DOI:
10.1039/C4RA15359F
(Paper)
RSC Adv., 2015,
5, 15547-15558
Tadpole-shaped β-cyclodextrin-tagged poly(N-vinylpyrrolidone): synthesis, characterization and studies of its complexation with phenolphthalein and anti tumor activities†
Received
27th November 2014
, Accepted 26th January 2015
First published on 26th January 2015
Abstract
Tadpole-shaped β-cyclodextrin-tagged poly(N-vinylpyrrolidone) (β-CD-PNVP) has been synthesised via the click reaction of alkyne-terminated PNVP and azide-functionalized β-CD. The formed polymer is characterized by 1H NMR, FTIR, and TGA study. The complexing ability of such a polymer with phenolphthalein is considerably lower with respect to that with β-CD alone. Doxorubicin (DOX)-loaded β-CD-PNVP (DOX-β-CD-PNVP) exhibits higher tumoricidal activity against a panel of tumor cell lines derived from sarcoma (U2-OS) and carcinomas (MCF-7 and HEPG2), causing significant lysis of tumor cells compared to free DOX. The formulation also demonstrates significantly higher uptake by the tumor cells and enhances apoptosis compared to free DOX.
Introduction
Poly(N-vinylpyrrolidone) (PNVP) has several applications in chemical and medical fields due to its low toxicity, high water solubility, and biocompatibility properties etc.1,2 Different controlled/living radical polymerization methods were explored to synthesise well-defined PNVP and its block copolymers.3–14 Recently, we have reported the detailed study of the polymerization of N-vinylpyrrolidone(NVP) using two xanthates: (S)-2-(ethyl propionate)-(O-ethylxanthate) (X1) and (S)-2-(ethyl isobutyrate)-(O-ethylxanthate) (X2) [Scheme S1†].15 Very recently, we have also reported the synthesis and characterization of two new alkyne containing xanthate RAFT agents, namely, (S)-2-(propynyl propionate)-(O-ethyl xanthate) (X3) and (S)-2-(propynyl isobutyrate)-(O-ethyl xanthate) (X4) and studied the usefulness of the same for the controlled RAFT polymerization of NVP.16
Cyclodextrin (CD) also has several applications in medical and pharmaceutical fields due to its inclusion properties of hydrophobic moieties in its hydrophobic internal cavity.17–20 Synthesis of polymer containing the combined properties of PNVP and CD may find application as carrier for drug, catalyst or, light absorbers. Nielsen et al. reported the synthesis of water soluble random copolymers poly[NVP-co-acrylamidomethyl-β-cyclodextrin)] and the study of the formation of micro particles with controllable disruption properties by self-assembly of these polymers with hydrophobically modified dextran derivatives.21 Later Ren et al. reported the synthesis of random copolymers poly[NVP-co-(mono-6-deoxy-6-methacrylate ethylamino-β-cyclodextrin)] and the formation of soluble graft like polymer complexes with adamantane end-capped poly(ε-caprolactone).22 Recently, Trellenkamp and Ritter reported the synthesis of random copolymer poly[NVP-co-(3-(6-desoxy)-β-cyclodextrin-3H-1,2,3-triazole-4-yl)-N-vinylpyrrolidone)] and the study of the complexation ability of CD-functionalized PNVP with phenolphthalein indicator.23 So far, to our knowledge, there is no report about the synthesis of β-CD-tagged PNVP system. We anticipate that tagging of hydrophilic PNVP may increase the hydrophilicity of the β-CD and a micelle with core as β-CD and shell as hydrophilic PNVP may form due to their aggregation in water.24 Internal cavity part of β-CD present in the core part of such micelle may trap hydrophobic molecule or, drug. Thus, the hydrophilic surface of such micelles will show enhanced efficiency as a nano-carrier via the resistance of non-specific protein adsorption.25–27 Moreover, the tagged PNVP chain may also enhance the retention of the loaded drug in β-CD cavity through its wrapping over the β-CD. Here, we report the synthesis and characterization of covalently linked tadpole-shaped β-CD-tagged PNVP (β-CD-PNVP) via the click reaction of alkyne-terminated PNVP and azide-functionalized β-CD and the comparison of the studies of the complexing ability of this β-CD-tagged PNVP and β-CD itself with phenolphthalein. Moreover, we have formulated the doxorubicin-loaded β-CD-PNVP (DOX-β-CD-PNVP) and explored its antitumor activities in comparison to doxorubicin drug itself. DOX-β-CD-PNVP reduces the viability of MCF-7 (breast adenocarcinoma), U2-OS (osteosarcoma), and HEPG2 (liver carcinoma) tumor cells in a dose dependent manner. It also directly kills these tumor cells significantly higher compared to free doxorubicin in a concentration dependent manner. Besides that it significantly restricts the proliferation of these cell lines. It shows significantly lower IC50 compared to free doxorubicin for antitumor effect in these cell lines. Uptake of it by the tumor cells, specifically in HEPG2, is found to be significantly higher compared to free doxorubicin. It also enhances the apoptosis of treated cell compared to free doxorubicin with the better uptake.
Experimental
Materials
β-Cyclodextrin (99%, Spectrochem, Mumbai, India), p-toluenesulfonyl chloride (98%, Spectrochem, Mumbai, India), and phenolphthalein (98%, CDH, India), 2-bromopropionyl bromide (BPB, >97%, Fluka, Israel), carbon disulfide (CS2, Loba Chemie, India), diethyl ether (s.d.fine, India), hexane (CDH, India), ethyl acetate (CDH, India), dichloromethane (CDH, India), sodium bicarbonate (NaHCO3, Loba Chemie, India), sodium carbonate (Na2CO3, Loba Chemie, India), potassium hydroxide (KOH, Qualigens, India), sodium hydroxide (97%, Rankem, India), anhydrous magnesium sulphate (MgSO4, Loba Chemie, India), sodium azide (99%, Loba Chemie, Mumbai, India), propargyl alcohol (Loba Chemie, Mumbai, India), triethyl amine (TEA) (99%, Loba Chemie, Mumbai, India), doxorubicin (DOX, Sellecxchem, USA) were used as received. N,N-Dimethyl formamide (DMF) (99.5%, Spectrochem, Mumbai, India) was distilled over anhydrous magnesium sulphate. Cuprous bromide (CuBr, 98%, Aldrich, Steinheim, Germany) was purified using a reported procedure and dried under vacuum.28 N-Vinylpyrrolidone (99%, Aldrich, St Louis, USA) was dried over anhydrous calcium hydride and distilled under reduced pressure as reported earlier.28 Water was used after re-distillation of double distilled water in all glass apparatus.
RPMI 1640, penicillin and streptomycin were purchased from GIBCO, Invitrogen, and Carlsbad, CA and fetal bovine serum was purchased from Hyclone, Logan, UT. Annexin V Apoptosis Detection Kit (sc-4252 AK) was purchased from Santa Cruz Biotechnology, Dallas, TX, U. S. A. Other general and fine chemicals unless otherwise stated were purchased from Sigma-Aldrich, St. Louis, MO, USA.
