DOI:
10.1039/C5RA04438C
(Paper)
RSC Adv., 2015,
5, 52420-52426
Design and development of a topical dosage form for the convenient delivery of electrospun drug loaded nanofibers
Received
13th March 2015
, Accepted 8th June 2015
First published on 8th June 2015
Abstract
In the recent years, nanofiber based delivery systems have gained a lot of importance in the biomedical field. However, convenient administration of nanofibers and development of a dosage form for nanofibers is a great challenge to the formulation scientist. Topical application of nanofibers has its own disadvantages such as vehicle deployment, spreading and retention in the specific site of action. Thus, in this study, we have attempted to develop a topical gel for the effective application and administration of fluconazole loaded eudragit nanofibers. The electrospun nanofiber loaded topical gel was evaluated for the drug content, rheological behavior, permeation efficiency and antifungal activity. Controlled release of the drug up to 24 h was observed in the in vitro diffusion studies and a better drug release was obtained in the nanofiber loaded gel based delivery system when compared to the release profile of nanofibers alone. Antifungal study confirmed the efficiency of the developed system in controlling fungal growth. Thus, an efficient dosage form for the delivery of drug loaded nanofibers has been developed and it opens up new avenues for the treatment of topical infections using nanofiber based therapeutics.
1. Introduction
Nanofibers have gained a lot of pharmaceutical interest in drug delivery due to their advantages like larger surface area, greater drug entrapment, minimal manufacturing wastage of formulation ingredients, better drug stability and ease of manufacturing.1–3 Electrospun nanofibers have helped in obtaining a controlled release of the drug systemically thereby achieving maximum bioavailability of the drug at the target site in any of the drug delivery routes.4 Another important factor due to which nanofibers has drawn the interest of the researchers was its core sheath structure which could be useful in encapsulating multiple drugs and for pulsatile drug delivery.5–7 Nanofibers can be readily applied as a dressing material for topical wounds and to treat other dermatological conditions. The application of nanofibers for the treatment of skin infections and regeneration has been discussed in various reviews.8–10 Azarbayjani et al., has developed a smart polymeric nanofibers for the topical delivery of levothyroxine which helps to reduce the deposition of adipose tissue on skin.11 Papain loaded poly vinyl alcohol nanofibers have been developed with an aim to enhance the wound debridement to hasten the process of wound healing.12 However, adherence and application of nanofibers topically to skin has certain challenges such as vehicle deployment, spreading, retention and hence may have poor patient compliance.13
Topical delivery systems have gained importance in the current scenario of drug delivery due to its high patient compliance, localized activity of the drug directly at the target site and thus reduced the risk of systemic side effects.14 Topical gels were selected in the present study due to their ease of application, non-greasiness and enhanced stability when compared to other semisolid dosage forms.15 Anionic acrylic acid derived polymer like carbopol 940 was preferred due to its excellent gelling properties and rheological behavior. Being an anionic polymer, it can be jellified by the usage of neutralizing agents like tri-ethanol amine. Carbopol has the advantage of greater viscosity at a lower concentration, good flow property, better thermal stability, and good adhering property.16
Wound infections are of major health concern and the most devastating form of trauma worldwide.17 These infections are caused not only by bacteria but also due to variety of fungal species. Most of the fungal infections are overlooked because of lack of clinical awareness, longer growth period and lack of good mycology laboratories.18 Major risk factors for acquiring fungal infection in wounds depends on body surface area, full thickness burns, prolonged hospital stay, open dressing, central venous catheters, antibiotics, steroid treatment, long-term artificial ventilation, fungal wound colonization (FWC), hyperglycemic episodes and other immunosuppressive disorders.19–21 Apart from this, chronic wound has the greater propensity for fungal infection. Therefore, development of materials that enhance the wound healing process along with the antifungal therapeutics would reduce the complication of wounds as well as trauma.
