DOI:
10.1039/C5AY02191J
(Paper)
Anal. Methods, 2016,
8, 197-204
A kinetic spectrofluorometric method, aided by chemometrics, for the analysis of sibutramine, indapamide and hydrochlorothiazide compounds found in weight-reducing tonic samples
Received
20th August 2015
, Accepted 28th October 2015
First published on 11th November 2015
Abstract
An uncomplicated and sensitive kinetic spectrofluorometric method was developed for the simultaneous analysis of sibutramine, indapamide, and hydrochlorothiazide commonly found in weight-reducing health foods. The mentioned compounds were oxidized by cerium(IV) ammonium sulfate dissolved in an acidic solution. The kinetic-fluorescence spectrum of the produced cerium(III) was recorded between 0 and 600 s reaction time at the excitation wavelength of 250 nm. It was found that the fluorescence intensity was proportional to the concentration of the compounds, and their linear ranges were 0.005–0.12, 0.03–1.44 and 0.01–0.20 μg mL−1; the corresponding detection limits were 1.6, 8.3 and 6.0 ng mL−1 for sibutramine, indapamide and hydrochlorothiazide, respectively. The measured three- and two-way data of the mixtures were processed by chemometrics (included methods: parallel factor analysis (PARAFAC), partial least squares (PLS), principal component regression (PCR), and radial basis function-artificial neural network (RBF-ANN)). The proposed method was applied for the simultaneous determination of the analytes in weight-reducing food samples, and the results were comparable with those from the reference HPLC method.
Introduction
It is generally well recognized, that in recent years, as the quality of life improved, so has obesity become an increasingly serious problem worldwide. Thus, overweight adults in the U.S. now exceed 60% of the population, and the rate is rapidly increasing among children and adolescents. The cost of obesity-related medical care has increased astronomically since 1987, and commonly this was accompanied by a loss of productivity and income.1 According to the World Health Organization (WHO), in 2005, more than 1 billion people worldwide were overweight (BMI (Body Mass Index) ≥ 25), and more than 300 million were obese (BMI ≥ 30).
Furthermore, mean BMI, weight increase and obesity are increasing worldwide because, in general, there have been changes in eating habits, which facilitate weight increase; also, there seems to be a growing tendency towards physical inactivity. The above observations are particularly unfortunate since obesity also increases the risk of coronary heart disease, stroke and type 2 diabetes, as well as breast, colon, and prostate cancers amongst other ailments.2 At present, it would appear that there are no effective pharmaceutical products, which will facilitate significant weight loss particularly in the short term. However, illegal chemical products are sometimes added to food or drinks with claims that these substances produce weight loss.3 Three such substances include sibutramine, indapamide and hydrochlorothiazide, and they form the subject of this paper.
Sibutramine (Table 1) is an appetite inhibitor, plays a major role in the central nervous system, and influences weight-loss by altering neurotransmitters within the brain. While 5–10% weight losses have been claimed by the consumers of sibutramine, this substance can cause serious side effects, and in general, it is a forbidden additive to the weight-reducing tonics. Currently, it has been withdrawn from the markets in most countries (http://www.drugbank.ca/drugs/DB01105). Indapamide and hydrochlorothiazide are diuretics (Table 1), which are generally used for treating hypertension. Both increase urination, and this promotes dehydration in the recipient. In addition, it is not uncommon to find that both the weight reducing compounds, e.g. sibutramine, and the diuretics, e.g. indapamide and hydrochlorothiazide, have been added to the weight-reducing tonics contrary to drug regulations. They were added so as to produce a tonic that reduces body weight faster. Therefore, simultaneous analysis of these substances in weight-reducing foods is clearly important.3,4
Table 1 Chemical structures of sibutramine, hydrochlorothiazide and indapamide and their reaction with cerium(IV)
Part A: Chemical structures |
Name |
Molecular formula |
Chemical structures |
Sibutramine |
C17H26CIN HCl H2O |
|
Hydrochlorothiazide |
C7H8ClN3O4S2 |
|
Indapamide |
C16H16ClN3O3S |
|
Part B: Oxidation reaction with Ce4+ in 0.04 mol L−1 H2SO4 medium |
Analyte + Ce4+ + H+ → Oxidation product + Ce3+ |
Several analytical methods for sibutramine, indapamide and hydrochlorothiazide have been described, e.g. spectrofluorometry,5,6 spectrophotometry,4,6,7 liquid chromatography4,8,9 and voltammetry.10 Zhou et al.5 applied a fluorescence quenching method for the analysis of sibutramine, which included a complex formation step with a halo-fluorescein dye; the linear range and limits of detection (LODs) were 0.1–4.0 μg mL−1 and 0.06 μg mL−1, respectively. Omar6 reported a sensitive spectro-fluorimetric method for the determination of three different diuretics in tablet form, and the obtained linear range and LOD for all these three compounds, indapamide, hydrochlorothiazide and xipamide, were 0.05–0.25 μg mL−1 and 0.013 μg mL−1, respectively. Recently, Teradal et al.10 described an electrochemical method for the determination of sibutramine with the use of an electro-reduced graphene oxide film modified glassy carbon electrode as the sensor; the linear range and the LOD were 0.083–6.60 μg mL−1 and 0.016 μg mL−1. In general, all these methods are rather insensitive, can be quite slow and often require quite sophisticated and dedicated instrumentation. On the other hand, the fluorescence technique normally offers relatively quite high sensitivities compared to the other methods. In addition, the use of a kinetic method coupled with the result interpretation with the use of chemometrics modelling has considerable advantages, such as the simplicity of the analytical method and the simultaneous determination of several analytes without the need for any chemical separation or masking steps.11–14 Also, importantly, it appears that this is the first study, which involves the use of a kinetic spectrofluorometric method for the simultaneous analysis of three analytes.