General methods
1H NMR of samples was performed using JEOL AL300 FTNMR (300 MHz) at ambient temperature in CDCl3 and DMSO-d6. Fourier Transform Infra Red (FTIR) spectra were recorded within range 400–4000 cm−1 using Perkin-Elmer Spectrum Version 10.03.05 by making the pellets of compound with KBr. Complexation constants of phenolphthalein and β-CD or, β-CD-tagged PNVP were determined using a JASCO V-670 UV-visible spectrophotometer at room temperature using a quartz shell having 1 cm path length. For the measurement, six solutions of 4 mL each were prepared by mixing homogeneously 2 mL of 100 μM buffered phenolphthalein (PP) solution at pH = 10 (NaHCO3 and Na2CO3) with 2 mL of six different concentrations (0 μM, 100 μM, 200 μM, 400 μM, 800 μM, 1600 μM) of buffered β-CD or, β-CD-tagged PNVP at pH = 10. The complexation constant (K)s were determined from the spectroscopic data using Scott's equation assuming the formation of 1
:
1 complex of β-CD and PP:
where, [β-CD] = total molar concentration of β-CD, [PP] = total molar concentration of PP, ΔA552nm = change in absorbance at λ = 552 nm after addition of β-CD or, β-CD-tagged PNVP solution = (A552nm)PP − (A552nm)β-CD/β-CD-PNVP, and Δε = difference in the molar absorption co-efficient of free and complex PP, Ks are calculated from the slope and intercept of the plots of [β-CD]/ΔA552nm vs. [β-CD].
Ks are also calculated from the law of the mass action of the complexation equilibrium:
β-CD (β-CD-PNVP) + PP ⇌ β-CD-PP complex (β-CD-PNVP-PP complex) |
where, [PP]
o = total concentration of PP, [β-CD]
o = total concentration of β-CD/β-CD-tagged PNVP, and [PP]
f = concentration of free (un-complexed) PP determined from the PP calibration curve.
Thermo gravimetric analysis (TGA) was performed using Perkin Elmer-STA 6000 thermo gravimetric analyzer in the temperature range from 30 °C to 800 °C with a heating rate of 10 °C min−1 under N2 atmosphere.
Dynamic Light Scattering (Malvern Zetasizer Ver. 7.01 Serial number: MAL1077742) study was performed to determine the hydrodynamic size (diameter, d) of the aggregated polymers and DOX-loaded aggregated polymers using 0.1 mg mL−1 solution in water at 90° angle.
Synthesis of mono-(6-O-(p-tolylsulfonyl))-β-cyclodextrin
Mono-(6-O-(p-tolylsulfonyl))-β-cyclodextrin was synthesised according to the literature procedure.23 Briefly, β-CD (1.0 g, 0.88 mmol) was dissolved in 10 mL of a 0.4 M aqueous sodium hydroxide solution and cooled down to 0 °C. To it, p-toluenesulfonyl chloride (0.7 g, 3.67 mmol) was added in small portions under vigorous stirring over 5 min. Formed suspension was kept under stirring at 5 °C for 30 min and then filtered off immediately. The filtrate was neutralized with hydrochloric acid and stirred for 1 h. The resultant precipitate was filtered off, washed three times with water and dried under vacuum at 60 °C for 20 h. Yield: 0.33 g (29%).1H NMR [Fig. 1a] (300 MHz, DMSO-d6): δ (ppm) 2.45 (3H, Ar-CH3); 3.18–3.78 (42H, 7Ha,a′ + 7Hb,b′ + 7Hc,c′ + 7Hd,d′ + 14He,e′); 4.79 (t, 6H, 7Hf,f′); 5.74 (14H, 7Hd-OH + 7He-OH); 7.45 (d, 2Hh); 7.77 (d, 2Hh). FTIR (KBr): ν = 3394 (O–H stretching), 2929 (C–H stretching), 1359 (S
O stretching), 1176 (S–O stretching), 1034 (C–O stretching) cm−1.
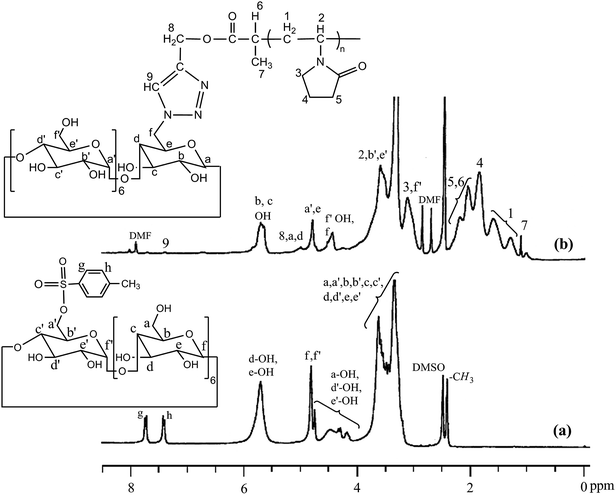 |
| Fig. 1 1H NMR spectra of (a) mono(6-O-(p-tolylsulfonyl))-β-cyclodextrin and (b) β-cyclodextrin-tagged PNVP in DMSO-d6. | |
Synthesis of mono-(6-azido-6-desoxy)-β-cyclodextrin
Mono-(6-azido-6-desoxy)-β-cyclodextrin was synthesised according to the literature procedure.23 Briefly, mono-(6-O-(p-tolylsulfonyl))-β-cyclodextrin (0.275 g, 0.24 mmol) suspended in water (10 mL) was heated to 80 °C. To this suspension, sodium azide (2.53 g, 38.5 mmol) was added and stirred for 4 h until the reaction mixture become transparent. Resulting solution was precipitated in acetone (80 mL) and the white solid was filtered off and dried under vacuum for 20 h yielding 0.17 g (69%) crude product. Product was purified by re-crystallization from water/acetone (1/1, v/v) yielding 0.145 g (58%). 1H NMR (300 MHz, DMSO-d6): δ (ppm) 3.32 (m, 14H, C6 + C6′); 3.60–3.84 (m, 28H, C2 + C2′ + C3 + C3′ + C4 + C4′ + C5 + C5′); 4.44–4.60 (m, 6H, C6-OH); 4.85 (d, 6H, C1); 4.92 (d, 1H, C1′); 5.73 (m, 14H, C2-OH + C2′-OH + C3-OH + C3′-OH) ppm. FTIR (KBr): ν (cm−1) = 3394 (O–H stretching), 2928 (C–H stretching), 2106 (N–N stretching), 2041 (OH), 1155 (C–N stretching).
Synthesis of O-propynyl-2-bromopropionate
O-Propynyl-2-bromopropionate was synthesized according to the literature procedure.16 Briefly, a solution of propargyl alcohol (4 mL, 3.9 g, 0.07 mol) and pyridine (5.6 mL, 5.4 g, 0.07 mol) in dry THF (30 mL) was added drop wise to a solution of 2-bromopropionyl bromide (5 mL, 10.2 g, 0.05 mol) in dry THF (30 mL) cooled at 0 °C under nitrogen. The reaction mixture was allowed to warm to room temperature and stirred overnight. The product mixture was washed consecutively with 5% (w/v) aqueous NaHCO3 solution (3 × 75 mL), 8.5% (v/v) aqueous HCl solution (2 × 50 mL), saturated aqueous brine (50 mL) solution, and distilled water (2 × 50 mL). The organic layer was dried over anhydrous MgSO4, filtered, and concentrated. Final purification of the ester was done by distillation and yield was 5.5 g (56%). 1H NMR (300 MHz, CDCl3): δ (ppm) 1.84 (d, 3H), 2.52 (s, 1H), 4.39 (q, 1H), 4.76 (s, 2H); 13C NMR (75 MHz, CDCl3): δ (ppm) 21.5, 39.1, 53.3, 75.6, 77.4, and 169.4. IR (neat): 3290 (
C–H stretching), 2980 and 2930 (C–H stretching), 2130 (C
C stretching), 1745 (C
O stretching) cm−1.