In humans, most of the topical fungal diseases were caused by Candida species.22 Fungal infection arises due to the fungal infestation through the topical layers of the skin and damage to the superficial stratum corneum layer.23 In this study, effective fungi static drug fluconazole was selected as a model drug to evaluate the efficiency of the delivery system. Fluconazole has gained interest in the treatment of topical fungal diseases due to its advantages of longer half life, lower molecular weight, poor protein binding and high level of penetration ability.24
Fluconazole loaded eudragit nanofiber dispersed topical gel based delivery system has been developed to facilitate the adherence and better intactness of the nanofibers on the surface of the skin. For the first time, a topical dosage form for the delivery of nanofiber has been attempted to enhance its applicability. This delivery system is expected to have better patient compliance due to its ease of application and handling.
2. Experimental section
Eudragit L 100 was gifted by Evonik Rohm Pharma polymers (Degussa India pvt. Ltd, India). Fluconazole was purchased from sigma life sciences (Sigma-Aldrich, U.S.A). Carbopol 940 was purchased from Loba chemie pvt. Ltd. All the other reagents used were of analytical grade.
2.1. Preparation of electrospinning solutions
Eudragit solution (10% w/v) was prepared using methanol as the solvent and after complete dissolution of the polymer, fluconazole was added to it. Drug and polymer were taken in a ratio of 1
:
5 and the solution was kept under continuous stirring to obtain a homogenous spinning solution.
2.2. Electrospinning process
The mixture of drug polymer solution was loaded in a 5 ml syringe. The flow rate was maintained at a constant feeding rate of 0.40 ml h−1 controlled by a syringe pump (Physics equipment & Co. India). A high voltage of 15–16 kV was applied to the metallic needle having an inner hole diameter of 0.5 mm. A horizontal collector containing an aluminum foil has been fixed at a distance of 18 cm away from the needle tip to collect the nanofibers. All the electrospinning procedures were conducted at the optimum conditions (temperature 24 ± 1 °C, relative humidity 68 ± 3%). The prepared nanofibers were dried at 40 °C under vacuum to ensure complete removal of organic residual solvents and moisture.
2.3. Preparation of electrospun nanofiber gel
Antifungal gel was prepared using dispersion method. Carbopol was dispersed in water and was left for an hour to ensure complete hydration and swelling of polymer. Weighed quantity of electrospun nanofibers equivalent to 30 mg of fluconazole were added to the polymer solution with continuous stirring to ensure the homogenous dispersion of electrospun fibers throughout the gel. Methyl and propyl paraben were added to prevent the microbial growth in the gel. Tri-ethanolamine was added in a sufficient quantity to the polymer–fiber mixture for neutralization and formation of clear homogenous gel. The gel was stored in the refrigerator until further evaluation.
2.4. Characterization
2.4.1. Morphological characterization. Surface morphology of the drug loaded electrospun nanofibers was studied using scanning electron microscope (Hitachi, Japan). A small piece of nanofibrous mat was affixed on the stud and the sample was subjected to gold coating in an inert atmosphere to render it electrically conductive. Images were obtained at an excitation voltage of 5–15 kV.The distribution of fibers in the carbomer gel was observed using scanning electron microscopy. The electrospun nanofibers loaded gel was subjected to imaging in the same pattern as that of nanofibers.
2.4.2. Compatibility studies. Fourier transform infrared spectrum was acquired using Perkin-Elmer FTIR spectrophotometer (USA) by using KBr disk method. The spectrum of drug, polymer, physical mixture of polymer & drug and drug loaded electrospun nanofibers were determined in a spectral range of 4000–400 cm−1.
2.4.3. Physical state of drug. Differential scanning calorimetric analysis was done using Q-200 differential scanning calorimeter (TA instruments co., U.S.A). The samples were sealed in the aluminum pans and were heated at a constant rate of 10 °C min−1 between the temperature ranges of 20–300 °C using nitrogen gas to create an inert atmosphere which was purged at a rate of 20 ml min−1.
2.4.4. Physical appearance and rheological behavior of gel. The prepared gel was visually examined for homogeneity. The pH of the gel was determined. Rheological behavior of the gel was estimated at ambient temperature condition using Brookfield rheometer with coaxial measuring system. 20 g of blank gel and nanofiber containing carbopol gel was placed in measuring gap between the stationary measuring cup and the rotating measuring bob. The rheogram was obtained by applying the shear rates in the range of 0 to 600 per second.