In recent years, multi-way and in particular, three-way complex data analyses have been developed, principally, in response to progress in hyphenated detection methods. Multi-component simultaneous determination by spectrophotometric differential kinetic procedures combined with spectral measurements is a simple but informative method to obtain three-way data, which also improves the accuracy of prediction for multi-component analysis.15,16 PARAFAC is a second-order method and its advantages have been widely utilized in recent years, both for qualitative17 and quantitative applications.18 This method facilitates the identification and quantification of the responses from the analytes of interest in the presence of unknown or uncalibrated interferences.19 The second-order condition of the PARAFAC method also affects the optimization procedure,16 because the extracted profile of the analyte of interest is also independent of the experimental conditions; thus, only one calibration curve is necessary for the determination of the concentration of all the experiments of the designed model. This strategy is useful for limiting the size of the experiment necessary for estimating the recovery of the analyte and establishing the robustness of an analytical procedure.
In this work, the aim was to develop a novel, indirect kinetic-spectrofluorometric method for the simultaneous analysis of sibutramine, indapamide and hydrochlorothiazide found in weight-reducing tonics. The results were to be interpreted by the best performing chemometrics method chosen from several alternatives: different chemometrics methods, such as parallel factor analysis (PARAFAC), principal component regression (PCR), partial least squares (PLS), radial basis function-artificial neural network (RBF-ANN), and principal component-radial basis function-artificial neural network (PC-RBF-ANN).
Methodology
Kinetic models
Consider that the nth analyte, Sn (n = 1, 2, …, N), reacts with a common reagent R in a sulfuric acid solution, to produce a product, Pn. If the concentration of R is much higher than that of Sn, the reaction can be assumed to follow the pseudo-first-order kinetics.20 Thus, the kinetic relationship involving the concentrations, Sn and Pn, and the fluorescence intensity caused by Pn, at time, t, can be represented by: | cSn = cSn,0 exp(−kSnt) (n = 1, 2, …, N) | (1) |
| cPn = cSn,0 [1 − exp(−kSnt)] | (2) |
| FPn = εPnbcPn = cSn,0εPnb[1 − exp(−kSnt)] = KSncSn,0 | (3) |
where cSn,0 is the initial concentration of the analyte Sn and kSn is its corresponding rate constant. cSn and cPn are the concentrations of Sn and Pn, respectively. FPn is the fluorescence intensity, which is associated with the concentrations, Pn, i.e., the concentration of the produced cerium(III) is equal to that of Pn, at a time, t, and εPn is its molar fluorescence coefficient. KSn is the proportional coefficient of the component, Sn, at a time, t, and b is the cell width.
For M standard mixed samples, the kinetic-fluorescence-spectral (three-way) data from this kinetic system, which is monitored at P wavelengths (p = 1, 2, …, P) and K time points (k = 1, 2, …, K), can be expressed in matrix form as:
If at a given wavelength, p, (this work: 355 nm), eqn (4) can be reduced to a two-way kinetic data matrix form,
then
eqn (4) and
(5) become typical three- and two-way spectro-fluorimetric datasets, respectively. Thus, it becomes possible to resolve the multi-component kinetic equations. This can be achieved with the use of suitable chemometrics models without knowing the reaction mechanisms in detail. In this work, such models were developed for the analytes: sibutramine, indapamide and hydrochlorothiazide.
Chemometrics methods
Chemometrics methods for the three-way data.