Synthesis of (S)-2-(propynyl propionate)-(O-ethyl xanthate) (X3)
(S)-2-(Propynyl propionate)-(O-ethyl xanthate) (X3) was synthesised according to the literature procedure.16 Briefly, a solution of O-propynyl-2-bromopropionate (3.5 g, 0.02 mol) in dry ethanol (20 mL) was added to a round-bottom flask already containing potassium O-ethyl xanthate (3 g, 0.02 mol), and stirred for 24 h at room temperature. The KBr so formed was filtered off and the filtrate, after dilution with diethyl ether (250 mL), was washed three times with deionised water (50 mL). The resulted diethyl ether solution was dried over anhydrous MgSO4, filtered, and solvent was evaporated to dryness. The yellow color liquid product was purified by column chromatography using hexane/ethyl acetate (95
:
5 v/v) as the eluent (Rf value = 0.71). A yellow colored oily product (40% yield by NMR) was obtained. 1H NMR (300 MHz, CDCl3): δ (ppm) 1.4 (t, 3H, C(S)-OCH2CH3), 1.57 (d, 3H, CH3CH), 2.49 (s, 1H, –CH2C(CH)), 4.42 (q, 1H, C(CH3)CH), 4.62 (q, 2H, C(S)OCH2), 4.73 (s, 2H, –CH2C(CH)). 13C NMR (75 MHz, CDCl3): δ (ppm) 13.6, 16.6, 46.7, 53.0, 70.3, 75.3, 77.4, 170.7, 211.6. IR (neat): 3290 (
C–H stretching), 2980 and 2930 (C–H stretching), 2130, 1745 cm−1.
Synthesis of alkyne-terminated poly(NVP) (PNVP)
Alkyne-terminated poly(NVP) was synthesised according to the literature procedure.16 Briefly, NVP (12 mL, 12.48 g, 112.3 mmol), X3 (130.8 mg, 0.560 mmol), and AIBN (18.6 mg, 0.113 mmol) were placed in a dry Schlenck tube containing a Teflon-coated magnetic bar, and was purged with dry N2 gas for 45 min. The polymerization tube was then placed in a thermostated bath preheated at 60 °C for 5 h. The reaction was stopped by dipping the Schlenck tube in liquid N2. The polymer was purified by repeated dissolution in THF (5 mL) and precipitation from hexane (200 mL) thrice and finally was dried under vacuum at 50 °C for 12 h. The Mn (GPC) and PDI of the obtained polymer were observed 4000 g mol−1 and 1.43, respectively, using PMMA standards.
Synthesis of β-CD-tagged PNVP
Mono-(6-azido-6-desoxy)-β-cyclodextrin (0.1 g, 0.086 mmol) and alkyne-terminated PNVP [Mn (GPC) = 4000 g mol−1, PDI = 1.43] (0.11 g, 0.0273 mmol) was dissolved in dry DMF (3 mL) taken in a dry Schlenck tube containing a Teflon-coated magnetic bar. Nitrogen was purged to the solution for 30 min and then CuBr (12.4 mg, 0.086 mmol) was added and nitrogen was purged for additional 5 min. The polymerization tube was then placed in a thermostated bath at 80 °C for 24 h. The reaction mixture was precipitated in 100 mL dry acetone. Precipitate was collected by centrifugation. Yield = 160 mg (76%). 1H NMR [Fig. 1b] (300 MHz, DMSO-d6): δ (ppm) 1.31 (3H7); 1.18–1.73 (2H1); 1.87 (2H4); 2.40–2.00 (2H5); 3.14 (12Hf′ + 2H3); 3.92–3.47 (6Hb′ + 6He′ + 1H2); 4.46 (6Hf′-OH + 2Hf); 4.81 (6Ha′ + 1He); 5.07 (2H8 + 1Ha + 1Hd); 5.60–5.82 (1Hc-OH + 1Hb-OH); FTIR (KBr): ν (cm−1) = 3420 (O–H and N–H stretching); 3255 (triazole ring C–H stretching), 2926 (C–H stretching), 2041 (N–N stretching), 1655 (NC
O stretching), 1292 (N–CO stretching).
Drug loading
30 mg of β-CD-tagged PNVP was dissolved in 2 mL of DMF, and of DOX (6 mg, 0.011 mmol) and TEA (4.6 μL, 0.033 mmol) were added into the polymer solution. The mixture was stirred at room temperature for 24 h. The final mixture was then dialyzed against distilled water using a dialysis membrane [molecular weight cut off (MWCO) = 3500 g mol−1] for 12 h. During the first 5 h, the water was replaced every 1 h and then every 3 h during the following 7 h for removing unloaded drug. Dialyzed drug-loaded β-CD-tagged PNVP solution was finally lyophilized. DMF solution of the lyophilized drug-loaded β-CD-tagged PNVP was then analyzed by UV absorbance at 485 nm using a standard calibration plot experimentally obtained from different DOX/DMF solutions. Drug loading content (DLC) and drug loading efficiency (DLE) were calculated according to the following formula:
Drug loading content (DLC) (wt%) = (weight of loaded drug/weight of polymer) × 100% |
Drug loading efficiency (DLE) (wt%) = (weight of loaded drug/weight of the drug in the feed) × 100% |
In vitro drug release study
5 mg of DOX-loaded β-CD-tagged PNVP was dissolved in 1.0 mL PBS (pH = 7.4/6.4) solution and transferred into the dialysis tubing (MWCO: 3500 g mol−1). The tubing was placed into 20 mL PBS (pH = 7.4/6.4) solution. The system was maintained under stirring at 37 °C and at predetermined interval, 2.0 mL PBS solution mixture was taken out and the volume of solution was held constant by adding 2.0 mL PBS (pH = 7.4/6.4) solution after each sampling. The amount of DOX released from micelles was quantified by UV absorbance at 485 nm on comparison with the standard calibration plot.
Cell lines and cell culture
Human breast adenocarcinoma MCF-7, osteosarcoma U2-OS and hepatocellular carcinoma HEPG2 were purchased from American Type Culture Collection (ATCC), Manahass, USA. The cells were maintained in RPMI 1640 (Invitrogen, Carlsbad, CA), supplemented with 10% fetal bovine serum (Hyclone, Logan, UT), 100 U mL−1 penicillin and 100 μg mL−1 streptomycin (Invitrogen, Carlsbad, CA), henceforth, called as complete medium. The cell lines used in the study were free from mycoplasma.
