DOI:
10.1039/C5CY00058K
(Communication)
Catal. Sci. Technol., 2015,
5, 1447-1451
Room temperature, solventless telomerization of isoprene with alcohols using (N-heterocyclic carbene)–palladium catalysts†
Received
12th January 2015
, Accepted 3rd February 2015
First published on 5th February 2015
Abstract
The use of a custom-made palladium complex for the telomerisation of isoprene with alcohols under green conditions is described. Using a very low catalyst loading (0.1 mol%), this protocol allows for the reactions to be carried out without the need of high pressure equipment, at room temperature and under solventless conditions, affording high yields of telomerization products with high selectivity towards linear head-to-head and tail-to-head telomers.
Introduction
During the last fifty years, organic synthesis has reached a high level of sophistication with nearly no limits, allowing for the synthesis of highly complex molecules through protocols that provide specific chemo-, regio- and stereoselectivity. Nowadays, the main issue is the efficiency of a synthesis, which can be measured as the increase of complexity per transformation.1 At the same time, there are economic and environmental factors that demand high atom efficiency, low E-factor (mass ratio of waste to desired product) and a decrease of waste generated, in accordance to the principles of green chemistry.2 The development of synthetic protocols that can account for all these requirements is a challenge that in many cases can be only accomplished using catalysis, in any of its forms.3
Telomerizations are 2 bond-forming, 100% atom-efficient reactions that involve the metal-catalyzed oligomerization of 1,3-dienes (also known as taxogens) followed by the addition of a nucleophile (or telogen) to achieve substituted longer chains (Scheme 1).4 The telomerization reaction has been a key route in the preparation of interesting industrial products since it was discovered in 1967 by Smutny5 and Takahashi,6 independently. A vast range of products can be obtained due to the large variety of substrates and nucleophiles available. Butadiene and isoprene (2-methyl-1,3-butadiene) are by far the most popular dienes used due to the plethora of applications of the resulting products, although other taxogenes like myrcene have been used.7 The most common nucleophiles are water and alcohols, but other interesting products are obtained using other nucleophiles such as polyols,8 amines,9 carbon dioxide10 or acids.11,12 At a much smaller scale, telomerization reactions have also been applied in the synthesis of natural products.13
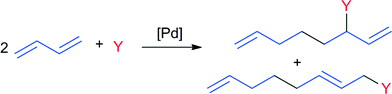 |
| Scheme 1 Telomerization of 1,3-butadiene with a nucleophile Y. | |
Isoprene is a common volatile diene naturally produced by many plants. Its skeleton is part of the terpenes family, which can be considered as chains consisting of multiple units of isoprene.14 The telomerization of isoprene is a more challenging reaction than the telomerization of butadiene, since isoprene is less reactive. In addition, not only isoprene is a non-symmetric molecule, meaning that the approach of the two units can be done in four different manners to give the head-to-head (H2H), head-to-tail (H2T), tail-to-head (T2H) and tail-to-tail (T2T) telomers, but also the attack of the nucleophile can occur in two different positions (Scheme 2). Therefore, eight different telomers are likely to form, as well as four dimers when no nucleophilic attack takes place, and trimers or oligomers if more than two isoprene units combine. Selectivity becomes an important goal to avoid separation of complex mixtures of products, whose compositions are strongly influenced by the catalyst employed and the reaction parameters, such as temperature and solvents.15
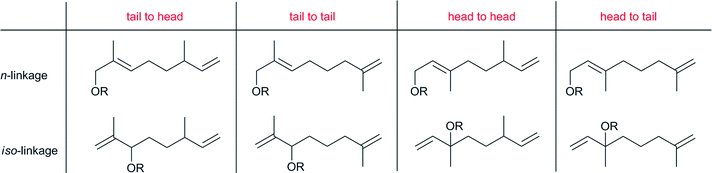 |
| Scheme 2 Telomers from the telomerization of isoprene with ROH. | |
Palladium catalysts have been by far the most widely employed in this reaction. Protocols in which a palladium complex and a phosphorous ligand are combined in situ are the most common in the literature.16 The substitution of phosphines by NHCs (N-heterocyclic carbenes)17 has shown, in general, improved activity and selectivities.15,18 More recently, the use of well-defined (NHC)-bearing Pd complexes in the telomerization of dienes with alcohols has allowed for a much easier handling, better control of metal to ligand stoichiometry and significant improvements in activity and chemo- and regioselectivity.19 This is especially pronounced for complexes bearing NHCs with mesityl groups as N-substituents.15,19–21 Our group recently developed a new series of (NHC)–palladium complexes with the general formula (NHC)PdCl2(TEA) (1a, 2a, Fig. 1),22 which have proven to be very active pre-catalysts for cross-coupling reactions such as Suzuki–Miyaura and Buchwald–Hartwig23 even under very mild reaction conditions. We decided to extend their application to telomerization reactions and therefore we synthesized two new complexes 1b and 2b, bearing mesityl N-substituents.