Parallel factor analysis (PARAFAC) is a multi-way method,21 which, in recent years, has found application in chemometrics because the method can process quite complex scientific data. Commonly, the data is presented in a three-way array format, and is decomposed into loading matrices, A, B and C, for the row-, column- and layer-items of the three-way stack, respectively. These three loading matrices with elements, amf (sample), bpf (emission wavelength), and ckf (time) produce a three-way array, (Fmpk), which effectively is a PARAFAC model for which the sum of squares of the residuals, empk, is minimized: | 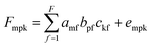 | (6) |
In this model, the three loading matrices, A, B and C, are not necessarily orthogonal,22 but the solution of the PARAFAC model is unique and does not suffer any indeterminate aspects, which may arise with principal components and factor analysis models. The number of factors in each model is identical and this number is chosen to be smaller than the original number of variables in the data matrix in order to achieve a considerable reduction. The elements of the loading matrices, A, B and C, are computed so as to minimize the sum of the squared residuals. PARAFAC is one of several decomposition methods for n-way data, and is less flexible. It uses fewer degrees of freedom and provides a unique solution independent of rotation.23 All of these features provide a great advantage for modeling of kinetic-spectrofluorometric data.
Chemometrics methods for two-way data.
Principal component regression (PCR) and partial least squares (PLS) are two well-known factor analysis, multivariate, statistical tools; they are similar in many ways and the theoretical relationship between them has been treated extensively in the literature.24 These two methods perform data decomposition into spectral scores and loadings prior to model building with the aid of these new variables. In PLS models, the data decomposition is carried out with the use of both spectral and concentration data, while the PCR models employ only spectral information. PLS models almost always require fewer latent variables than the PCR ones, but this does not appear to influence their predictive abilities.25
Radial basis function-artificial neural network (RBF-ANN) modeling has been often applied to resolve analytical problems, and it offers many advantages when dealing with the non-linearity inherent in such applications. Recently, the RBF-ANN model has been noted for its simple network structure that avoids lengthy calculations as compared to the back propagation (BP)-ANN models. It is quite robust, and its sensitivity improves in the presence of noisy data.26 PC-RBF-ANN is an improved RBF-ANN model, and it has been applied only occasionally.11
In this work, principal component analysis (PCA) was applied in the form of a pretreatment of the calibration data matrix, and the PC scores were then used as the input of the network. However, artificial neural network (ANN) models were designed to work on non-linear, multi-modal, and poorly understood, improperly or ill-posed problems (Kolmogorov continuity theorem).27
Among the data collection methods reported so far, derivative spectrophotometry28 is an advanced modern technique, which can lead to the separation of overlapped signals as well as the elimination of background caused by the presence of compounds other than the analyte(s) in the sample of interest.
Experimental
Chemicals and reagents
All chemicals were analytical grade reagents and were used without further purification. Doubly distilled water was used throughout. For spectro-fluorimetric measurements, the following solutions were prepared: 0.2 mol L−1 H2SO4 solution from commercial H2SO4 (Merck, Darmstadt, Germany); sibutramine, indapamide, and hydrochlorothiazide solutions (50 μg mL−1 each; Shanghai Sixth Pharmaceutical Factory, Shanghai, China) – an appropriate amount of each compound was dissolved in methanol in a 50 mL volume flask. A stock solution containing 0.01 mol L−1 Ce(IV) was prepared from solid cerium(IV) sulfate tetra-hydrate, which was dissolved in 0.02 mol L−1 H2SO4.
Instrumentation
All kinetic, fluorescence and other spectral data were obtained from measurements on a Perkin-Elmer LS-55 spectrofluorimeter (Perkin Elmer Co., MA, U.S.), which was equipped with a 1.0 cm quartz cell and a thermostated bath (Model ZC-10, Ningbo Tianheng Instruments Factory, China) to maintain the reaction temperature at 70 °C. The excitation and emission slits were all set at 10 nm, while the scanning rate was 1500 nm min−1. The emission spectra were recorded in the 250–450 nm range with an excitation wavelength of 250 nm.
The pH was monitored with a Model SA-720 pH meter (Orion Research Inc., Boston, MA, U.S.).
The food sample solutions were sonicated for about 5 min with the use of an ultrasonic bath (SK1200H, Kudos Ultrasonic Instrument Co. Ltd., Shanghai), and all solution volumes of less than 1.0 mL were delivered with micropipettes (Finnpipette-Labsystems, Helsinki, Finland).
The obtained data were processed with programs written in-house in MATLAB 6.5 (Mathworks, MA, U.S.).
HPLC measurements were performed on an Agilent 1100 series (Agilent Technologies, Palo Alto, CA, U.S.) equipped with a G1315B diode array detector, a G1379A vacuum degasser, quaternary pump solvent management system, a G1311A auto-sampler, and an injector with a 100 μL loop.
An Agilent Zorbax eclipse XDB-C18 column (4.6 mm × 250 mm, 5 μm) was used with an Agilent Zorbax high pressure reliance cartridge guard-column (C18, 4.6 mm × 12.5 mm, 5 μm), and a variable wavelength UV-vis detector set at 223 nm. All chromatograms were run at 20 ± 1 °C using a mixture of methanol–H2O–triethylamine (75
:
24
:
1/v/v/v) buffer solution. Other experimental parameters were: flow rate, 1.0 mL min−1; retention times, 6.10, 7.85 and 9.20 min for sibutramine, hydrochlorothiazide and indapamide, respectively.