2.4.5. Loading efficiency of nanofibers. The drug content in the nanofibers was estimated by dissolving fluconazole loaded nanofibers (2 mg) in a suitable solvent (1 ml methanol) and was analyzed spectrophotometrically at 262 nm. Drug loading was estimated using the following equation,
2.4.6. Content uniformity of gel. The drug content of gel is checked by dissolving 100 mg of gel in 1 ml methanol and was analyzed spectrophotometrically at a wavelength of 262 nm after suitable dilution. The analysis was performed in triplicates and the average was reported.
2.4.7. In vitro drug release and permeation studies. 100 mg of the electrospun fluconazole loaded nanofibers was weighed and suspended in the dissolution medium (PBS pH-7.4), to study the release pattern of the drug from the fibers. The release study was conducted at 37 ± 0.5 °C and 100 rpm. Aliquots of 1 ml each were withdrawn and replaced by an equal amount of fresh dissolution medium at regular intervals of time (60 min) to maintain a constant volume. The collected samples were analyzed using UV spectrophotometer for drug release using pH 7.4 phosphate buffer as blank at a wavelength of 262 nm.In vitro permeation study of electrospun nanofiber loaded gel was carried out using dialysis method. A semipermeable egg membrane was isolated from egg using 20% HCl. The isolated membrane was repeatedly washed to remove the debris of the egg shell using distilled water and immersed in phosphate buffer (pH 7.4) for 24 hours. 1 g of the gel was accurately weighed and placed on the egg membrane and tied to one end of the open ended cylinder (donor compartment) and was immersed in a beaker containing100 ml of buffer (receptor compartment). This diffusion system was maintained at 37 ± 0.5 °C and 100 rpm. Aliquots were withdrawn at regular intervals of time and were analyzed spectrophotometrically at 262 nm.
2.4.8. Antifungal assay. The antifungal assay for fluconazole was performed using yeast extract medium by micro broth assay. Yeast extract medium (broth and agar) was prepared and sterilized in an autoclave at 15 Lbs pressure for 15 min at a temperature of 121 °C.Yeast extract agar medium was poured in sterile Petri plates (control and test) and allowed to solidify in laminar air flow system. A single strain of Candida albicans was taken from the glycerol stock, streaked on the agar plate and kept in the incubator at 25 ± 0.5 °C for 48 h. From this a single inoculum of fungal strain was taken and inoculated in broth. Broth (20 ml) was taken in clean sterilized conical flasks (control and blank). The fungal strain was inoculated in one flask and was set as control while the other was kept free from organism (blank). This was kept for incubation for 24 h and observed for growth of fungal organisms in control. This was termed as the mother culture.
Serial dilution of the broth (5 ml) were prepared containing 10 μl of the mother culture along with different concentrations of the gel (10 mg, 25 mg, 50 mg and 100 mg) along with control (without formulation) and blank. These were incubated at 25 °C to ensure the growth of the fungal strains. After 24 h of incubation in broth, optical density (OD) values for the growth of organism were obtained in a microplate reader. 200 μl of fungal broth of each concentration along with control was taken in a sterile well micro plate and kept in microplate reader and OD values were obtained at 405 nm. A turbidimetric growth curve was plotted based on the OD values of the fungal growth for each drug concentration and control. At the same time 10 μl of the formulated broth is taken from each concentration and inoculated on the agar plate by spread plate technique using an L-rod. The plates were incubated at 25 °C and the growth of colonies was observed after 48 h.
