DOI:
10.1039/C5SC00794A
(Edge Article)
Chem. Sci., 2015,
6, 3886-3890
Unified total synthesis of the natural products endiandric acid A, kingianic acid E, and kingianins A, D, and F†
Received
5th March 2015
, Accepted 27th April 2015
First published on 7th May 2015
Abstract
A measure of the strength of a synthetic strategy is its versatility: specifically, whether it allows structurally distinct targets to be prepared. Herein we disclose a unified approach for the total synthesis of natural products of three distinct structural types, all of which occur naturally as racemic mixtures. The point of divergence involves the terminal alkylation of a conjugated tetrayne, and culminates in a significantly shortened synthesis of endiandric acid A (8 steps), the first total synthesis of kingianic acid E (8 steps), and a second-generation synthesis of kingianins A, D, and F (11 steps). Evidence for redox catalysis in the biosynthesis of kingianic acid E is presented.
Introduction
Isolated from the leaves of Endiandra intorsa, the endiandric acids1 (e.g. endiandric acid A, 1, Scheme 1) possess complex and synthetically challenging tetracyclic frameworks. Black, Banfield and co-workers brilliantly de-convoluted their structural complexity, and proposed a biosynthetic hypothesis involving a domino sequence of pericyclic reactions from linear conjugated (E,Z,Z,E)- or (Z,Z,Z,Z)-tetraene precursors.2 The endiandric acids were isolated as racemic mixtures, hence the proposed thermal 8π-conrotatory/6π-disrotatory electrocyclization-intramolecular Diels–Alder (IMDA) sequence was postulated to occur “readily… in non-enzymic reactions”.2 Nicolaou and co-workers' landmark biomimetic synthesis of endiandric acids A–G provided experimental support to the Black/Banfield hypothesis and identified the (E,Z,Z,E)-tetraene as a viable biosynthetic precursor.3 Our recent total synthesis of the kingianins4 (e.g. kingianin A, 2, Scheme 1) – structures that are biosynthetically formulated as products of a thermal 8π–6π electrocyclization then Diels–Alder dimerization – examined the (Z,Z,Z,Z)-tetraene as a possible biosynthetic precursor5 for the first time.6 Thus, a bold approach involving a four-fold cis-selective partial reduction of a conjugated tetrayne led to the electrocyclization precursor, albeit from a non-selective crossed Mori–Hiyama coupling. The high temperatures required to achieve (Z,Z,Z,Z)-tetraene 8π–6π electrocyclization led us to conclude that (E,Z,Z,E)-tetraenes are the more likely biosynthetic precursors to bicyclo[4.2.0]octadiene natural products.6 In spite of its unlikely intermediacy in biosynthetic pathways, the deployment of the (Z,Z,Z,Z)-tetraene enabled a step-economical7 synthesis of kingianins A (2), D and F (longest linear sequence 10 steps). Our synthetic studies also demonstrated that the kingianins were likely products of a SET-mediated stepwise intermolecular dimerization process.6 Important early studies by Moses described an inability to perform the concerted [4 + 2] cycloaddition.8a In studies conducted in parallel with our own, Parker performed an elegant intramolecular SET-mediated dimerization process.9a Parker subsequently disclosed a full account of a second-generation approach to kingianin natural products by way of intermolecular formal radical cation Diels–Alder reactions similar to our own.9b Most recently, Moses and co-workers successfully prepared kingianin A via an electrochemically-mediated formal radical cation Diels–Alder dimerization event.8b
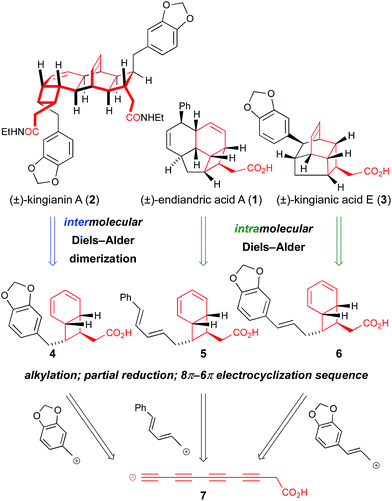 |
| Scheme 1 Retrosynthetic analysis of kingianin A, endiandric acid A, and kingianic acid E reveals the potential for a unified total synthesis. | |
Recently, Litaudon and co-workers reported the isolation of a second family of natural products from Endiandra kingiana, the kingianic acids10 (e.g. kingianic acid E, 3, Scheme 1). Biosynthetically, the tetracyclic framework of kingianic acid E (3) (Scheme 1) can be viewed as the result of a Black/Banfield-type2 domino 8π–6π electrocyclization–IMDA reaction sequence, albeit with diene and dienophile reversed, relative to endiandric acid A. Given the similar biosynthetic origin of kingianin,4 endiandric1 and kingianic10 natural products, we became intrigued by the opportunity for a unified synthetic approach to these polycyclic molecules.