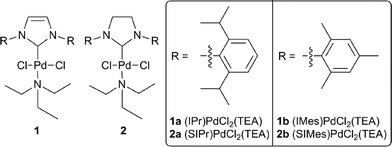 |
| Fig. 1 Pre-catalysts tested in this study. | |
Results and discussion
The new complexes 1b and 2b were prepared in excellent yields following the standard procedure for the synthesis of complexes 1a and 2a (Scheme 3). Both complexes were fully characterized by means of elemental analysis and 1H and 13C NMR spectroscopy. Crystals suitable for X-ray diffraction for each complex were obtained from dichloromethane/hexane solutions.24 The solid-state structures are depicted in Fig. 2 and 3 showing, as expected, square planar conformations for both complexes with the NHC trans to TEA. As it is customary for (NHC)–Pd complexes, the Pd–Ccarbenic distance is in the range of a single bond, with very similar values for 1b and 2b. Interestingly, both Pd–N1 distances are also very similar, implying a similar extent of electron donation from the NHC to the Pd centre.
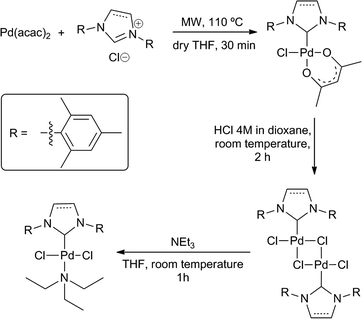 |
| Scheme 3 General procedure for the synthesis of (NHC)PdCl2(TEA) complexes. | |
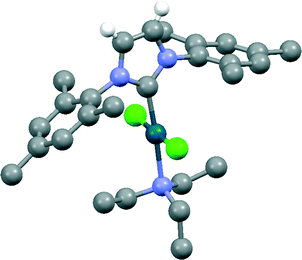 |
| Fig. 2 Crystal structure of (IMes)PdCl2(TEA) (1b). Hydrogen atoms are omitted for clarity except those on the backbone of the NHC. Selected distances (Å): Pd–Ccarbenic, 1.968(2); Pd–Cl1, 2.2931(6); Pd–Cl2, 2.3250(6); Pd–N1, 2.206(2). | |
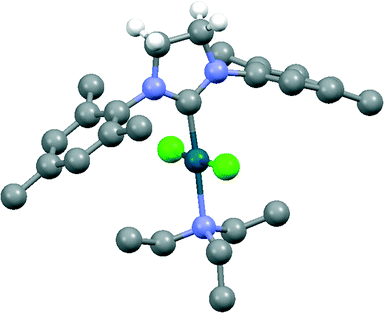 |
| Fig. 3 Crystal structure of (SIMes)PdCl2(TEA) (2b). Hydrogen atoms are omitted for clarity except those on the backbone of the NHC. Selected distances (Å): Pd–Ccarbenic, 1.974(4); Pd–Cl1, 2.3088(10); Pd–Cl2, 2.3013(10); Pd–N1, 2.198(3). | |
A review of the literature revealed that Pd-catalyzed telomerization reactions are performed under an inert atmosphere, usually in a pressure vessel or an autoclave, with temperatures ranging in most cases from 40 to 90 °C.16–21 Our previous experience with this type of complexes in catalytic cross-coupling reactions prompted us to attempt our telomerizations at 25 °C. Also, since different solvents, like THF or n-hexane, have proven to have some influence on the selectivity, we decided to carry out our reactions without solvent or excess of any of the coupling partners. In addition and to make our catalytic system as user-friendly as possible, we performed our reactions in regular glass pressure vials fitted with a screw-cap with a septum, using a low catalyst loading of 0.1 mol%.25 All the reagents except isoprene were loaded in open air. It is important to remark that all the reagents were used as received without further purification or drying. After closing it, the air in the vial was flushed out with N2, followed by the injection of the corresponding amount of isoprene through the septum. The reactions were carried out at room temperature and stopped after 72 h, when they were analyzed by gas-chromatrography/mass-spectrometry using pentadecane as internal standard. An