Spectrofluorometric analytical procedure
Oxidation of the analytes was carried out directly in a spectro-fluorometer cell. A suitable volume of an analyte solution (<0.4 mL) was placed into a 1 cm cuvette, 0.5 mL of 0.2 mol L−1 H2SO4 and a suitable amount of water were then added to let the total volume equal to 2.4 mL. The system was stirred at a constant temperature (70 °C) throughout the reaction. For the reaction to occur, 0.1 mL of 0.01 mol L−1 Ce(IV) solution was added to the above mixture to give a total volume of 2.5 mL. The fluorescence of the reduced Ce(III) was measured at λex = 250 nm immediately as a function of time, and was recorded during 600 s at 2 s intervals (total 301 points) in the spectral range of 250–450 nm every 2 nm (total 101 wavelengths). Generally, it was found that during this time period, enough quantitative, kinetic reaction data could be collected. Thus, the kinetic data obtained from the fluorescence measurements, and the kinetic-spectral data could be collected and arranged into a three-way data matrix at λex = 250 nm. To avoid interference caused by the initial presence of Ce(III), all measurements were obtained against the blank solution treated in the same way but without sibutramine, indapamide, and hydrochlorothiazide. Also, the fluorescence readings of the blank were recorded as a function of time.
Preparation of weight-reducing tonic samples
The solid weight-reducing food samples (slimming chocolate, biscuits, candy and others) were ground to a fine powder, while the liquid weight-reducing food samples (diet coffee, milk tea, and others) were filtered through a Wohua #202 quantitative filter paper (Hangzhou, China). Subsequently, the fine powder (50.0 g) or the filtered liquid samples (50.0 mL each) were diluted with 150 mL methanol and were collected in 250 mL Erlenmeyer flasks. These samples were then shaken for 1.5 h. A suitable aliquot of each of these mixtures was subsequently transferred to 10 mL centrifuge tubes, and 2.0 mL of 2 mg mL−1 sodium 1-heptane-sulfonate solution were added to each tube so as to destroy any polyphenol and alkaline matter, which might have been present in the weight reducing tonic.6 The resulting mixture was then centrifuged at 4000 rpm for 10 min and the clear solution in the tube was collected. A suitable amount of this clear sample was transferred into a 25 mL flask, and 5.0 mL 95% methanol was then added. This final solution was analyzed by the procedure described above (section “Spectrofluorometric analytical procedure”).
HPLC procedure for the analysis of weight-reducing tonic samples
Each sample (20 μL of each solution from the above section) was injected and the concentration was calculated on the basis of the peak area ratios from the standard solutions. The linearity between the peak area and the concentration was confirmed in each concentration range. In general, for experimental conditions of this procedure consult the Instrumentation section. It is important to note that under the conditions described in this section, the peaks were well resolved.
Spectral and kinetic behaviour
The overall reaction involved the three compounds: sibutramine, indapamide, and hydrochlorothiazide, which reduced Ce(IV) in a sulfuric acid medium to produce a highly fluorescent Ce(III) cation. The resulting spectrum had a maximum fluorescence at 355 nm after excitation at 250 nm (inset: Fig. 1). The oxidation product did not fluoresce, and this confirmed that the fluorescence produced during the oxidation of the investigated analytes with Ce(IV), could not be attributed to its oxidation product but rather to the formation of Ce(III). The kinetic curves of the analytes obtained from the reactions with Ce(IV) under the selected conditions (Fig. 1), showed that the reaction rates of these three compounds were different. Thus, the rate constants for the respective reactions were estimated by fitting the kinetic data obtained from the samples containing one of the analytes at a time.
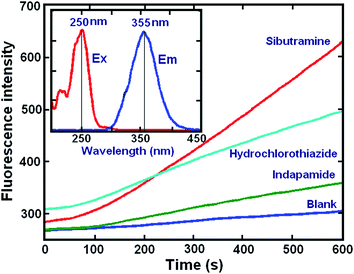 |
| Fig. 1 Plot of kinetic absorbance versus time recorded at λex = 250 nm and λem = 355 nm, for the analytes, sibutramine, hydrochlorothiazide and indapamide (0.08 μg mL−1 each), with a total reaction time of 600 s, T = 70 °C, cH2SO4 = 0.04 mol L−1, and cCe(IV) = 4.0 × 10−4 mol L−1. | |
The relevant rate equation applied was: A = a0 − a1
exp(−kt),29 and the rate constants for sibutramine, indapamide, and hydrochlorothiazide were 0.0138 ± 0.0003, 0.0092 ± 0.0005 and 0.0060 ± 0.0008, respectively. Even though the analytes showed differences in their reaction rates with Ce(IV), their kinetic profiles overlapped, and thus, it was necessary to use chemometrics methods to resolve this data (see Scheme 1).