Results and discussion
At the core of the strategy outlined in Scheme 1 is the identification of a common bicyclo[4.2.0]octadiene–CH2CO2H motif in precursors 4, 5, and 6 to kingianin A (2), endiandric acid A (1) and kingianic acid E (3), respectively. Each precursor would be required to undergo a different mode of (formal) [4 + 2] cycloaddition to furnish the requisite target. Bicyclo[4.2.0]octadienes 4, 5, and 6, differing only in the endo-substituent about the cyclobutane ring, could, in principle, be accessed through alkylation of a common tetrayne anion 7 with different electrophiles, followed by four-fold partial reduction and 8π–6π electrocyclization.
The feasibility of alkylating a synthetic equivalent of tetrayne anion 7 could not be objectively assessed at the outset of this work, since such transformations were without precedent.11,12 We viewed this lack of convincing precedent as an opportunity to explore new synthetic space. We elected to target tetrayne 8 (Scheme 2) in anticipation that the TMS- and –CH2CH2OTBS substituents would bestow a sufficient degree of stabilization to render the compound kinetically stable.13 The synthesis of tetrayne 8 was eventually optimized to a three-step sequence from commercially available alkyne 9 (Scheme 2). Thus, deprotonation of terminal alkyne 9 with n-butyllithium followed by trapping of the lithium acetylide with 4-formylmorpholine and a phosphate-buffered work-up furnished aldehyde 10 on decagram scale.14 Aldehyde 10 was then subjected to a Colvin alkyne homologation,15 using lithiated TMS diazomethane, and the resulting terminal diyne was deprotonated and brominated in situ to form bromodiyne 11. This modified homologation protocol, which provides direct access to 1-bromoalkynes from aldehydes, should enjoy wider application.16 Negishi cross-coupling17a of bromodiyne 11 with organozinc reagent 12
17b,c delivered tetrayne 8 and set the scene for the point of divergence in the synthesis. This short sequence has been reproduced several times to prepare multi-gram quantities of tetrayne 8.18 All attempts to directly generate an anionic-tetrayne species from TMS tetrayne 8 using TBAF19 or MeLi17b led to decomposition. This problem was solved by firstly generating the terminal tetrayne 13
20 by selective desilylation of 8 with potassium carbonate in methanol, and subsequent metalation with tert-butylmagnesium bromide. The resulting tetrayne Grignard reagent was then alkylated with allylic bromides 14