2.4.9. Live/dead assay. The live/dead assay was performed to understand the cytocompatibility and fungicidal activity of the drug loaded nanofibers. Cytocompatibility of the nanofibers was evaluated by performing MTT (3-(4,5-dimethylthiazol-2-yl)-2,5-diphenyl tetrazolium bromide) assay25 using immortalized stromal epidermal keratinocyte (HaCaT) cells. Cells were seeded on the coverslips coated with drug loaded nanofibers in the 12 well plates (7000 cells per well) containing Dulbecco's modified eagle medium (DMEM) with 10% FBS and allowed to adhere overnight in a CO2 incubator. Controls were maintained on empty coverslips without the influence of any treatment. After 24 h, 1 ml of MTT solution (0.5 mg ml−1) was added to each culture well and incubated at 37 °C for 3–4 h. At the end of the incubation period, the medium was removed and the purple formazan crystals formed were solubilised with dimethyl sulphoxide. The absorbance of this solution was measured at a wavelength of 570 nm using a micro plate reader (Bio-Rad). Cell viability was also measured by acridine orange/propidium iodide (AO/PI) staining. The cells were stained with 1 μl of 10 μg each of AO and PI in PBS. The cells were incubated for 20 minutes at 37 °C and viewed using a fluorescent microscope (450–490 nm) (Leica Microscope, Germany).The fungicidal activity of fluconazole loaded nanofibers was tested by adapting the method followed by Lee et al.26 For this, tubes containing 2 ml of broth was inoculated with 10 μl of the mother culture and nanofibers equivalent to 10 mg of fluconazole was added to one of the tube. Control was maintained in the same condition without the influence of drug loaded nanofibers. These were incubated at 25 °C to ensure the growth of the fungal strains. After 24 h of incubation in broth, the yeast cells were stained with molecular probes such as acridine orange and propidium iodide and then examined under the fluorescent microscope (450–490 nm) (Leica Microscope, Germany).
3. Results and discussion
The current treatment methods for topical infections comprises from the delivery of silver sulfadiazine to newer antibiotics like mupirocin.27,28 However, treating with these antibiotics leads to the development of drug resistance and hence necessitates the development of sustained release system for these drugs. Nanotechnology based techniques offers solutions to this problem and formulations such as Acticoat A.B, Silverlon and Silvasorb are few examples of such products.8 Anti-fungal drug loaded eudragit nanofibers has been developed using electrospinning technique. Further, to facilitate the easy administration of the nanofibers a topical gel based dosage form was developed. Administration of the nanofibers using topical gel system allows for prioritization of physical attributes which effect patient perceptions without disturbing the biological activity of the drug.
3.1. Morphological characterization
The morphology of the drug loaded nanofiber was determined using SEM as shown in Fig. 1a. The nanofibers possessed a semitransparent appearance with an ultrafine structure and smooth surface. The diameter of nanofibers was estimated to be in the range of 50–200 nm and ensuring that the drug encapsulated in the fibers was in a nano range.
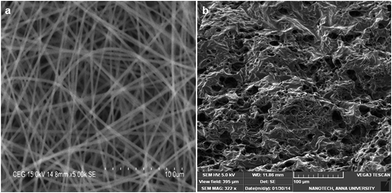 |
| Fig. 1 Scanning electron micrographic image of (a) fluconazole loaded eudragit nanofibers and (b) drug loaded nanofibers dispersed carbomer gel. | |
Gel containing nanofibers was assessed using SEM and the result was given in Fig. 1b. The image depicted the uniform dispersion of the fibers throughout the gel matrix. During the gold sputter coating of the antifungal gel, the moisture content was lost due to the application of vacuum and we have observed a spongy mass with vacuoles or air spaces before imaging. The same may be confirmed from the images by the presence of pore like structures.
3.2. Compatibility studies
Prominent secondary interactions such as hydrogen bonds, electrostatic interactions, and hydrophobic interactions between the components of the formulation tend to increase the compatibility between the components of the formulation which in turn results in the yielding of a better delivery system.29
The secondary interactions between the individual components of nanofibers were studied by FTIR spectroscopy and the results were given in Fig. 2a–d. The resulted spectrum of the individual components, physical mixture of polymer and drug, and the drug loaded nanofibers showed distinct peaks. The spectrum of fluconazole showed C–F stretching at 1137 cm−1, C–H stretching at 2996 cm−1, C–C stretching at 1560 cm−1 & C
C stretching at 1622 cm−1. Polymer spectrum showed prominent broad band of hydroxyl group stretching at 2950 cm−1, C–O stretching vibration at 1720 cm−1, characteristic band of ester vibrations was obtained in the range of 1268–1161 cm−1.
