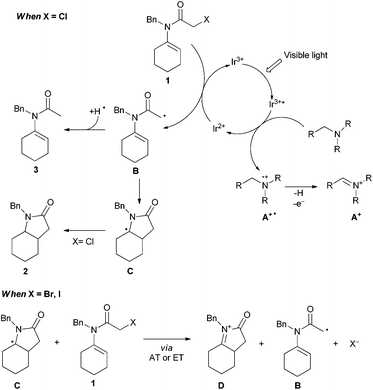 |
| Scheme 4 Postulated mechanism. | |
However, the ratio between the cyclised product 2 and the directly reduced product 3 depends on the ability of the radical precursor 1 to react with C, either by direct electron transfer (ET) or by halogen atom transfer (AT), to give an acyliminium ion that leads to D after deprotonation. This pathway is particularly favoured for iodo precursors, since they can compete with the hydrogen donor A+˙, while chlorides cannot. For the iodides it was further reported that the high amount of the directly reduced product 3 can be attributed to the formation of HI during the reaction, which, if not consumed fast enough by an acid/base reaction with the stannane or silane (n-Bu3N in our case), might react ionically with 1 to form 3 and I2.
In conclusion, we developed a mild and tin-free method for the preparation of synthetically valuable γ-lactams24 in good yields and high diastereomeric ratios, using visible light photoredox catalysis. Therewith, we provide the first example of visible light mediated 5-endo-trig cyclisation of α-chloroenamides that, in spite of its synthetic utility, still remains a challenging transformation. Furthermore, the diastereoselective reaction resulted in the formation of a single diastereoisomer, which is of interest for the synthesis of enantiomerically enriched products. The use of α-chloroenamides for the generation of monocyclic γ-lactams is particularly appealing and further application of this photoredox catalysis methodology is currently under investigation.
Acknowledgements
The research leading to these results has received funding from the European Research Council under the European Union's Seventh Framework Programme (FP/2007-2013)/ERC Grant Agreement No. 617044 (SunCatChem). Financial support by KAUST is gratefully acknowledged.
Notes and references
- For selected reviews and books on SET processes, see:
(a)
V. E. Balzani, Electron Transfer in Chemistry, Wiley-VCH, Weinheim, 2001 Search PubMed;
(b)
P. Renaud and M. P. Sibi, Radicals in Organic Synthesis, Wiley-VCH, Weiheim, 2001 Search PubMed;
(c)
P. S. Mariano, Advances in Electron Transfer Chemistry, JAI Press-Elsevier, Hampton Hill, Middlesex, England, 1994 Search PubMed;
(d)
L. Eberson, Electron Transfer Reactions in Organic Chemistry, Springer-Verlag, Berlin, 1987 Search PubMed;
(e)
R. D. Cannon, Electron Transfer Reactions, Butterworths, London, 1980 Search PubMed;
(f) A. Houmam, Chem. Rev., 2008, 108, 2180 CrossRef CAS PubMed;
(g) D. R. Weinberg, C. J. Gagliardi, J. F. Hull, C. F. Murphy, C. A. Kent, B. C. Westlake, A. Paul, D. H. Ess, D. G. McCafferty and T. J. Meyer, Chem. Rev., 2012, 112, 4016 CrossRef CAS PubMed;
(h) N. Zhang, S. R. Samanta, B. M. Rosen and V. Percec, Chem. Rev., 2014, 114, 5848 CrossRef CAS PubMed.
- For overviews of free radical initiators applied in natural product synthesis, see:
(a) X. J. Salom-Roig, F. Dénès and P. Renaud, Synthesis, 2004, 1903 CAS;
(b) G. J. Rowlands, Tetrahedron, 2009, 65, 8603 CrossRef CAS;
(c) K. C. Nicolaou, S. P. Ellery and J. S. Chen, Angew. Chem., Int. Ed., 2009, 48, 7140 CrossRef CAS PubMed;
(d) G. J. Rowlands, Tetrahedron, 2010, 66, 1593 CrossRef CAS;
(e)
J. Lalevée and J. P. Fouassier, in Encyclopedia of Radicals in Chemistry, Biology and Materials, ed. C. Chatgilialoglu and A. Studer, John Wiley & Sons, Chichester, UK, 2012 Search PubMed.