In vitro cell viability assay
Effect of free DOX or, DOX-loaded β-cyclodextrin-tagged poly(N-vinylpyrrolidone) (DOX-β-CD-PNVP) on the viability of tumor cells was evaluated by a colorimetric XTT (sodium 3′-[1-(phenylaminocarbonyl)-3,4-tetrazolium]-bis(4-methoxy-6-nitro) benzene sulfonic acid hydrate) assay (Roche Molecular Biochemicals, Indianapolis, USA). Tumor cells were plated (5 × 103 cells per well) in a 96-well plate and allowed to become confluent by culturing 3 days. The cells were exposed to serial concentrations (0.1, 0.5, 1, 5, and 10 μM) of free DOX or, DOX-β-CD-PNVP, and incubated at 37 °C, 5% CO2, for 18 h. Absorbance (Optical density, OD) was measured at 450 nm using Synergy HT Multi-Mode Micro plate Reader BioTek, USA.29 The data was presented as the percentage of viable cell calculated from the following formula:
In vitro cytotoxicity assay
The lytic activity of DOX-β-CD-PNVP against tumor cells was measured by non-radioactive cytotoxicity assay using the CytoTox 96 Non-Radioactive Cytotoxicity assay kit from Promega, USA.30 Target cells (5 × 103) were added to 96-well tissue culture plate allowed to become confluent and exposed to serial concentrations (0.1, 0.5, 1, 5, and 10 μM) of free DOX, β-CD-PNVP or, DOX-β-CD-PNVP and incubated for 18 h at 37 °C, 5% CO2. Percent-specific lysis was determined using the following formula:
Tumor cell proliferation assay
Growth inhibitory potential of free DOX or, DOX-β-CD-PNVP against MCF-7, U2-OS or, HEPG2 cells was studied by MTT assay. In a 96-well tissue culture plate, 5 × 103 cells per well were added and allowed to become confluent and exposed to serial concentrations (50, 100 and 200 nM) of free DOX, β-CD-PNVP or, DOX-β-CD-PNVP. Plates were incubated at 37 °C, 5% CO2 for 48 h. The cell proliferation was measured by CellTiter 96 Non-Radioactive Cell Proliferation Assay (MTT) kit from Promega, USA. The data presented as the percentage of inhibition of tumor cells and was calculated from the following formula:
where, experimental OD value is the reading of tumor cells exposed to various concentrations of MTX and target OD value is the value of tumor cell only cultured in the absence of the drug.31
Detection of apoptosis
Apoptotic cell death in MCF-7, U2-OS or, HEPG2 cells by free DOX, β-CD-PNVP or, DOX-β-CD-PNVP was assessed by binding FITC-conjugated Annexin V (Sanata Cruz Biotechnology, USA). After 6 h of incubation, apoptotic cells were analyzed by staining with FITC-conjugated Annexin V for 20 min in ice-cold PBS. Cells were washed in Annexin buffer and a drop of mounting medium was added in order to reduce fluorescence photo bleaching. The FITC-conjugated Annexin V positive cells were visualized under EVOS® FL Cell Imaging System (Life Technologies, USA).
Fluorescence microscopy for doxorubicin uptake
MCF-7, U2-OS or, HEPG2 cells were plated in a 24 well plates at concentration of 5 × 103 cells per well. The cells were allowed to adhere and cultured for 3 days in order to become confluent. Cells were washed twice with PBS and incubated with free doxorubicin or, β-CD-tagged PNVP with equivalent concentration of doxorubicin (5.0 mM) in complete medium for 4 h at 37 °C. Cells were washed twice with ice-cold PBS and fixed with freshly prepared 2% (w/w) paraformaldehyde for 15 min at room temperature. The cells were counterstained with Hoechst stain (for nucleus staining) and with a drop of mounting media to reduce fluorescence photobleaching. The intracellular DOX localization was visualized under the fluorescence microscope EVOS® FL Cell Imaging System (Life Technologies, USA). Image analysis and merging were performed by using Image-Pro Plus AMS analysis software (Media Cybernetics, Inc. 401 N. Washington Street, Suite 350 Rockville, MD 20850 USA).
Statistical analysis
In this study, n reflects the number of times experiments were performed independently in triplicate. The mean ± SD were calculated for each experimental group (n = 3–5). Differences between groups were analyzed by unpaired Student's t-test and one- or, two-way ANOVA analysis of variance depending on the requirement. One or, two-way ANOVA followed by Holm-Sidak post hoc multiple comparison tests was used to conduct pair wise comparisons using PRISM statistical analysis software (Graph Pad Software, Inc., San Diego, CA, USA). Significant differences among groups were calculated at P < 0.05 or less. The statistical significance of survival differences for the mice in different groups was determined by the log-rank test using Graph Pad PRISM software.
Results and discussion
Synthesis of β-cyclodextrin-tagged poly(N-vinylpyrrolidone) (β-CD-PNVP)
Synthesis of β-CD-tagged PNVP (β-CD-PNVP) was performed via the click reaction of the azide-functionalized β-CD and the alkyne-terminated PNVP (Scheme 1). At first, β-CD was reacted with p-toluene sulfonyl chloride in basic medium at 5 °C to synthesis mono-(6-O-(p-tolylsulfonyl))-β-cyclodextrin with 29% yield (Fig. 1a). Resultant mono-(6-O-(p-tolylsulfonyl))-β-cyclodextrin was then reacted with sodium azide to synthesis its corresponding azide, mono-(6-azido-6-desoxy)-β-cyclodextrin with 69% yield (Scheme 1). Alkyne-terminated PNVP was prepared by the RAFT polymerization of NVP using X3 as RAFT agent in the presence of AIBN as initiator at 60 °C. Observed number average molecular weight (Mn) and polydispersity (PDI) of the prepared polymer are 4000 g mol−1 and 1.43, respectively.
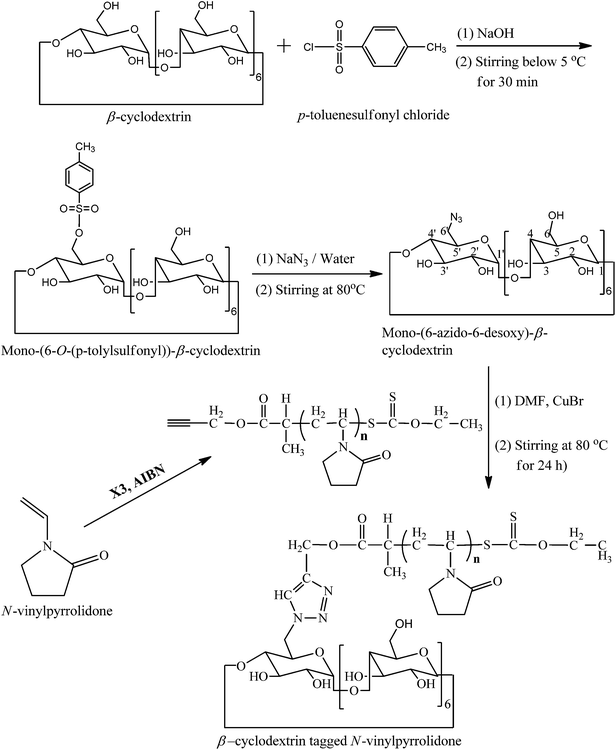 |
| Scheme 1 Synthesis of β-cyclodextrin-tagged PNVP via click chemistry. | |
Click reaction was then performed between the mono-(6-azido-6-desoxy)-β-cyclodextrin and the alkyne-terminated PNVP in the presence of CuBr in DMF at 80 °C. Resultant β-CD-tagged PNVP (β-CD-PNVP) has the molecular weight (Mn = 5100 g mol−1, PDI = 1.46) close to the parent alkyne-terminated polymer. Conjugation of β-CD to PNVP is confirmed by the appearance of the triazole proton peak at ∼7.35 ppm apart from the other characteristic peaks of β-CD and PNVP in its 1H NMR spectrum (Fig. 1b). This is also supported by the presence of characteristics peaks at 3255 cm−1 for triazole group apart from the characteristics peaks of PNVP and CD and also by the absence of characteristics peaks of alkyne and azide functional group at 2121 and 2100 cm−1, respectively in its FTIR spectrum (Fig. 2).