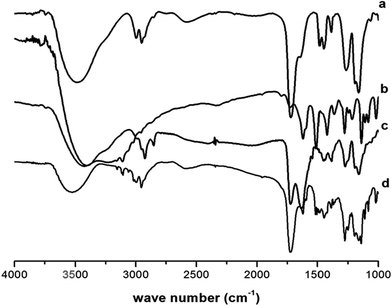 |
| Fig. 2 FTIR spectrum of (a) Eudragit L 100 (b) fluconazole (c) physical mixture of drug and polymer and (d) drug loaded composite electrospun nanofibers. | |
Spectrum of physical mixture was also probed and it displayed the distinct peaks of both the drug and polymer with slight variations. A characteristic C–C stretching band of Eudragit L-100 was seen at 1560 cm−1, C–H stretching at 2968 cm−1 and prominent peak corresponding to C
C stretching was observed at 1622 cm−1. The peaks corresponding to fluconazole were also observed at 1139 cm−1 corresponding to C–F stretching and C
C stretching at 1622 cm−1. The spectrum of the drug loaded nanofibers showed characteristic peaks corresponding to the polymer alone at 2950 cm−1, 1720 cm−1 and 1268 cm−1. Thus, the FTIR spectrum of composite nanofibers showed prominent peaks of eudragit and the characteristic peaks of drug were scarcely present. This indicated that the drug has sufficient secondary interactions with the polymer and hence the eudragit nanofiber offers a stable and more compatible delivery system for fluconazole.
3.3. Physical state of drug in electrospun nanofibers
Physical state of the drug in the electrospun nanofibers is an important factor in achieving the expected in vitro drug release profile.30 DSC analysis data was used to evaluate the physical state of the components of electrospun nanofibers and the thermograms were given in Fig. 3a–d.
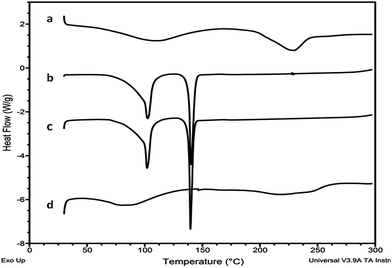 |
| Fig. 3 DSC thermograms of (a) Eudragit L 100 (b) fluconazole (c) physical mixture of drug and polymer and (d) drug loaded composite electrospun nanofibers. | |
Thermogram of the drug has shown a sharp endothermic peak at 140 °C corresponding to the melting point of the drug. The polymer thermogram has shown two distinct endothermic peaks at 109 °C and 230 °C corresponding to the glass transition phenomenon of Eudragit L 100. Thermogram of physical mixture of polymer and drug showed prominent transitions at 102 °C and 139 °C corresponding to the glass transition of Eudragit L 100 and melting of fluconazole respectively. DSC thermogram of the drug loaded nanofibers showed distinct peaks at 83 °C and 217 °C. The absence of fluconazole peak in the thermogram indicated that the drug has lost its crystalline structure and molecularly dispersed throughout the polymer matrix. The presence of drug in its amorphous form is a prerequisite to achieve sustained release from the respective formulation.31
3.4. Physical appearance and rheological behavior of gel
The physical evaluation of the nanofibers loaded carbomer gel revealed a semi-transparent and homogeneous outlook. pH of the gel was found to be in the range of 6.6–7.2. Hence, the pH of the nanofibers loaded gel was well within the limits of acceptable physiological pH of skin. The rheological studies indicated that, when a higher shear rate is applied, viscosity of the formulation decreases indicating the pseudo plastic behavior of the formulation.11 The rheogram (Fig. 4) of the electrospun nanofiber gel showed the shape of pseudo plastic (shear thinning) behavior of the formulation. Thus the desired characteristic feature for the pharmaceutical formulation for topical use was achieved. The rheogram revealed that the addition of nanofiber to the carbopol did not show much variation in the viscosity of the gel. The physical evaluation of the gel after the analysis indicated that the application of higher shear rate (>500 per second) did not break or deformed the gel. This suggests that the formulation had good gel strength.
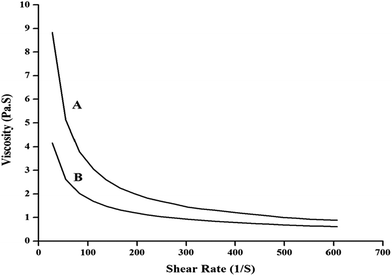 |
| Fig. 4 Rheological behavior of carbopol gel (a) without nanofibers and (b) with nanofibers. | |
3.5. Drug loading and content uniformity in gel
The loading of fluconazole in eudragit nanofibers was found to be 14 ± 1.38% w/w and the drug content in the gel formulation was found to be 3.5 ± 0.63% w/w. The content uniformity was verified by estimating the concentration of drug in three different portions of the gel and the average was reported.