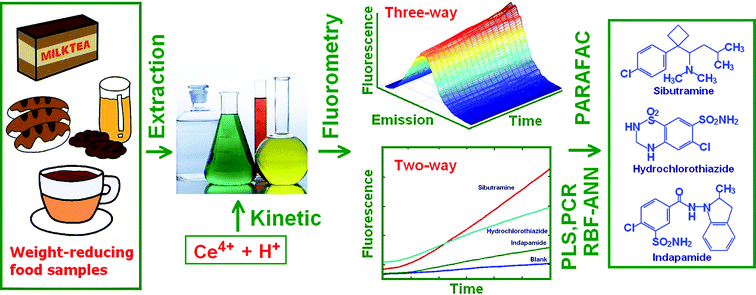 |
| Scheme 1 From samples to analysis of sibutramine, hydrochlorothiazide and indapamide in food samples. | |
Optimization of the reaction conditions
The kinetic behavior of most compounds in chemical reactions can be controlled by changing the microenvironment of the reaction. Thus, first the method operating spectral conditions for calibration and prediction were optimized and different concentrations of the Ce(IV) solution in the range of 1.0–6.0 × 10−4 mol L−1 were investigated. Higher concentrations were not considered because it was likely that at these levels the fluorescence inner filter effect could interfere. This could occur because Ce(IV) absorbs at 350 nm (λmax ∼ 320 nm),12i.e. the same wavelength at which Ce(III) emits. The results showed that the fluorescence intensity as measured against a blank sample, increased substantially with the increase in the concentration of Ce(IV) solution up to 4.0 × 10−4 mol L−1; however, at higher Ce(IV) concentrations the fluorescence decreased significantly. Thus, 4.0 × 10−4 mol L−1 Ce(IV) was chosen as the optimum concentration.
Different concentrations of H2SO4 solution (0.01–0.06 mol L−1) were also investigated, and it was found that the increase in acidity caused a decrease in the ΔF of the reaction system. Ultimately, 0.04 mol L−1 was selected for this work.
Experiments with the reaction temperature (40–75 °C), showed that the ΔF values increased with increasing temperature up to 70 °C; thereafter ΔF values remained unchanged. Thus, 70 °C was selected for subsequent work.
Calibration models for individual analytes
The fluorescence kinetic profiles of each compound, at different concentrations, were measured at λem = 355 nm after excitation at 250 nm (Fig. 2). Linear calibration equations and their relative parameters were established with the data recorded at 600 s. The parameters of the linear models for these data indicated that there was a good linear correlation between the fluorescence intensity (F) and concentration (c). The linear analytical ranges and the LODs were: sibutramine (0.005–0.12 μg mL−1, 1.6 ng mL−1), indapamide (0.03–1.44 μg mL−1, 8.3 ng mL−1) and hydrochlorothiazide (0.01–0.20 μg mL−1, 6.0 ng mL−1). It should be noted that the LODs were evaluated using the statistic, sy/x, which estimates the random errors in the y-direction of the measured data of a set of samples.30 These results compare well with or outperform results from similar previous studies.5,6,10 Thus, the proposed kinetic spectrofluorometric method is appropriate for the determination of the individual analytes.
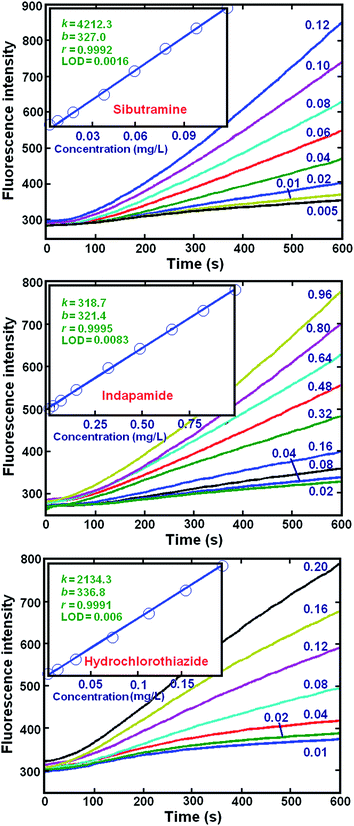 |
| Fig. 2 Kinetic curves obtained from sibutramine, hydrochlorothiazide and indapamide with different concentrations (mg L−1) at λem = 355 nm. Insets: k, b, r and LOD represent the slope (mL μg−1), intercept, correlation coefficient and the limit of detection (μg mL−1) of the calibration plots, respectively. They were calculated according to Miller's method.30 Experimental conditions are as in Fig. 1. | |
Simultaneous determination of the three analytes in synthetic mixtures
A calibration set, consisting of a total of sixteen solutions with four concentration levels, was constructed according to the orthogonal array design denoted by OA16(44).31 It was prepared so as to extract the maximum quantitative information about the samples with the use of a minimum number of experimental trials. The concentration levels for the three analytes were: sibutramine (0.008–0.8 μg mL−1), indapamide (0.06–0.8 μg mL−1), and hydrochlorothiazide (0.01–0.12 μg mL−1). These levels were selected to allow for a wide distribution of concentrations, which would also cover the range of levels found in some food samples. The sixteen samples were prepared for analysis by the experimental procedure (section “Spectrofluorometric analytical procedure”); the spectrofluorometric-kinetic three-way data matrix, F (16 × 301 × 101), and the two-way data matrix, F (16 × 301) (fixed λem = 355 nm), were then constructed from the measurements with respect to the concentration matrix, C (16 × 3). The PARAFAC method was then applied to the F (16 × 301 × 101) matrix in order to establish the three-way calibration