- Selected examples for the use of alkyl stannanes as hydrogen atom donors:
(a) D. H. R. Barton and S. W. McCombie, J. Chem. Soc., Perkin Trans. 1, 1975, 1574 RSC;
(b) D. H. R. Barton, D. Crich and W. B. Motherwell, J. Chem. Soc., Chem. Commun., 1983, 939 RSC;
(c) P. Renaud and S. Schubert, Angew. Chem., Int. Ed., 1990, 29, 433 CrossRef;
(d) D. P. Curran and C. M. Seong, J. Am. Chem. Soc., 1990, 112, 9401 CrossRef CAS; For the use of boranes as hydrogen atom donors, see:
(e) D. H. R. Barton and M. Jacob, Tetrahedron Lett., 1998, 39, 1331 CrossRef CAS;
(f) M. Lucarini, G. F. Pedulli and L. Valgimigli, J. Org. Chem., 1996, 61, 1161 CrossRef CAS;
(g) J. A. Baban and B. P. Roberts, J. Chem. Soc., Perkin Trans. 2, 1988, 1195 RSC;
(h) B. P. Roberts, Chem. Soc. Rev., 1999, 28, 25 RSC.
- For the use of N-heterocyclic carbene–borane complexes (NHC–BH3) as hydrogen atom donors, see:
(a) S.-H. Ueng, M. M. Brahmi, E. Derat, L. Fensterbank, E. Lacôte, M. Malacria and D. P. Curran, J. Am. Chem. Soc., 2008, 130, 10082 CrossRef CAS PubMed;
(b) S.-H. Ueng, A. Soloyev, X. Yuan, S. J. Geib, L. Fensterbank, E. Lacôte, M. Malacria, M. Newcomb, J. C. Walton and D. P. Curran, J. Am. Chem. Soc., 2009, 131, 11256 CrossRef CAS PubMed;
(c) Q. Chu, M. M. Brahmi, A. Soloyev, S.-H. Ueng, D. P. Curran, M. Malacria, L. Fensterbank and E. Lacôte, Chem. – Eur. J., 2009, 15, 12937 CrossRef CAS PubMed.
- Selected examples of alkyl boranes as initiators:
(a) H. Yorimitsu, T. Nakamura, H. Shinokubo and K. Oshima, J. Org. Chem., 1998, 63, 8604 CrossRef CAS;
(b) H. Yorimitsu, T. Nakamura, H. Shinokubo, K. Oshima, K. Omoto and H. Fujimoto, J. Am. Chem. Soc., 2000, 122, 11041 CrossRef CAS;
(c) H. Yorimitsu, H. Shinokubo, S. Matsubara, K. Oshima, K. Omoto and H. Fujimoto, J. Org. Chem., 2001, 66, 7776 CrossRef CAS PubMed;
(d) D. A. Spiegel, K. B. Wiberg, L. N. Schacherer, M. R. Medeiros and J. L. Wood, J. Am. Chem. Soc., 2005, 127, 12513 CrossRef CAS PubMed;
(e) B. Wyler, F. Brucelle and P. Renaud, Org. Lett., 2016, 18, 1370 CrossRef CAS PubMed.
- For reviews on thin-free radical reactions, see:
(a) P. A. Baguley and J. C. Walton, Angew. Chem., Int. Ed., 1998, 37, 3072 CrossRef CAS;
(b) A. Studer and S. Amrein, Synthesis, 2002, 835 CrossRef CAS.
- Selected review articles on photoredox catalysis:
(a) K. Zeitler, Angew. Chem., Int. Ed., 2009, 48, 9785 CrossRef CAS PubMed;
(b) T. P. Yoon, M. A. Ischay and J. Du, Nat. Chem., 2010, 2, 527 CrossRef CAS PubMed;
(c) J. M. R. Narayanam and C. R. J. Stephenson, Chem. Soc. Rev., 2011, 40, 102 RSC;
(d) F. Teply, Collect. Czech. Chem. Commun., 2011, 76, 859 CrossRef CAS;
(e) J. W. Tucker and C. R. J. Stephenson, J. Org. Chem., 2012, 77, 1617 CrossRef CAS PubMed;
(f) J. Xuan and W. Xiao, Angew. Chem., Int. Ed., 2012, 51, 6828 CrossRef CAS PubMed;
(g) M. A. Ischay and T. P. Yoon, Eur. J. Org. Chem., 2012, 3359 CrossRef CAS;
(h) L. Shi and W.-J. Xia, Chem. Soc. Rev., 2012, 41, 7687 RSC;
(i) D. Ravelli and M. Fagnoni, ChemCatChem, 2012, 4, 169 CrossRef CAS;
(j) C. K. Prier, D. A. Rankic and D. W. C. MacMillan, Chem. Rev., 2013, 113, 5322 CrossRef CAS PubMed;
(k)
M. Rueping, R. M. Koenigs and I. Atodiresei, in Chemical Photocatalysis, ed. B. Koenig, de Gruyter, Berlin, 2013, p. 169 Search PubMed;
(l) J.-R. Chen, X.-Q. Hu, L.-Q. Lu and W.-J. Xiao, Chem. Soc. Rev., 2016, 45, 2044 RSC.