3.6. In vitro drug release and permeation studies
In vitro release studies of the drug from the electrospun nanofibers and the topical gel were carried out using pH 7.4 PBS at a temperature of 37 ± 0.5 °C and 100 rpm. The release profile of nanofibers (Fig. 5a) at the end of 2 h showed 34.5% of drug release and then a gradual release were obtained till 8 h. Finally, at the end of 24 h 54.2% drug release was observed. This has clearly evidenced that the polymer has showed a very good sustainability in the release pattern of the drug from the fibers.
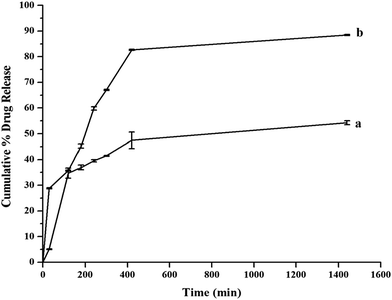 |
| Fig. 5 In vitro release profiles from (a) eudragit nanofibers and (b) carbopol gel dispersed with drug loaded nanofibers (data were the mean ± SD of three measurements). | |
In case of release studies from the topical antifungal gel dispersed with drug encapsulated electrospun fibers has showed a better release profile. The release profile was shown in Fig. 5b. At the end of initial 2 h, 35% of the drug was released. Around 88% drug release was observed after a total period of 24 h. The increase in the percentage of drug release from the gel may be due to the swelling of the polymer in the gel owing to the presence of alkaline atmosphere in the gel matrix. The swelling of the polymer facilitated the increased drug release from the gel formulation. Further, the disruption in the fiber network due to the mechanical force applied during the formulation of gel could also contribute to the enhanced drug release. The better drug release profile of the gel suggests that the attempt of developing a gel based nanofiber drug delivery system was ideal for the treatment of topical infections.
3.7. Antifungal assay
Fungal inhibition assay was carried out in both kinetics and agar plating method. Turbidimetric growth curves of Candida albicans during the period of incubation were plotted in the increasing order of concentration of formulation. After 24 h of incubation, 10 mg of gel induced only 24% reduction in the fungal growth whereas 25 mg of gel produced a significant reduction (70%). 86 and 92% of killing was obtained with 50 and 100 mg of gel respectively. The results were shown in Fig. 6. This clearly indicated that the fluconazole loaded nanofiber containing antifungal gel has induced a dose dependent killing of the microbes in comparison to that of the control. The observation of agar plates inoculated with 10 μl of test solution from each concentration (Fig. 7) also depicted the efficiency of the formulation in killing the fungus in a dose dependent manner and confirmed the efficacy of the topical gel based nanofiber delivery system.
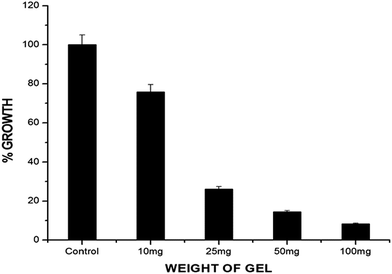 |
| Fig. 6 Turbidimetric growth analysis depicting the efficiency of gel containing drug loaded nanofibers (data were the mean ± SD of three measurements). | |
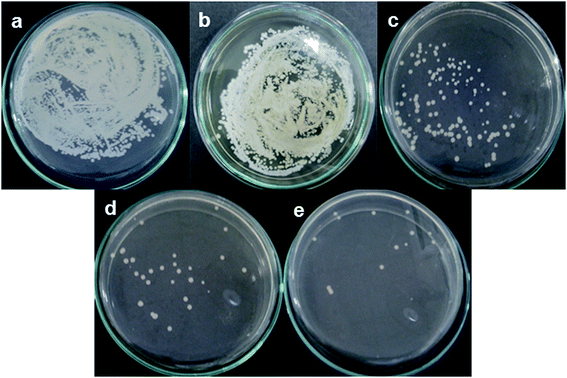 |
| Fig. 7 Agar plates depicting the dose dependent activity of nanofibers dispersed gel preparation. (a) Control (b) 10 mg (c) 25 mg (d) 50 mg and (e) 100 mg. | |
3.8. Live/dead assay
The live/dead assay revealed the cytocompatible behavior of the drug loaded eudragit nanofibers. The result was given in the Fig. 8c and it showed the cell friendly nature of the nanofibers. Moreover, staining of cells with AO/PI solution has also been carried out. AO is a cell permeable dye and it permeates in to the cell to give a green color. PI is a non-permeable dye and can enter cells only when the membrane gets damaged and reacts with the nucleus to give a reddish orange color. The fluorescent microscopic images of control and cells treated with drug loaded nanofibers were given in Fig. 8a and b. From the image, it was clear that the nanofiber does not induce any damage to the cells.