models; other methods such as PLS, PCR, RBF-ANN, and PC-RBF-ANN were used separately to process the two-way data matrix, F (16 × 301), so as to obtain the different calibration models in which the dimensions of the datasets were reduced significantly. The resulting five established calibration models were further verified by predicting the concentration of the three analytes in an unknown set of mixtures. The relative prediction errors (both RPES and RPET)32 for all of these cases were obtained from each method (Table 2). Also, it should be noted that the PARAFAC method is based on the tri-linear decomposition modelling and it uses the signal matrix without data unfolding. Thus, this method reflects the raw three-way data structure. In addition, the two-way kinetic data were obtained at λem = 355 nm and λex = 250 nm, and hence, two-way calibration models were built for the PLS, PCR, RBF-ANN and PC-RBF-ANN methods.
Table 2 Prediction results for sibutramine, indapamide and hydrochlorothiazide in mixtures with the use of different chemometrics methods
Methods |
%RPES |
%RPET |
Indapamide |
Hydrochlorothiazide |
Sibutramine |
Values in brackets correspond to the number of factors used in PCR and PLS models.
Data pretreatment with normalization. The parameter values in brackets are: the nodes in the hidden layer and the spread coefficient (sc), respectively.
Data pretreatment: as above in (b), and then the resulting matrix was submitted to PCA. The extracted scores were used as data for the neural network training. The parameter values in brackets: the number of factors used in PCA, nodes in the hidden layer and the spread coefficient (sc), respectively.
Values in brackets correspond to the mean recoveries (%).
|
Three-way kinetic-spectral data
|
PARAFAC |
8.5 (104.2)d |
6.1 (105.1) |
9.2 (98.4) |
7.8 |
![[thin space (1/6-em)]](https://www.rsc.org/images/entities/char_2009.gif) |
Kinetic data without any pretreatment
|
PLS (4)a |
10.7 (103.5) |
6.2 (105.5) |
12.8 (88.4) |
8.9 |
PCR (6)a |
11.1 (105.7) |
8.1 (102.2) |
13.5 (92.7) |
9.1 |
RBF-ANN (5, 400)b |
14.7 (96.7) |
11.2 (97.3) |
16.5 (97.4) |
13.4 |
PC-RBF-ANN (4, 5, 800)c |
12.5 (97.2) |
9.3 (98.6) |
14.6 (98.8) |
11.2 |
![[thin space (1/6-em)]](https://www.rsc.org/images/entities/char_2009.gif) |
Kinetic data with first-derivative pretreatment
|
PLS (5)a |
10.1 (104.2) |
6.0 (109.1) |
11.3 (90.8) |
8.5 |
PCR (6)a |
10.2 (106.8) |
7.8 (104.2) |
11.8 (94.5) |
8.8 |
RBF-ANN (4, 600)b |
13.5 (94.6) |
10.4 (96.5) |
15.0 (96.4) |
11.3 |
PC-RBF-ANN (4, 6, 700)c |
11.0 (95.7) |
8.5 (97.0) |
13.4 (97.6) |
10.3 |
The performances of the chemometrics prediction models (Table 2) indicated that the PARAFAC one, which can be used for resolving the three-way kinetic-spectral data, gave better results than the four models used with the two-way kinetic data. However, it should be noted that the PLS model performed somewhat better than the other three used with the two-way data. Also, when the two-way kinetic data matrices were converted with the use of the 1st-derivate as a pre-treatment step, and this new data were submitted to the chemometrics models, it was found that all of the methods produced improved prediction errors, i.e. RPES and RPET (see Table 2). In addition, it was found that the PARAFAC model produced the best prediction results. Such an outcome may be attributed to the use of the combined, raw kinetic-spectral data, which contained much more information than either the kinetic or spectral datasets alone. This outcome is consistent with the literature.19,24
Method selectivity
The selectivity of the proposed method was investigated and the effects of various substances that could be in different foods together with the sibutramine, indapamide, and hydrochlorothiazide analytes were analysed. The PARAFAC model was used for prediction. The tolerance limit was defined as the concentration of the added substance, which produced an RPET value of less than ±10%. The tolerance ratios for the determination of the improved performance were: Na+, K+ and Ca2+ (500
:
1); glucose, sucrose and fructose (250
:
1); Zn2+, Mn2+, Pb2+, Cu2+ and Fe3+ (200
:
1); lactic acid, citric acid and protein (150
:
1); sunset yellow, indigo blue and brilliant blue (100
:
1).