21 and 15 under Cu(I) catalysis.22 Tetraynes 16 and 17 were isolated in 16% and 29% yield, respectively, over two steps from TMS tetrayne 8. In the case of tetrayne 18, a Negishi cross coupling protocol, with benzyl bromide 19, proved superior.17a Thus, all of the carbon atoms required for endiandric acid A (1), kingianic acid E (3), and kingianins A (2), D (20), and F (21) were installed in a longest linear sequence of five steps from commercially available precursors. The somewhat modest yields obtained for the metallotetrayne alkylation betray a seminal transformation that requires further optimization. Nonetheless, the generality of this transformation, and the step-economy7 that it conveys upon this synthetic approach, are undeniable. This second-generation synthesis of unsymmetrical tetrayne 18 constitutes a formal synthesis of kingianins A, D, and F, and avoids the use of a non-selective Mori–Hiyama C(sp)–C(sp) cross-coupling, which was a weakness in our previous approach.6
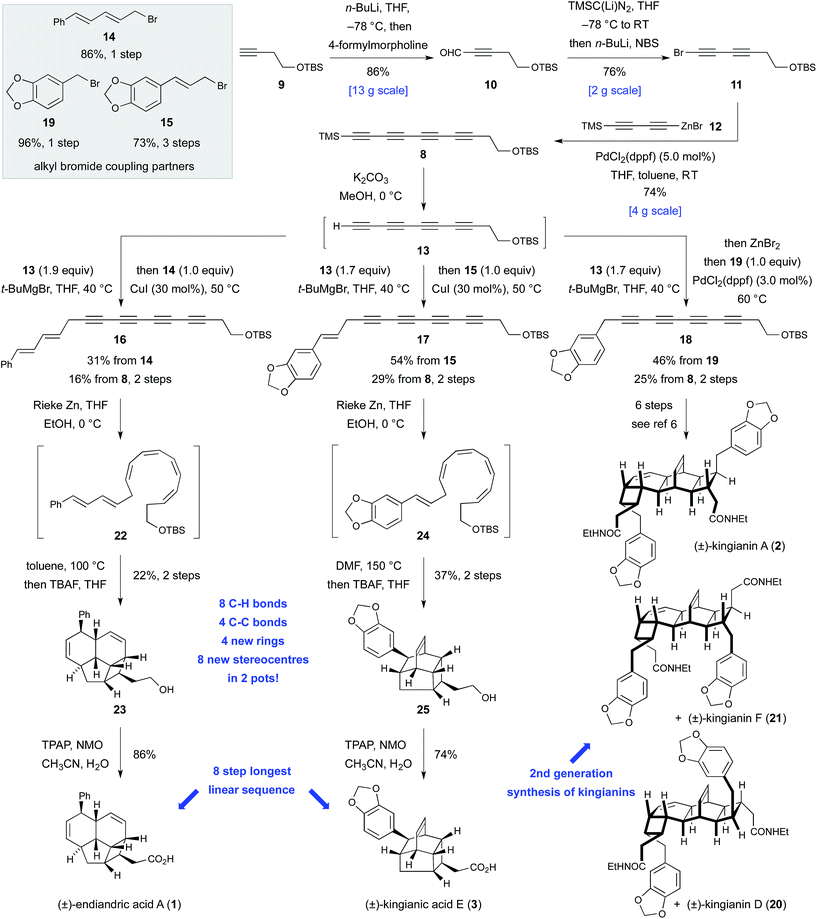 |
| Scheme 2 Total synthesis of the racemic natural products endiandric acid A (1), kingianic acid E (3), and kingianins A (2), D (20) and F (21). | |
Conversion of tetraynes 16 and 17 into endiandric acid A (1) and kingianic acid E (3) first required transformation of the conjugated tetrayne units into the corresponding (Z,Z,Z,Z)-tetraenes. Subjection of endiandric tetrayne 16 to our previously optimized cis-selective partial reduction conditions6 successfully generated (Z,Z,Z,Z)-tetraene 22. This material was then immediately heated to 100 °C in toluene to bring about the requisite domino 8π–6π electrocyclization–IMDA sequence. Subsequent TBS deprotection, conducted in the same flask, led to isolation of alcohol 23 in 22% yield from tetrayne 16. Finally, oxidation with TPAP/NMO23 furnished a synthetic sample of endiandric acid A (1).24 Thus, some 30 years after Black and Banfield's biosynthetic hypothesis and Nicolaou's ground-breaking biomimetic synthesis via the (E,Z,Z,E)-tetraene, the (Z,Z,Z,Z)-tetraene route to endiandric acid A has finally been realized in the laboratory.
The first total synthesis of kingianic acid E (3) was achieved in a similar fashion. Partial reduction of kingianic tetrayne 17, followed by heating of (Z,Z,Z,Z)-tetraene 24 to 150 °C in DMF and in situ TBAF deprotection, led to the isolation of tetracyclic alcohol 25 in 37% yield. Oxidation gave kingianic acid E (3), the analytical data of which matched that reported by Litaudon and co-workers in all respects.10
The thermal, concerted IMDA reaction en route to kingianic acid E (3) was sufficiently sluggish at 88 °C to allow isolation of bicyclo[4.2.0]octadiene 26 (Scheme 3). The lethargic behavior of this concerted cycloaddition is hardly surprising given the electron rich nature of the dienophile of 26. In light of this observation, we ventured that the biosynthesis of kingianic acid E (3) might involve a redox-catalyzed process.