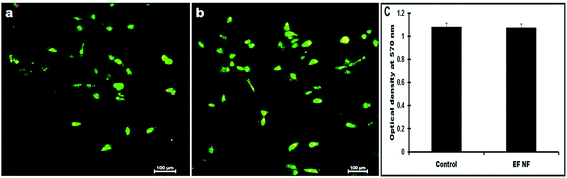 |
| Fig. 8 Fluorescent microscopic images of (a) control and (b) cells treated with drug loaded nanofibers. (c) Cell viability data depicting the non-toxic behavior of nanofibers. | |
The effect of fluconazole on the integrity of yeast cells was screened using acridine orange/propidium iodide molecular probes. Fluorescent microscopic observation (Fig. 9) revealed that the cell membrane integrity was disturbed in the drug loaded nanofiber treated cells as most of the cells produce an orange red fluorescence whereas the control cells were mostly green in color. As we discussed earlier, PI is a non-permeable dye and only if the cell membrane got damaged, the dye could penetrate the cell. From the image it was clear that the fluconazole present in the nanofibers induced the cell damage and hence the cells produce a red fluorescence whereas in the control group, ‘no’ such phenomenon occurred.
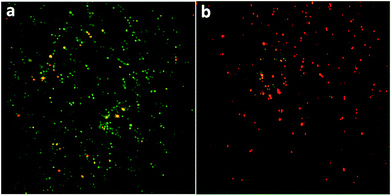 |
| Fig. 9 Fluorescent microscopic images of (a) control, predominantly showing green color and (b) cells treated with drug loaded nanofibers showing orange red fluorescence depicting cell membrane damage. | |
4. Conclusion
This current study depicted the development and application of a dosage form for the delivery of drug loaded polymeric nanofibers. A convenient dosage form for the administration of nanofibers has been developed. The topical gel based delivery system containing fluconazole loaded nanofibers has shown sufficient sustainability and successfully prevented the fungal growth. Moreover, this formulation helps to overcome the drawbacks currently available with the administration of nanofibers such as vehicle deployment, spreading and retention. Apart from this, it offers certain advantages such as ease of manufacture and application and hence, better patient compliance.
Acknowledgements
The first author is extremely grateful to the Council of Scientific and Industrial Research, New Delhi, for the award of senior research fellowship. We are grateful to Dr A. B. Mandal, Director, CSIR – Central Leather Research Institute for providing the encouragement and facilities to carry out this work. This work was supported by Council of Scientific and Industrial Research, New Delhi (ADD-CSC0302).
References
- T. J. Sill and H. A. Von Recum, Biomaterials, 2008, 29, 1989 CrossRef CAS PubMed.
- G. C. Rutledge and S. V. Fridrikh, Adv. Drug Delivery Rev., 2007, 59, 1384 CrossRef CAS PubMed.
- D. G. Yu, L. D. Gao, K. White, W. C. Brandford, W. Y. Lu and L. M. Zhu, Pharm. Res., 2010, 27, 2466 CrossRef CAS PubMed.
- D. G. Yu, L. M. Zhu, K. White and W. C. Brandford, Health, 2009, 1, 67 CrossRef.
- S. Yan and M. Xiumei, J. Controlled Release, 2011, 152, e82 CrossRef PubMed.
- S. Yan, S. Qianqian, L. Wei, J. Guori, M. Xiumei and R. Seeram, J. Biomater. Sci., Polym. Ed., 2012, 23, 861 CrossRef PubMed.