Determination of the three analytes in weight-reducing tonic samples
The proposed method for the simultaneous determination of the three analytes with the use of the PARAFAC method was applied for the analysis of a weight-reducing tonic. The results (Table 3) showed that the solid diet food, mainly contained sibutramine, i.e. the appetite inhibitor, whereas the liquid diet food contained sibutramine, indapamide, and hydrochlorothiazide, i.e. both the appetite inhibitor and the diuretics. Importantly, the analytical results produced with the novel spectrofluorometric method compared well with those from the reference HPLC method. The relative standard errors from the proposed method and HPLC were below 10%, which is a result that supports the reliability of the spectrofluorometric method.
Table 3 Determination of indapamide, hydrochlorothiazide and sibutramine in weight loss food samples with the use of the PARAFAC method
Samplesa |
Found (μg g−1) |
Added (μg g−1) |
Foundb (μg g−1) |
Recoveryc (%) |
All the food samples were purchased from the local markets in Nanchang city, China.
Found after standard additions.
Recovery (%) = 100 × [(cFound-Added − cFound)/cAdded] (brackets: HPLC values).
Mean of three replicates ± standard deviation (brackets: HPLC values).
ND: not detected.
|
Weight loss chocolate
|
Sibutramine |
452.1 ± 0.1 (452.4 ± 0.2)d |
10.0 |
461.6 ± 0.1 (462.0 ± 0.1) |
95 (96) |
Hydrochlorothiazide |
NDe |
5.0 |
4.8 ± 0.1 (4.9 ± 0.2) |
96 (98) |
Indapamide |
ND |
5.0 |
4.9 ± 0.1 (5.0 ± 0.1) |
98 (100) |
![[thin space (1/6-em)]](https://www.rsc.org/images/entities/char_2009.gif) |
Weight loss biscuits
|
Sibutramine |
316.2 ± 0.1 (317.4 ± 0.1) |
10.0 |
327.0 ± 0.2 (326.8 ± 0.1) |
108 (106) |
Hydrochlorothiazide |
ND |
5.0 |
4.8 ± 0.1 (4.9 ± 0.1) |
96 (98) |
Indapamide |
ND |
5.0 |
4.9 ± 0.1 (5.1 ± 0.2) |
98 (102) |
![[thin space (1/6-em)]](https://www.rsc.org/images/entities/char_2009.gif) |
Diet coffee
|
Sibutramine |
282.0 ± 0.1 (284.3 ± 0.1) |
10.0 |
392.9 ± 0.1 (394.0 ± 0.2) |
109 (110) |
Hydrochlorothiazide |
255.7 ± 0.2 (257.3 ± 0.2) |
5.0 |
260.4 ± 0.2 (262.1 ± 0.1) |
94 (96) |
Indapamide |
ND |
5.0 |
5.1 ± 0.1 (5.2 ± 0.1) |
102 (104) |
![[thin space (1/6-em)]](https://www.rsc.org/images/entities/char_2009.gif) |
Slimming milk tea
|
Sibutramine |
ND |
10.0 |
10.6 ± 0.2 (10.4 ± 0.1) |
106 (104) |
Hydrochlorothiazide |
ND |
5.0 |
4.8 ± 0.1 (4.9 ± 0.1) |
96 (98) |
Indapamide |
302.3 ± 0.1(303.6 ± 0.1) |
5.0 |
307.1 ± 0.2 (308.7 ± 0.1) |
96 (102) |
Conclusions
An uncomplicated and sensitive spectrofluorometric method for the simultaneous determination of sibutramine, indapamide, and hydrochlorothiazide was researched and developed. Chemometrics methods were applied to extract the results from the spectroscopic data. Importantly, the literature indicates that this is the first time that such a kinetic-spectrofluorometric method was researched, developed and applied for the quantitative, simultaneous analysis of ternary mixtures. This novel method is based on the oxidation of the analytes by cerium(IV) dissolved in a sulfuric acid solution and the accompanying, emitted fluorescence was monitored at λex = 250 nm. The spectroscopic results were interpreted with the use of the PARAFAC method, which was found to be the best performing chemometrics method for the simultaneous prediction of the three analytes. When this novel method was applied for the analysis of commercial weight-reducing samples, the results were satisfactory, and were within 3–4% of those obtained with the HPLC reference method. Importantly, this study provides a novel, uncomplicated method for the simultaneous analysis of sibutramine, indapamide, and hydrochlorothiazide in weight-reducing tonic samples and thus, facilitates the control of drug abuse in such commercial samples.