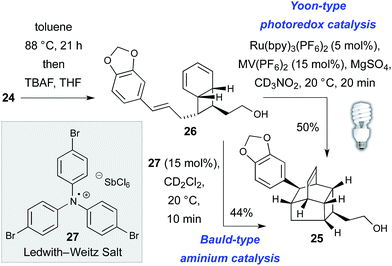 |
| Scheme 3 Radical cation formal IMDA reaction of bicyclo[4.2.0]octadiene 26. | |
Support for this proposal was obtained in the form of a rapid formal Diels–Alder reaction of bicyclo[4.2.0]octadiene 26 to alcohol 25 at ambient temperature using either visible light photoredox catalysis25 or via treatment with the Ledwith–Weitz radical cation salt 27.26 Given that, under redox catalysis, the formal IMDA reaction of bicyclo[4.2.0]octadiene 26 is significantly faster than the concerted, thermal cycloaddition, we became curious as to whether the entire 8π–6π double electrocyclization–IMDA sequence might be a redox-catalyzed process in Nature. While there is no compelling mechanistic case for redox catalysis of the electrocyclization sequence, in principle the conversion of tetraene 24 to bicycle 26 could also occur by way of a redox-catalyzed [2 + 2]-cycloaddition mechanism, for which there is precedent.27 Disappointingly, when (Z,Z,Z,Z)-tetraene 24 was subjected to both Bauld-type aminium catalysis and Yoon-type photoredox catalysis, only complex mixtures resulted, with no sign of the target products. These results do not disprove redox catalysis of the complete process (i.e. from tetraene 24 to caged product 25) in nature but they do appear to indicate that the formal Diels–Alder process is especially predisposed to it.
Conclusions
In summary, a unified synthesis of three major sub-families of polycyclic natural products from the Endiandra genus of plants has been accomplished. Our recently-developed four-fold cis-selective partial reduction of conjugated tetraynes to (Z,Z,Z,Z)-tetraenes has inspired a significantly shortened synthesis of endiandric acid A (longest linear sequences: this work, 8 steps; previous syntheses 14 steps [methyl ester]3 and 23 steps28), the first total synthesis of kingianic acid E and an improved synthesis of three kingianin natural products. We anticipate that this approach will prove applicable to step-economical syntheses of related natural products.
Acknowledgements
This work was supported by the Australian Research Council. S.L.D. gratefully acknowledges financial support from the Rickards family through the Rodney Rickards Scholarship. We thank Prof. David Black (UNSW) for providing authentic samples of the endiandric acids.
Notes and references
- The endiandric acids:
(a) W. M. Bandaranayake, J. E. Banfield, D. St. C. Black, G. D. Fallon and B. M. Gatehouse, J. Chem. Soc., Chem. Commun., 1980, 162 RSC;
(b) W. M. Bandaranayake, J. E. Banfield, D. St. C. Black, G. D. Fallon and B. M. Gatehouse, Aust. J. Chem., 1981, 34, 1655 CrossRef CAS;
(c) W. M. Bandaranayake, J. E. Banfield and D. St. C. Black, Aust. J. Chem., 1982, 35, 557 CrossRef CAS;
(d) W. M. Bandaranayake, J. E. Banfield, D. St. C. Black, G. D. Fallon and B. M. Gatehouse, Aust. J. Chem., 1982, 35, 567 CrossRef CAS;
(e) J. E. Banfield, D. St. C. Black, S. R. Johns and R. I. Willing, Aust. J. Chem., 1982, 35, 2247 CrossRef CAS.
- W. M. Bandaranayake, J. E. Banfield and D. St. C. Black, J. Chem. Soc., Chem. Commun., 1980, 902 RSC.