- For selected recent examples of photoredox catalysed reactions from our group, see:
(a) S. Zhu, A. Das, L. Bui, H. Zhou, D. P. Curran and M. Rueping, J. Am. Chem. Soc., 2013, 135, 1823 CrossRef CAS PubMed;
(b) M. Rueping and C. Vila, Org. Lett., 2013, 15, 2092 CrossRef CAS PubMed;
(c) H. Hou, S. Zhu, F. Pan and M. Rueping, Org. Lett., 2014, 16, 2872 CrossRef CAS PubMed;
(d) D. C. Fabry, J. Zoller, S. Raja and M. Rueping, Angew. Chem., Int. Ed., 2014, 53, 10228 CrossRef CAS PubMed;
(e) J. Zoller, D. Fabry, M. Ronge and M. Rueping, Angew. Chem., Int. Ed., 2014, 53, 13264 CrossRef CAS PubMed;
(f) D. C. Fabry, M. A. Ronge and M. Rueping, Chem. – Eur. J., 2015, 21, 5350 CrossRef CAS PubMed;
(g) D. C. Fabry, M. A. Ronge, J. Zoller and M. Rueping, Angew. Chem., Int. Ed., 2015, 54, 2801 CrossRef CAS PubMed;
(h) M. Nakajima, E. Fava, S. Loescher, Z. Jiang and M. Rueping, Angew. Chem., Int. Ed., 2015, 54, 8828 CrossRef CAS PubMed;
(i) Q. Lefebvre, N. Hoffmann and M. Rueping, Chem. Commun., 2016, 52, 2493 RSC;
(j) E. Fava, A. Millet, M. Nakajima, S. Loescher and M. Rueping, Angew. Chem., Int. Ed., 2016, 55, 6776 CrossRef CAS PubMed;
(k) A. Millet, Q. Lefebvre and M. Rueping, Chem. – Eur. J., 2016, 22 DOI:10.1002/chem.201602257.
- For selected examples of photoredox C–Hal reduction, see:
(a) K. Hironaka, S. Fukuzumi and T. J. Tanaka, J. Chem. Soc., Perkin Trans. 2, 1984, 1705 RSC;
(b) D. A. Nicewicz and D. W. C. MacMillan, Science, 2008, 322, 77 CrossRef CAS PubMed;
(c) J. W. Tucker, J. D. Nguyen, J. M. R. Narayanam, S. W. Krabbe and C. R. J. Stephenson, Chem. Commun., 2010, 46, 4985 RSC;
(d) J. W. Tucker and C. R. J. Stephenson, Org. Lett., 2011, 13, 5468 CrossRef CAS PubMed;
(e) J. D. Nguyen, E. M. D'Amato, J. M. R. Narayanam and C. R. J. Stephenson, Nat. Chem., 2012, 4, 854 CrossRef CAS PubMed;
(f) H. Kim and C. Lee, Angew. Chem., Int. Ed., 2012, 51, 12303 CrossRef CAS PubMed;
(g) G. Pratsch and L. E. Overman, J. Org. Chem., 2015, 80, 11388 CrossRef CAS PubMed.
- Only a few examples involving C–Cl mesolysis have so far been reported; for recent examples of photoredox reduction of aryl chlorides, see:
(a) I. Ghosh, T. Ghosh, J. I. Bardagi and B. König, Science, 2014, 346, 725 CrossRef CAS PubMed. Examples of photoredox reduction of C(sp3)–Cl for radical addition, see:
(b) J. M. R. Narayanam, J. W. Tucker and C. R. J. Stephenson, J. Am. Chem. Soc., 2009, 131, 8756 CrossRef CAS PubMed;
(c) M. Pirtsch, S. Paria, T. Matsuno, H. Isobe and O. Reiser, Chem. – Eur. J., 2012, 18, 7336 CrossRef CAS PubMed;
(d) C.-J. Wallentin, J. D. Nguyen, P. Finkbeiner and C. R. J. Stephenson, J. Am. Chem. Soc., 2012, 134, 8875 CrossRef CAS PubMed;
(e) M. Nakajima, Q. Lefebvre and M. Rueping, Chem. Commun., 2014, 50, 3619 RSC.
-
J. Fossey, D. Lefort and J. Sorba, Free Radicals in Organic Chemistry, John Wiley & Sons, New York, 1995 Search PubMed.
- H. Ishibashi, N. Nakamura, T. Sato, M. Takeuchi and M. Ikeda, Tetrahedron Lett., 1991, 32, 1725 CrossRef CAS.