Acknowledgements
The authors greatly appreciate the financial support from the State Key Laboratory of Food Science and Technology of Nanchang University, China (SKLF-ZZA201302 and SKLF-ZZB201303).
References
- S. B. Wyatt, K. P. Winters and P. M. Dubbert, Am. J. Med. Sci., 2006, 331, 166–174 CrossRef PubMed.
-
World Health Organization, Global health risks: mortality and burden of disease attributable to selected major risks, WHO Press, Switzerland, 2009 Search PubMed.
- O. Zaporozhets, I. Tsyrulneva and M. Ischenko, Am. J. Anal. Chem., 2012, 3, 320–327 CrossRef CAS.
- G. Kilicarslan, E. Imamoglu, A. Kucuk and A. Ozdemir, Rev. Anal. Chem., 2010, 29, 169–195 CAS.
- S. Zhou and J. D. Yang, Chin. J. Anal. Lab., 2010, 29, 56–59 CAS (in Chinese).
- M. A. Omar, J. Fluoresc., 2010, 20, 275–281 CrossRef CAS PubMed.
- D. F. Maluf, P. V. Farago, S. M. W. Barreira, C. F. Pedroso and R. Pontarolo, Lat. Am. J. Pharm., 2007, 26, 909–912 CAS.
- I. C. F. Diefenbach, M. Friedrich, M. R. Dos Santos and C. F. Bittencourt, J. AOAC Int., 2009, 92, 148–151 CAS.
- W. Ma, L. Cheng, L. W. Zhang, Y. C. Zhang, H. B. Wang, Y. H. Jiao, H. H. Dai and Y. Z. Tang, Chin. J. Anal. Chem., 2014, 42, 1161–1170 CAS.
- N. L. Teradal, P. S. Narayan and S. Jaladappagari, Anal. Methods, 2013, 5, 7090–7095 RSC.
- Y. N. Ni, N. Deng and S. Kokot, Int. J. Environ. Anal. Chem., 2009, 89, 939–955 CrossRef CAS.
- M. R. Moghadam, S. Dadfarnia, A. M. H. Shabani and P. Shahbazikhah, Anal. Biochem., 2011, 410, 289–295 CrossRef CAS PubMed.
- Y. N. Ni, N. Deng and S. Kokot, Anal. Methods, 2010, 2, 1302–1309 RSC.
- G. Mansori and S. Asadpour, Asian J. Chem., 2011, 23, 268–272 CAS.
- A. Garcia-Reiriz, P. C. Damiani and A. C. Olivieri, Talanta, 2007, 71, 806–815 CrossRef CAS PubMed.
- Y. N. Ni, C. F. Huang and S. Kokot, Anal. Chim. Acta, 2007, 599, 209–218 CrossRef CAS PubMed.
- Q. L. Zhang, Y. N. Ni and S. Kokot, J. Agric. Food Chem., 2013, 61, 7730–7738 CrossRef CAS PubMed.
- F. Y. Tan, T. Chao, A. P. Zhao and M. L. Li, J. Agric. Food Chem., 2011, 59, 10839–10847 CrossRef CAS PubMed.
- D. Arroyo, M. C. Ortiz, L. A. Sarabia and F. Palacios, J. Chromatogr. A, 2008, 1187, 1–10 CrossRef CAS PubMed.
-
D. Harvey, Modern Analytical Chemistry, McGraw-Hill, New York, 2000 Search PubMed.
- R. Bro, Chemom. Intell. Lab. Syst., 1999, 46, 133–147 CrossRef CAS.
- A. C. Olivieri, Anal. Methods, 2012, 4, 1876–1886 RSC.
-
R. Bro, Multi-way analysis in the food industry: models, algorithms and applications, Doctoral thesis, University of Amsterdam, 1998.
- Y. N. Ni, W. Q. Xiao and S. Kokot, Food Chem., 2009, 113, 1339–1345 CrossRef CAS.
- R. Ergon, Chemom. Intell. Lab. Syst., 2003, 65, 293–303 CrossRef CAS.
- A. Pulido, I. Ruisanchez and F. X. Rius, Anal. Chim. Acta, 1999, 388, 273–281 CrossRef CAS.
- B. Karl, M. H. John, W. B. Lloyd and R. K. Bruce, J. Chemom., 1995, 9, 263–282 CrossRef.
- A. A. Y. EI-Sayed and N. A. EI-Salem, Anal. Sci., 2005, 21, 595–614 CrossRef.
-
N. R. Draper and H. Smith, Applied Regression Analysis, Wiley, New York, 3rd edn, 1998 Search PubMed.
- J. N. Miller, Analyst, 1991, 116, 3–14 RSC.
-
Y. N. Ni, Application of Chemometrics in Analytical Chemistry, Chinese Science Press, Beijing, 2004 Search PubMed.
- M. Otto and W. Wegscheider, Anal. Chem., 1985, 57, 63–69 CrossRef CAS.
|
This journal is © The Royal Society of Chemistry 2016 |