initial comparison of the performance of complexes 1a–2b in the telomerization reaction of isoprene with MeOH was performed (Table 1). In all the cases, only n-linkage products were obtained. The conversion of isoprene to products was very high regardless of the complex utilized, but only those bearing unsaturated carbenes led to telomerization products (Table 1, entries 3, 4). (IMes)PdCl2(TEA) showed to be the most selective towards the formation of a given telomer, affording 83% of the T2H and H2H product (88% yield of all combined telomers). On the other hand, SIMes- and SIPr-bearing complexes resulted to be efficient catalysts for the synthesis of oligomers and polymers that are formed by condensation of at least three isoprene units, posing as potential catalysts for isoprene polymerization protocols. These results are consistent with previous findings in the literature, since bulkier carbenes such as SIPr have been reported to promote trimerization of isoprene producing sesquiterpenes.15
Table 1 Activity comparison in the solventless telomerization of isoprene with MeOH

|
Entry |
Complex |
Conversionb(%) |
H2Tc(%) |
T2Tc(%) |
T2Hc(%) |
H2Hc(%) |
Dimersc(%) |
Trimersc(%) |
Polymersc(%) |
a
Catalyst loading calculated as (mmol catalyst × 100)/(mmol alcohol). Conversion of isoprene to products. Percentage of component in the product mixture. |
1 |
2a
|
99 |
— |
— |
— |
— |
10 |
13 |
77 |
2 |
2b
|
89 |
— |
— |
— |
— |
1 |
1 |
98 |
3 |
1b
|
99 |
— |
5 |
7 |
76 |
4 |
6 |
2 |
4 |
1a
|
99 |
— |
15 |
2 |
29 |
32 |
8 |
15 |
The promising results accomplished with complex 1b encouraged us to test the scope of the reaction of isoprene with different alcohols. The same procedure used for the telomerisation of isoprene with methanol was applied for ethanol, 1- and 2-propanol and butanol (Table 2). The mixtures of telomers were isolated by distillation, analyzed by GC/MS, 1H and 13C NMR and identified by comparison with previously reported data (see ESI†). In all cases, with the exception of MeOH, this protocol allows for the selective formation of only two of the telomers, T2H and H2H. In general, the results showed that when the length of the alcohol chain increases, the conversion decreases, probably as a consequence of the increased steric hindrance of bulkier nucleophiles, as mechanistic studies suggest.15 The selectivity towards telomerization vs. dimerization and polymerization also decreased from methanol to butanol. Interestingly, while in most cases the H2H telomer is obtained as the major telomerization product, the selectivity switches towards the T2H when iPrOH is the nucleophile, which could also be a consequence of the bulk of the alcohol and the interactions with the corresponding dimers. To the best of our knowledge, these are the highest values of TON for telomerizations of isoprene with alcohols carried out at room temperature.