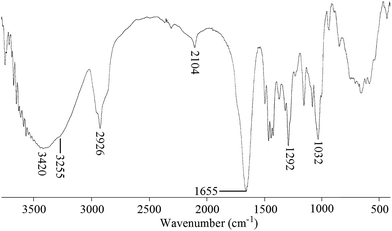 |
| Fig. 2 FTIR spectrum of β-cyclodextrin-tagged PNVP in KBr matrix. | |
Fig. 3 shows the TGA curves of β-CD, PNVP and β-CD-tagged PNVP. The observed initial weight loss for all compounds up to 124 °C is due to the evaporation of moisture from the compounds. PNVP shows two steps degradation: firstly, from 170 °C to 315 °C is due to loss of pyrrolidone ring and secondly, from 315 °C to 490 °C is due to NVP backbone chain loss.32 β-CD shows the major degradation in the temperature range 265 °C to 400 °C associated with ∼66% weight loss due to decomposition of β-CD ring with formation of char.33 After 400 °C, β-CD shows very slow degradation of char. β-CD-tagged PNVP clearly shows three steps degradation. The observed first ∼23.5% weight loss in the temperature range from 215 °C to 315 °C is due to the decomposition of pyrrolidone ring in β-CD-tagged PNVP. The observed second ∼42% of weight loss occurred in the range from 315 °C to 490 °C owing to the decompositions of both the backbone chain of PNVP block and β-CD ring in β-CD-tagged PNVP. Lastly, the observed degradation from 480 °C to 720 °C belongs to the thermal oxidation of β-CD.33 There is no char degradation was found in the case of β-CD-tagged PNVP degradation and no residue was left during the degradation of β-CD-tagged PNVP. All these results support the formation of β-CD-tagged PNVP.
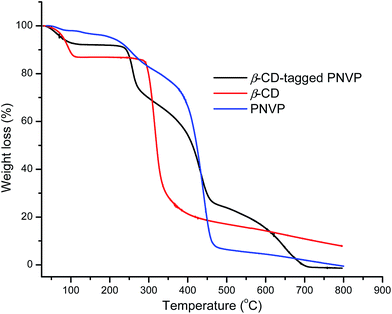 |
| Fig. 3 TGA curves of β-CD, PNVP and β-CD-tagged PNVP. | |
Complexing ability of β-CD and β-CD-tagged PNVP (β-CD-PNVP) with phenolphthalein
Complexing ability of β-CD and β-CD-tagged PNVP (β-CD-PNVP) with phenolphthalein was verified using UV-visible spectroscopy. Complexation constant (K) was calculated using both Scott's equation and law of mass action of the complexation equilibrium. UV-visible spectra of the mixtures of buffered PP solution and the different concentration of β-CD/β-CD-PNVP are shown in Fig. S1(a) and (b).† There is a gradual decrease in absorbance with the increase in the β-CD concentration. The corresponding Scott's plots are shown in Fig. 4a and (b). Linearity of these plots confirms the formation of 1
:
1 complex of PP and β-CD. Calculated K values for β-CD-PP and β-CD-PNVP-PP complex system are 13
280 and 5586 M−1, respectively. Using the similar method, Trellenkamp and Ritter also reported the K value of 13
100 M−1 for β-CD-PP complexation system.23 So our result is comparable to their result for this system. But our observed considerably low value of K for β-CD-PNVP-PP system may be due to the wrapping of long PNVP chain around the β-CD preventing the complexation with PP. Calculated K values from the above mentioned complexation reactions involving 50 μM buffered PP and different concentrations of buffered β-CD or β-CD-PNVP using the PP calibration curve (Fig. 5) are shown in Table 1. Average K values for β-CD-PP and β-CD-PNVP-PP complex system using mass action law are 21
500 and 7718 M−1, respectively. Trends are similar as observed for the results obtained using the Scott's equation. Therefore, the complexing ability of phenolphthalein with β-CD-PNVP is lower than that with the β-CD.
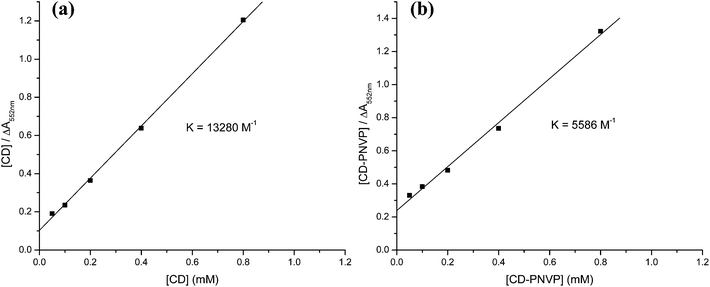 |
| Fig. 4 The plot between [β-CD] vs. [β-CD]/ΔA552nm (Scott's equation) for (a) β-CD and (b) β-CD-tagged PNVP. | |
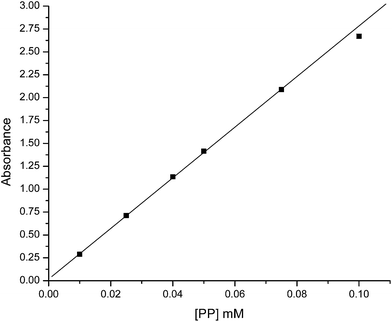 |
| Fig. 5 Calibration curve of phenolphthalein (PP) for determination of KUV-vis by law of mass action. | |
Table 1 Binding constant determined by law of mass actiona
[β-CD]o/[β-CD-PNVP]o (μM) |
KUV-vis [law of mass action] (M−1) Kβ-CD/Kβ-CD-PNVP |
In all measurement concentration of phenolphthalein [PP]o = 50 μM. |
50 |
17 578/6250 |
100 |
21 428/6860 |
200 |
22 021/8075 |
400 |
23 737/9154 |
800 |
22 737/8252 |
Average |
21 500/7718 |
Loading of DOX in β-cyclodextrin-tagged poly(N-vinylpyrrolidone) (β-CD-PNVP)and its release study
In order to check the usefulness of the β-CD-PNVP for drug delivery, we have loaded the FDI approved anticancer drug doxorubicin (DOX) in β-CD-tagged PNVP. The observed drug loading content (DLC) and drug loading efficiency (DLE) are 10.1 and 52.5%, respectively. Loading of the drug is evidenced from the increase in the hydrodynamic volume (diameter) of β-CD-PNVP from 162.0 nm (PDI = 0.455) to that of the DOX-loaded β-CD-PNVP of 192 nm (PDI = 0.114) as observed in the dynamic light scattering (DLS) study (Fig. 6). Similar order hydrodynamic diameter (173.4 nm) for the blank micelles of CD-tagged poly(lactic acid)-b-poly(ethylene glycol) is also reported in the literature.20 In vitro drug release study was carried out at 37 °C in PBS solutions having pHs of 7.4 and 6.4 (Fig. 7). The corresponding release profiles show the sustained release behaviour up to ∼25 and 48%, respectively.