- M. H. Zheng, Y. Z. Zhang, M. Kotaki and R. Seeram, Compos. Sci. Technol., 2003, 69, 1804 Search PubMed.
- T. D. J. Heunis and L. M. T. Dicks, J. Biomed. Biotechnol., 2010, 510682 Search PubMed.
- C. Gandhimathi, J. R. Venugopal, V. Bhaarathy, S. Ramakrishna and S. D. Kumar, Int. J. Nanomed., 2014, 9, 4709 CAS.
- M. Naghibzadeh, Trends Biomater. Artif. Organs, 2012, 26, 86 Search PubMed.
- A. F. Azarbayjani, J. R. Venugopal, S. Ramakrishna, P. F. Lim, Y. W. Chan and S. Y. Chan, J. Pharm. Pharm. Sci., 2010, 13, 400 CAS.
- E. Shoba, R. Lakra, M. S. Kiran and P. S. Korrapati, RSC Adv., 2014, 4, 60209 RSC.
- K. B. Anna, B. Cameron, A. K. Emily and A. W. Kim, Antiviral Res., 2013, 100, S9 CrossRef PubMed.
- S. P. Kalpana, M. Mikolaj, L. S. Courtney, K. B. Nicole, G. Priyanka and L. S. Audra, Ther. Delivery, 2010, 1, 109 CrossRef.
- C. M. Klich, Encyclopedia of Pharmaceutical Technology, 1992, vol. 6, p. 415 Search PubMed.
- T. I. Mohammad, R. H. Naır, C. Susan and A. Chrisita, Pharm. Res., 2004, 21, 1192 CrossRef.
- D. Church, S. Elsayed, O. Reid, B. Winston and R. Lindsay, Clin. Microbiol. Rev., 2006, 19, 403 CrossRef PubMed.
- R. C. Malini, S. Sujata, K. T. Vinay and P. N. Ravi, Indian J. Plast. Surg., 2010, 43, S37 CrossRef PubMed.
- E. E. Horvath, C. K. Murray, G. M. Vaghan, K. K. Chung, D. R. Hospenthal, C. E. Wade, J. B. Holcomb, S. E. Wolf, A. D. Mason Jr and L. C. Cancio, Ann. Surg., 2007, 245, 978 CrossRef PubMed.
- B. P. Mathew and M. Nath, ChemMedChem, 2009, 4, 310 CrossRef CAS PubMed.
- M. F. Struck, Scand J. Trauma Resusc. Emerg. Med., 2009, 17, 51 CrossRef PubMed.
- J. M. Achkar and B. C. Fries, Clin. Microbiol. Rev., 2010, 23, 253 CrossRef PubMed.
- A. Del Palacio, M. Garau, E. A. Gonzalez and M. T. Calvo, Biology of Dermatophytes and other Keratinophilic Fungi, 2000, p. 148 Search PubMed.
- Y. Pavithra, R. G. S. V. Prasad, S. J. Venkata, R. S. L. Aparna and A. R. Phani, J. Pharm. Res., 2013, 6, 26 CrossRef PubMed.
- T. Mosmann, J. Immunol. Methods, 1983, 65, 55 CrossRef CAS.
- J. A. Lee and H. Y. Chee, Mycobiology, 2010, 38, 328 CrossRef PubMed.
- D. Church, S. Elsayed, O. Reid, B. Winston and R. Lindsay, Clin. Microbiol. Rev., 2006, 19, 403 CrossRef PubMed.
- H. Rode, D. Hanslo, P. M. de Wet, A. J. W. Millar and S. Cywes, Antimicrob. Agents Chemother., 1989, 33, 1358 CrossRef CAS.
- Z. Chen, X. Mo, C. He and H. Wang, Carbohydr. Polym., 2008, 72, 410 CrossRef CAS PubMed.
- X. Shen, D. Yu, L. Zhu, W. C. Branford, K. White and N. P. Chatterton, Int. J. Pharm., 2011, 408, 200 CrossRef CAS PubMed.
- K. Karthikeyan, S. Guhathakarta, R. Rajaram and P. S. Korrapati, Int. J. Pharm., 2012, 438, 117 CrossRef CAS PubMed.
|
This journal is © The Royal Society of Chemistry 2015 |