-
(a) K. C. Nicolaou, N. A. Petasis, R. E. Zipkin and J. Uenishi, J. Am. Chem. Soc., 1982, 104, 5555 CrossRef CAS;
(b) K. C. Nicolaou, N. A. Petasis, J. Uenishi and R. E. Zipkin, J. Am. Chem. Soc., 1982, 104, 5557 CrossRef CAS;
(c) K. C. Nicolaou, R. E. Zipkin and N. A. Petasis, J. Am. Chem. Soc., 1982, 104, 5558 CrossRef CAS;
(d) K. C. Nicolaou, N. A. Petasis and R. E. Zipkin, J. Am. Chem. Soc., 1982, 104, 5560 CrossRef CAS.
- The kingianins:
(a) A. Leverrier, M. E. Tran Huu Dau, P. Retailleau, K. Awang, F. Guéritte and M. Litaudon, Org. Lett., 2010, 12, 3638 CrossRef CAS PubMed;
(b) A. Leverrier, K. Awang, F. Guéritte and M. Litaudon, Phytochemistry, 2011, 72, 1443 CrossRef CAS PubMed.
- Huisgen and co-workers have shown that 8π–6π electrocyclization of the 1,8-dimethyl-substituted (Z,Z,Z,Z)-octatetraene required a higher temperature than the (E,Z,Z,E)-congener: R. Huisgen, A. Dahmen and H. Huber, J. Am. Chem. Soc., 1967, 89, 7130 CrossRef CAS.
- S. L. Drew, A. L. Lawrence and M. S. Sherburn, Angew. Chem., Int. Ed., 2013, 52, 4221 CrossRef CAS PubMed.
-
(a)
P. A. Wender and B. L. Miller, Organic Synthesis: Theory and Applications, ed. T. Hudlicky, JAI, Greenwich, 1993, vol. 2, pp. 27–66 Search PubMed;
(b) P. A. Wender, V. A. Verma, T. J. Paxton and T. H. Pillow, Acc. Chem. Res., 2008, 41, 40 CrossRef CAS PubMed;
(c) P. A. Wender and B. L. Miller, Nature, 2009, 460, 197 CrossRef CAS PubMed.
-
(a) P. Sharma, D. J. Ritson, J. Burnley and J. E. Moses, Chem. Commun., 2011, 47, 10605 RSC;
(b) For a recent formal synthesis of kingianin A, see: J. C. Moore, E. S. Davies, D. A. Walsh, P. Sharma and J. E. Moses, Chem. Commun., 2014, 50, 12523 RSC.
-
(a) H. N. Lim and K. A. Parker, Org. Lett., 2013, 15, 398 CrossRef CAS PubMed;
(b) H. N. Lim and K. A. Parker, J. Org. Chem., 2014, 79, 919 CrossRef CAS PubMed.
- The kingianic acids: M. N. Azmi, C. Gény, A. Leverrier, M. Litaudon, V. Dumontet, N. Birlirakis, F. Guéritte, K. H. Leong, S. N. A. Halim, K. Mohamad and K. Awang, Molecules, 2014, 19, 1732 CrossRef PubMed.
- The highest
reported example of terminal alkyne alkylation is that of a conjugated 1,3-diyne; for selected examples, see:
(a) N. J. Matovic, P. Y. Hayes, K. Penman, R. P. Lehmann and J. J. De Voss, J. Org. Chem., 2011, 76, 4467 CrossRef CAS PubMed;
(b) V. Fiandanese, D. Bottalico, G. Marchese and A. Punzi, J. Organomet. Chem., 2005, 690, 3004 CrossRef CAS PubMed;
(c) V. Fiandanese, D. Bottalico, G. Marchese and A. Punzi, Tetrahedron Lett., 2003, 44, 9087 CrossRef CAS PubMed.
- For a single example of terminal tetrayne lithiation and subsequent nucleophilic addition to aldehydes and ketones, see:
(a) M. Yamaguchi, H.-J. Park, M. Hirama, K. Torisu, S. Nakamura, T. Minami, H. Nishihara and T. Hiraoka, Bull. Chem. Soc. Jpn., 1994, 67, 1717 CrossRef CAS;
(b) M. Yamaguchi, K. Torisu, S. Nakamura and T. Minami, Chem. Lett., 1990, 19, 2267 CrossRef.