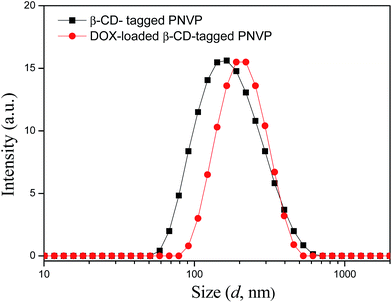 |
| Fig. 6 Plot of scattering intensity vs. the effective hydrodynamic diameter of without and with DOX drug loaded β-CD-tagged PNVP at 0.1 mg mL−1 concentration in water at 90° scattering angle. | |
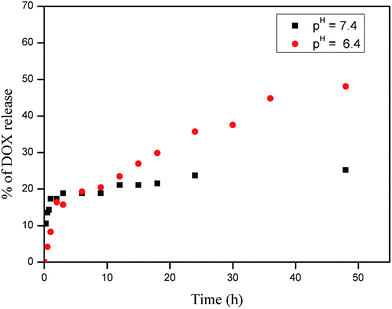 |
| Fig. 7 In vitro release profiles of DOX from DOX-loaded β-CD-tagged PNVP in PBS (pH = 7.4 and 6.4) at 37 °C (error ± 1–2%). | |
Effect of DOX-loaded β-cyclodextrin-tagged PNVP (DOX-β-CD-PNVP) on tumor cell viability and cytotoxicity
We have performed cell viability and cellular cytotoxicity study for the free DOX, β-CD-tagged PNVP(β-CD-PNVP) and DOX-loaded β-CD-tagged PNVP (DOX-β-CD-PNVP) against MCF-7, U2-OS and HEPG2 (Fig. 8A–C) cancer cell lines. Compared to free DOX, DOX-β-CD-PNVP significantly inhibits the cell viability in a concentration dependent manner in all the cell lines tested. HEPG2 responds better compared to other two cell lines possibly because DOX is one of the effective drug in hepatocellular carcinoma. This was evident by the greater loss of cell viability in the presence of DOX-β-CD-PNVP compared to free DOX alone (Fig. 8C). β-CD-PNVP itself has some mild effect but is significantly less compared to DOX-β-CD-PNVP. Loss of cell viability possibly correlated with the increased susceptibility of tumor cells against DOX-β-CD-PNVP with respect to cellular cytotoxicity (Fig. 8D and E). Our results suggest that DOX-β-CD-PNVP is highly cytotoxic compared to free DOX at each molar concentration tested. β-CD-PNVP itself does not show cytotoxicity against any of the cell lines tested.
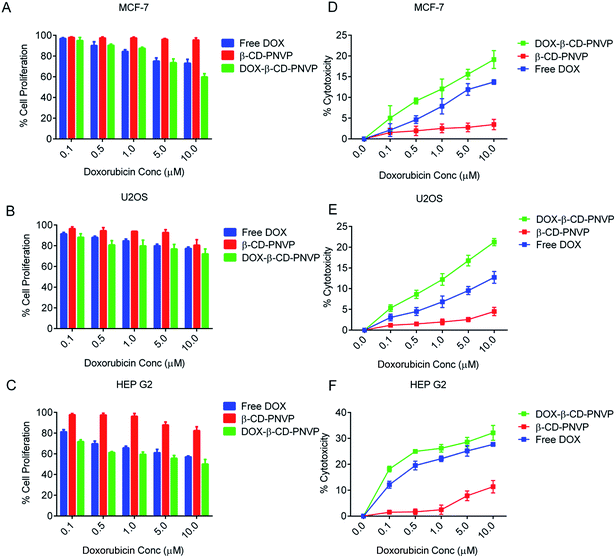 |
| Fig. 8 Killing of tumor cells by free DOX, β-cyclodextrin-tagged poly(N-vinylpyrrolidone) (β-CD-PNVP) and DOX-loaded β-CD-PNVP (DOX-β-CD-PNVP). (A–C) Viability of MCF-7, U2-OS and HEPG2 cells were studied following XTT viability assay (Promega, USA). 5 × 103 cells were plated in 96 well plates, and were cultured for 3 days for confluence. The cells were treated in complete medium in presence free DOX, β-CD-PNVP or DOX-β-CD-PNVP for 24 h at 37 °C, 5% CO2. The percent viability was calculated by considering the viability of tumor cell cultured in complete medium only as 100%. Cytotoxicity of free DOX, β-CD-PNVP and DOX-β-CD-PNVP against MCF-7, U2-OS and HEPG2 (D–F) were determined by 18 h LDH release assay. Cells were incubated with free DOX, β-CD-PNVP, or DOX-β-CD-PNVP at different concentrations for 18 h followed by measurement of the released LDH according to the manufacture's protocol. Data presented are the mean ± SD of triplicate determinations of n = 4 where n represents the number of times experiment performed. | |
Cellular growth inhibition by DOX-loaded β-cyclodextrin-tagged PNVP (DOX-β-CD-PNVP)
The cell viability and cytotoxicity data suggest that DOX-β-CD-PNVP has significantly higher anti-tumor potential with respect to the reduction in the viability of sarcoma and carcinomas of human origin. DOX-β-CD-PNVP shows significant growth inhibition against MCF-7, U2-OS and HEPG2 cancer cell lines, suggesting broad spectrum usefulness of the compound (Fig. 9A–C). Growth inhibition in HEPG2 was very significant and dramatic compared to MCF-7 or, U2-OS. The corresponding IC50 of free DOX for HEPG2 is ten times higher compared to DOX-β-CD-PNVP (Fig. 9C).
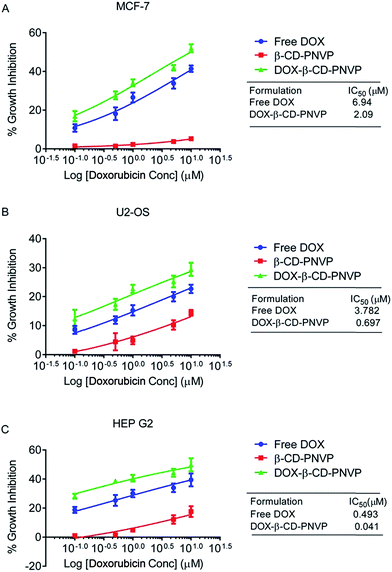 |
| Fig. 9 Inhibition of the growth of the tumor cells MCF-7 (A), U2-OS (B) and HEPG2 (C) by free DOX, β-cyclodextrin-tagged poly(N-vinylpyrrolidone) (β-CD-PNVP) and DOX-loaded β-CD-PNVP(DOX-β-CD-PNVP) as a function of concentration. Data presented are the mean ± SD of triplicate determination of n = 3, where n represents the number of times experiment performed. Differences in IC50 values in cell lines are also mentioned. | |
Induction of tumor cell apoptosis by DOX-loaded β-cyclodextrin-tagged PNVP (DOX-β-CD-PNVP)
Broad spectrum growth inhibition by DOX-β-CD-PNVP raises the question whether it also causes apoptosis of tumor cells, and if so, whether it induces cell death in tumor cells. Apoptosis was determined by monitoring changes in cell size and externalization of phosphatidylserine qualitatively in MCF-7 (Fig. 10A), U2-OS (Fig. 10B) and HEPG2 (Fig. 10C) to document the events of apoptosis. There was an abundance of Annexin V positive cells in all the cell lines tested upon treatment with DOX-β-CD-PNVP compared to free DOX.