- Selected examples:
(a) H. Ishibashi, T. Sato and M. Ikeda, Synthesis, 2002, 695 CrossRef CAS;
(b) I. V. Alabugin and M. Manoharan, J. Am. Chem. Soc., 2005, 127, 9534 CrossRef CAS PubMed;
(c) C. Chatgilialoglu, C. Ferreri, M. Guerra, V. Timokhin, G. Froudakis and T. Gimisis, J. Am. Chem. Soc., 2002, 124, 10765 CrossRef CAS PubMed;
(d) H. Fuwa and M. Sasaki, J. Org. Chem., 2009, 74, 212 CrossRef CAS PubMed.
- Cyclisation of α-haloenamides:
(a) M. A. Guerrero, R. Cruz-Almanza and L. D. Miranda, Tetrahedron, 2003, 59, 4953 CrossRef CAS;
(b) L. D. Miranda and S. Z. Zard, Org. Lett., 2002, 4, 1135 CrossRef CAS PubMed;
(c) T. Sato, N. Nakamura, K. Ikeda, M. Okada, H. Ishibashi and M. Ikeda, J. Chem. Soc., Perkin Trans. 1, 1992, 2399–2407 RSC.
- O. Tamura, H. Matsukida, A. Toyao, Y. Takeda and H. Ishibashi, J. Org. Chem., 2002, 67, 5537 CrossRef CAS PubMed.
-
(a) D. P. Curran, D. B. Guthrie and S. Geib, J. Am. Chem. Soc., 2008, 130, 8437 CrossRef CAS PubMed;
(b) D. B. Guthrie, K. Damodaran, D. P. Curran, P. Wilson and A. J. Clark, J. Org. Chem., 2009, 74, 4262 CrossRef CAS PubMed;
(c) A. J. Clark, D. P. Curran, J. V. Geden, N. James and P. Wilson, J. Org. Chem., 2011, 76, 4546 CrossRef CAS PubMed.
- Reaction conditions utilised: syringe pump addition of Bu3SnH (1.2 equiv.) to α-haloenamides at reflux in toluene.
- J. E. Baldwin, J. Chem. Soc., Chem. Commun., 1976, 734 RSC.
-
(a)
D. J. Hart, in Radicals in Organic Synthesis, ed. P. Renaud and M. P. Sibi, Wiley-VCH, Weinheim, Germany, 2001, vol. 2, p. 279 Search PubMed;
(b)
D. P. Curran, N. A. Porter and B. Giese, Stereochemistry of Radical Reactions, VCH, Weinheim, Germany, 1996 Search PubMed;
(c) T. V. Rajan Babu, Acc. Chem. Res., 1991, 24, 139 CrossRef CAS.
- H. Ishibashi, Y. Fuke, T. Yamashita and M. Ikeda, Tetrahedron: Asymmetry, 1996, 7, 2531 CrossRef CAS.
- The optical rotation of compound 6 has been measured [α]RTD = −24 (c = 0.388, EtOH) and compared with that of the reported chiral compound.
- J. P. Bussien, J. Nussberger, M. Porchet, B. Waeber, H. R. Brunner, M. Perisic, M. J. Tansey, M. Bomm and P. Hajdu, Naunyn-Schmiedeberg's Arch. Pharmacol., 1985, 329, 63 CrossRef CAS PubMed.
- D. D. M. Wayner, J. J. Dannenberg and D. Griller, Chem. Phys. Lett., 1986, 131, 189 CrossRef CAS.
- For the importance of γ-lactam as a key structural feature in biologically active compounds, see:
(a)
Ł. Albrecht, A. Albrecht and T. Janecki, in Natural Lactones and Lactams, Wiley-VCH, Weinheim, 2013, p. 147 Search PubMed;
(b) A. A. Stierle, D. B. Stierle and B. Patacini, J. Nat. Prod., 2008, 71, 856 CrossRef CAS PubMed;
(c) H. J. Shin, T. S. Kim, H.-S. Lee, J. Y. Park, I.-K. Choi and H. J. Kwon, Phytochemistry, 2008, 69, 2363 CrossRef CAS PubMed;
(d) S. Samwel, J. O. Odalo, M. H. H. Nkunya, C. C. Joseph and N. A. Koorbanally, Phytochemistry, 2011, 72, 1826 CrossRef CAS PubMed.
Footnote |
† Electronic supplementary information (ESI) available. See DOI: 10.1039/c6gc01099g |
|
This journal is © The Royal Society of Chemistry 2016 |