-
(a) W. A. Chalifoux and R. R. Tykwinski, C. R. Chim., 2009, 12, 341 CrossRef CAS PubMed;
(b) W. A. Chalifoux and R. R. Tykwinski, Nat. Chem., 2010, 2, 967 CrossRef CAS PubMed.
-
(a) M. Journet, D. Cai, L. M. DiMichele and R. D. Larsen, Tetrahedron Lett., 1998, 39, 6427 CrossRef CAS;
(b) H. F. Sneddon, M. J. Gaunt and S. V. Ley, Org. Lett., 2003, 5, 1147 CrossRef CAS PubMed.
- E. W. Colvin and B. J. Hamill, J. Chem. Soc., Perkin Trans. 1, 1977, 869 RSC.
- For an alternative one-pot alkyne homolgation-bromination protocol, see: X. Y. Mak, A. L. Crombie and R. L. Danheiser, J. Org. Chem., 2011, 76, 1852 CrossRef CAS PubMed.
-
(a) M. Qian and E.-I. Negishi, Tetrahedron Lett., 2005, 46, 2927 CrossRef CAS PubMed;
(b) A. B. Holmes and G. E. Jones, Tetrahedron Lett., 1980, 21, 3111 CrossRef CAS;
(c) T. N. Hoheisel and H. Frauenrath, Org. Lett., 2008, 10, 4525 CrossRef CAS PubMed.
- Importantly, TMS tetrayne 8 proved to be a stable oil that could be stored neat in the freezer for several months without any appreciable decomposition.
- F. Montel, R. Beaudegnies, J. Kessabi, B. Martin, E. Muller, S. Wendeborn and P. M. J. Jung, Org. Lett., 2006, 8, 1905 CrossRef CAS PubMed.
-
Caution: terminal tetrayne 13 is unstable when concentrated neat and must be handled as a solution; see the ESI† for details.
- D. D. Kim, S. J. Lee and P. Beak, J. Org. Chem., 2005, 70, 5376 CrossRef CAS PubMed.
-
L. Brandsma, Reactions of Metallated Acetylenes and Allenes with Alkylating Agents. Synthesis of Acetylenes, Allenes and Cumulenes: Methods and Techniques, Elsevier, Oxford, 2004, ch. 4, p. 87 Search PubMed.
- A.-K. C. Schmidt and C. B. W. Stark, Org. Lett., 2011, 13, 4164 CrossRef CAS PubMed.
- All spectroscopic data of our synthetic sample matched that of an authentic natural endiandric acid A sample kindly provided by Prof. David Black.
-
(a) S. Lin, M. A. Ischay, C. G. Fry and T. P. Yoon, J. Am. Chem. Soc., 2011, 133, 19350 CrossRef CAS PubMed;
(b) S. Lin, C. E. Padilla, M. A. Ischay and T. P. Yoon, Tetrahedron Lett., 2012, 53, 3073 CrossRef CAS PubMed.
- D. J. Bellville, D. W. Wirth and N. L. Bauld, J. Am. Chem. Soc., 1981, 103, 718 CrossRef CAS.
-
(a) Z. Lu and T. P. Yoon, Angew. Chem., Int. Ed., 2012, 51, 10329 CrossRef CAS PubMed;
(b) J. Du, K. L. Skubi, D. M. Schultz and T. P. Yoon, Science, 2014, 344, 392 CrossRef CAS PubMed;
(c) A. E. Hurtley, Z. Lu and T. P. Yoon, Angew. Chem., Int. Ed., 2014, 53, 8991 CrossRef CAS PubMed.
- S. A. May, P. A. Grieco and H.-H. Lee, Synlett, 1997, 493 CrossRef CAS PubMed.
Footnotes |
† Electronic supplementary information (ESI) available: Details of experimental procedures, spectroscopic data, and copies of 1H and 13C NMR spectra. See DOI: 10.1039/c5sc00794a |
‡ Present address: School of Chemistry, University of Edinburgh, Joseph Black Building, David Brewster Road, Edinburgh, EH9 3FJ, UK, E-mail: E-mail: a.lawrence@ed.ac.uk. |
|
This journal is © The Royal Society of Chemistry 2015 |