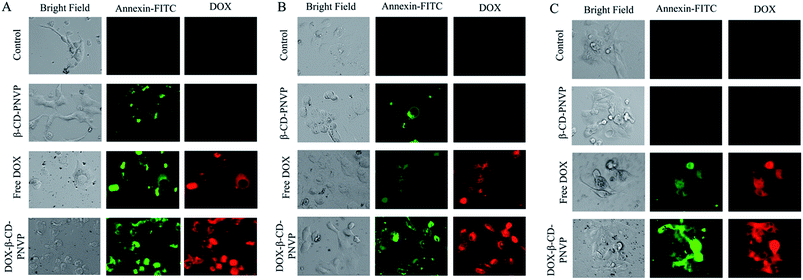 |
| Fig. 10 Microscopic analysis of the induction of apoptosis in MCF-7 (A), U2-OS (B) and HEPG2 (C). Cells were treated with free DOX, or, DOX-β-CD-PNVP having equivalent DOX concentration of 5.0 mM in complete RPMI 1640 medium for 8 h at 37 °C. The FITC-conjugated Annexin V positive cell apoptotic cells were visualized under the fluorescence microscope EVOS® FL Cell Imaging System (Life Technologies, USA). Representative images shown were obtained with green and red filters for FITC and DOX, respectively. n = 4, where n represents the number of times experiment performed. | |
Cellular uptake studies
We then qualitatively studied the intracellular DOX concentration in MCF-7 (Fig. 11A), U2-OS (Fig. 11B) and HEPG2 (Fig. 11C) following incubation with free DOX, β-CD-PNVP or, DOX-β-CD-PNVP by microscopic analysis. It is clear from the figure that (i) the DOX incorporation is significantly higher following the treatment with DOX-β-CD-PNVP in all the cell lines tested, (ii) DOX-uptake from DOX-β-CD-PNVP compared to free DOX at microscopic level is significantly higher in all the cell lines tested, (iii) Intracellular DOX concentration in HEPG2 is particularly significantly higher compared to MCF-7 or, U2-OS. Overall, the observed significantly higher intracellular level of DOX in cells treated with DOX-β-CD-PNVP compared to free DOX suggests high drug delivery efficiency of the compound.
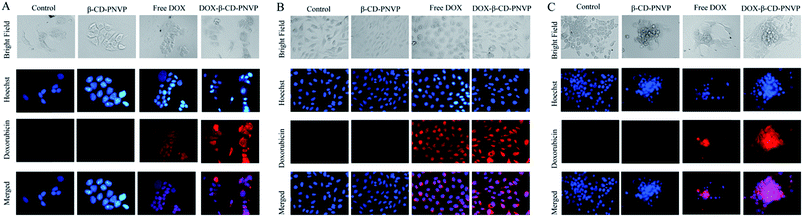 |
| Fig. 11 Uptake of free doxorubicin and DOX-loaded β-cyclodextrin-tagged poly(N-vinylpyrrolidone) (DOX-β-CD-PNVP). Uptake of free DOX or, DOX-β-CD-PNVP in MCF-7 (A), U2-OS (B), and HEPG2 (C) cells were studied by incubating in 24 well plates at a concentration of 50 000 cells per well for 4 h. Representative images shown were obtained using the fluorescence microscope EVOS® FL Cell Imaging System (Life Technologies, USA) equipped with Plan Fluor, 40X, NA 0.75 objective and with blue and red filters for Hoechst and Doxorubicin, respectively (n = 3). | |
Conclusions
In conclusion, tadpole-shaped β-cyclodextrin-tagged poly(N-vinylpyrrolidone) (DOX-β-CD-PNVP) has been synthesised via the click reaction of alkyne-terminated PNVP and azide-functionalized β-CD. Formed polymer is characterized by 1H NMR, FTIR, and TGA studies. The complexing ability of phenolphthalein with such polymer is lower than that of with β-CD. Loading of DOX in β-CD-PNVP is evidenced from DLS study. In vitro release study reveals the sustained DOX release behaviour up to 25 and 48% in PBS solutions of pHs 7.4 and 6.4, respectively, at 37 °C. DOX-β-CD-PNVP is found to be effective in intracellular transportation of doxorubicin, causing apoptosis, and retards the growth of the tumor cells of diverse origin. Our results suggest that DOX-β-CD-PNVP critically regulates the proliferative capacity of tumor cells where doxorubicin is specifically used like the end stage breast cancer and hepatocellular carcinomas. The results in HEPG2 cells are particularly encouraging since doxorubicin is one of the preferred drug to treat hepatocellular carcinomas. Overall, results presented in the current study demonstrate that DOX-loaded β-CD-PNVP can be used in targeted delivery of doxorubicin more effectively compared to doxorubicin alone.
Authors contributions
Performed the experiments: NKV, VKP, SKH, KR, PS, KM, SS; conceived and designed the experiments: BR, PPM, NM, NKV, VKP, SKH, KR; analyzed the data: NKV, VKP, SKH, KR, NM, BR, PPM; contributed reagents/materials/analysis tools: BR, PPM, NM, DC, PM; wrote the paper: BR, PPM, NKV, VKP, KR, SKH.
Acknowledgements
We gratefully acknowledge the financial supports from the Department of Biotechnology (DBT), New Delhi, India, through the grant nos BT/PR11490/BRB/10/675/2008 (PPM) and BT/PR889/NNT/28/570/2011 (BR and PPM). VKP, and NKV acknowledge CSIR, Government of India for the Research Fellowships.
References
- A. S. Wood, Kirk-Othmer Encyclopedia of Chemical Technology, ed. J. J. McKetta, D. F. Othmer and A. Standen, 2nd edn, Interscience, New York, 1970, p. 427 Search PubMed.
- F. Haaf, A. Sanner and F. Straub, Polymers of N-Vinylpyrrolidone: Synthesis, Characterization and Uses, Polym. J., 1985, 17, 143–152 CrossRef CAS.
- J. Chiefari, R. T. A. Mayadunne, G. Moad, E. Rizzardo and S. H. Thang, 1999, PCT IntAppl W099/31144.
- V. Coessens, T. Pintaver and K. Matyzaszewski, Functional polymers by atom transfer radical polymerization, Prog. Polym. Sci., 2001, 26, 337–377 CrossRef CAS.
- D. Wan, K. Satoh, M. Kamigaito and Y. Okamoto, Xanthate-mediated radical polymerization of N-vinylpyrrolidone in fluoroalcohols for simultaneous control of molecular weight and tacticity, Macromolecules, 2005, 38, 10397–10405 CrossRef CAS.
- R. Devasia, R. L. Bindu, N. Mougia and Y. Gnanou, Controlled radical polymerization of N-vinylpyrrolidone by reversible addition-fragmentation, Macromol. Symp., 2005, 229, 8–17 CrossRef CAS.
- P. Bilalis, M. Pitsikalis and N. Hadjichristidis, Controlled nitroxide-mediated and reversible addition-fragmentation chain transfer polymerization of N-vinylpyrrolidone: synthesis of block copolymers with styrene and 2-vinylpyridine, J. Polym. Sci., Part A: Polym. Chem., 2006, 44, 659–665 CrossRef CAS.
- S. Yamago, B. Ray, K. Iida, J. I. Yoshida, T. Tada, K. Yoshizawa, Y. Kwak, A. Goto and T. Fukuda, Highly versatile organostibine mediators for living radical polymerization, J. Am. Chem. Soc., 2004, 126, 13908–13909 CrossRef CAS PubMed.
- B. Ray, M. Kotani and S. Yamago, Highly controlled synthesis of poly(N-vinylpyrrolidone) and its block copolymers by organostibine-mediated living radical polymerization, Macromolecules, 2006, 39, 5259–5265 CrossRef CAS.
- S. I. Yusa, S. Yamago, M. Sugahara, S. Morikawa, T. Yamamoto and Y. Morishima, Thermoresponsivediblock copolymers of poly(N-isopropylacrylamide) and poly(N-vinyl-2-pyrroridone) synthesized via organotellurium-mediated controlled radical polymerization (TERP), Macromolecules, 2007, 40, 5907–5915 CrossRef CAS.
- S. Yamago, E. Kayahara, M. Kotani, B. Ray, Y. Kwak, A. Goto and T. Fukuda, Highly controlled living radical polymerization through dual activation of organobismuthines, Angew. Chem., Int. Ed., 2007, 46, 1304–1306 CrossRef CAS PubMed.
- X. Lu, S. Gong, L. Meng, C. Li, S. Yang and L. Zhang, Controllable synthesis of poly(N-vinylpyrrolidone) and its block copolymers by atom transfer radical polymerization, Polymer, 2007, 48, 2835–2842 CrossRef CAS PubMed.
- G. Pound, Z. Eksteen, R. Pfukwa, J. M. McKenzie, R. F. M. Lange and B. Klumperman, Unexpected reactions associated with the xanthate-mediated polymerization of N-vinylpyrrolidone, J. Polym. Sci., Part A: Polym. Chem., 2008, 46, 6575–6593 CrossRef CAS.
- G. Pound, F. Aguesse, J. B. McLeary, R. F. M. Lange and B. Klumperman, Xanthate-mediated copolymerization of vinyl monomers for amphiphilic and double-hydrophilic block copolymers with poly(ethylene glycol), Macromolecules, 2007, 40, 8861–8871 CrossRef CAS.
- V. K. Patel, A. K. Mishra, N. K. Vishwakarma, C. S. Biswas and B. Ray, (S)-2-(Ethyl propionate)-(O-ethyl xanthate) and (S)-2-(Ethyl isobutyrate)-(O-ethyl xanthate)-mediated RAFT polymerization of N-vinylpyrrolidone, Polym. Bull., 2010, 65, 97–101 CrossRef CAS PubMed.
- V. K. Patel, A. K. Mishra, N. K. Vishwakarma, C. S. Biswas, P. Maiti and B. Ray, Synthesis of Alkyne-Terminated Xanthate RAFT Agents and Their Uses for the Controlled Radical Polymerization of N-Vinylpyrrolidone and the Synthesis of Its Block Copolymer Using Click Chemistry, J. Appl. Polym. Sci., 2013, 127, 4305–4317 CrossRef CAS.
- K. Uekama, F. Hirayama and T. Irie, Cyclodextrin Drug Carrier Systems, Chem. Rev., 1998, 98, 2045–2076 CrossRef CAS PubMed.
- X. Ma and Y. Zhao, Biomedical Applications of Supramolecular Systems Based on Host-Guest Interactions, Chem. Rev., 2015 DOI:10.1021/cr500392w Special Issue, Supramolecular Chemistry.
- J. Xin, Z. Guo, X. Chen, W. Jing and J. Li, Studies of branched cationic β-cyclodextrin polymer/Iodomethacin complex and its release profile from alginate hydrogel, Int. J. Pharm., 2010, 386, 221–228 CrossRef CAS PubMed and references therein.
- Q. He, W. Wu, K. Xiu, Q. Zhang, F. Xu and J. Li, Controlled drug release system based on cyclodextrin-conjugated poly(lactic acid)-b-poly(ethylene glycol) micelles, Int. J. Pharm., 2013, 443, 110–119 CrossRef CAS PubMed and references therein.
- A. L. Nielsen, K. Steffensen and K. L. Larsen, Self-assembling microparticles with controllable disruption properties based on cyclodextrin interactions, Colloids Surf., B, 2009, 73, 267–275 CrossRef CAS PubMed.
- S. Ren, D. Chen and M. Jiang, Noncovalently Connected Micelles Based on a β-Cyclodextrin-Containing Polymer and Adamantane End-Capped Poly(ε-caprolactone) via Host–Guest Interactions, J. Polym. Sci., Part A: Polym. Chem., 2009, 47, 4267–4278 CrossRef CAS.
- T. Trellenkamp and H. Ritter, Poly(N-vinylpyrrolidone) Bearing Covalently Attached Cyclodextrin via Click-Chemistry: Synthesis, Characterization, and Complexation Behavior with Phenolphthalein, Macromolecules, 2010, 43, 5538–5543 CrossRef CAS.
- W. Zhang, B. Fang, A. Walther and A. H. E. Müller, Synthesis via RAFT Polymerization of tadpole-shaped organic/inorganic hybrid poly(acrylic acid) containing polyhedral oligomeric silsesquioxane (POSS) and their self-assembly in water, Macromolecules, 2009, 42, 2563–2569 CrossRef CAS.
- J. Y. Kim, W. I. Choi, Y. H. Kim, G. Tae, S. Y. Lee, K. Kim and I. C. Kwon, In-vivo tumor targeting of pluronic-based nano-carriers, J. Controlled Release, 2010, 147, 109–117 CrossRef CAS PubMed.
- X. P. Duan and Y. P. Li, Physicochemical characteristics of nanoparticles affect circulation, biodistribution, cellular internalization and trafficking, Small, 2013, 9, 1521–1532 CrossRef CAS PubMed.
- W. Wu, Q. Zhang, J. Wang, M. Chen, S. Li, Z. Lin and J. Li, Tumor-targeted aggregation of pH-sensitive nanocarriers for enhanced retention and rapid intracellular drug release, Polym. Chem., 2014, 5, 5668–5679 RSC.
- A. K. Mishra, V. K. Patel, N. K. Vishwakarma, C. S. Biswas, M. Raula, A. Mishra, T. K. Mandal and B. Ray, Synthesis of Well-Defined Amphiphilic Poly(ε-caprolactone)-b-poly-(N-vinylpyrrolidone) Block Copolymers via the Combination of ROP and Xanthate-Mediated RAFT Polymerization, Macromolecules, 2011, 44, 2465–2473 CrossRef CAS.
- T. P. Thomas, I. J. Majoros, A. Kotlyar, J. F. Kukowska-Latallo, A. Bielinska, A. Mycand and J. R. Baker, Targeting and Inhibition of Cell Growth by an Engineered Dendritic Nanodevice, J. Med. Chem., 2005, 48, 3729–3735 CrossRef CAS PubMed.
- P. P. Manna, S. K. Hira, A. A. Das, S. Bandyopadhyay and K. K. Gupta, IL-15 activated human peripheral blood dendritic cell kill allogeneic and xenogeneic endothelial cells via apoptosis, Cytokine, 2013, 61, 118–126 CrossRef CAS PubMed.
- S. K. Hira, A. K. Mishra, B. Ray and P. P. Manna, Targeted Delivery of Doxorubicin-Loaded Poly(ε-caprolactone)-b-Poly(N-vinylpyrrolidone) Micelles Enhances Antitumor Effect in Lymphoma, PLoS One, 2014, 9(4), e94309 Search PubMed.
- K. Ramesh, A. K. Mishra, V. K. Patel, N. K. Vishwakarma, C. S. Biswas, T. K. Paira, T. K. Mandal, P. Maiti, N. Misra and B. Ray, Synthesis of well-defined amphiphilic poly(D,L-lactide)-b-poly(N-vinylpyrrolidone) block copolymers using ROP and xanthate-mediated RAFT polymerization, Polymer, 2012, 53, 5743–5753 CrossRef CAS PubMed.
- F. Trotta, M. Zanetti and G. Camino, Thermal degradation of cyclodextrins, Polym. Degrad. Stab., 2000, 69, 373–379 CrossRef CAS.
Footnotes |
† Electronic supplementary information (ESI) available. See DOI: 10.1039/c4ra15359f |
‡ All three authors have equal contributions. |
|
This journal is © The Royal Society of Chemistry 2015 |