Table 2 Solventless telomerization of isoprene with different alcohols

|
Entry |
R |
Conversionc(%) |
TONc |
H2Td(%) |
T2Td(%) |
T2Hd(%) |
H2Hd(%) |
Dimersd(%) |
Oligomersd(%) |
a
Catalyst loading calculated as (mmol catalyst × 100)/(mmol alcohol). Conversion of isoprene to products. Turnover number for the formation of telomerisation products, based on mmol of alcohol. Percentage of component in product mixture. |
1 |
Me |
99 |
871 |
— |
5 |
7 |
76 |
4 |
8 |
2 |
Et |
91 |
637 |
— |
— |
27 |
43 |
24 |
6 |
3 |
Pr |
80 |
616 |
— |
|
30 |
47 |
18 |
5 |
4 |
iPr |
64 |
358 |
— |
— |
53 |
3 |
22 |
22 |
5 |
Bu |
75 |
443 |
— |
— |
14 |
45 |
20 |
21 |
The reaction of isoprene with methanol using complex 1b was also attempted without an inert atmosphere, under the same conditions. Although the conversion to products decreased to 33%, it is noteworthy that the selectivity and conversion rates for dimers, telomers and oligomers remained the same, being the linear H2H telomer the main product (75%).
Conclusions
In summary, we have synthesized two new (NHC)–Pd(II) complexes to apply them in isoprene telomerization reactions with alcohols under green conditions. In particular, the use of (IMes)PdCl2(TEA) (1b) as pre-catalyst allowed for the reactions to be carried out at room temperature, without the need of a solvent or excess of any of the reagents and at atmospheric pressure, achieving very high yields and remarkable selectivity towards linear T2H and H2H telomers. Ongoing work in our labs is focusing in the use of different nucleophiles and the application of this methodology to natural product synthesis.
Experimental
General considerations
All reagents were used as received. Imidazolium and imidazolidinium salts IMes·HCl, SIMes·HCl, IPr·HCl and SIPr·HCl were prepared following literature procedures.26,27 Complexes 1b and 2b were prepared following the standard procedure for the synthesis of 1a and 2a.22 Chemical shifts are reported in ppm using CDCl3 as solvent.
General procedure synthesis of (NHC)–Pd complexes
In a glovebox, a microwave vial equipped with a magnetic stir bar was charged with 0.5 mmol of Pd(acac)2, and 0.55 mmol of the corresponding NHC·HCl salt under nitrogen. Then 5 mL of dry THF were injected and the mixture was reacted for 0.5 h at 110 °C in a microwave reactor to obtain (IMes)PdCl(acac). After completion, THF was evaporated and the solid redisolved in CH2Cl2 and filtered through a silica plug. This solid was reacted with HCl in dioxane under nitrogen for 2 h, with vigorous stirring. A change in colour from yellow to orange was immediately detected after addition of HCl to obtain the dimer. 0.12 mmol of [Pd(μCl)Cl(IMes)]2, or 0.29 mmol of [Pd(μCl)Cl(SIMes)]2 were suspended in dichloromethane and an excess of triethylamine (0.5 mL) was added, stirring this mixture at room temperature for 1 hour. Finally, the solvent was removed under vacuum, resulting in a pale yellow solid that was washed several times with cold pentane.
(IMes)PdCl2(TEA) (1b).
The general procedure afforded 134 mg (96%) of the title compound. 1H NMR (CDCl3, 300 MHz): δ 0.92 (t, J = 7.2 Hz, N(CH2CH3)3, 9H), 2.34 (s, o-Ar–CH3, 12H), 2.36 (s, p-Ar–CH3, 6H), 2.55 (q, J = 7.2 Hz, N(CH2CH3)3, 6H), 6.99 (s, CH, 2H), 7.03 (s, CH, 2H), 7.03 (s, ArH, 4H). 13C{1H} NMR (CDCl3, 75 MHz): δ 9.2 (s, N(CH2CH3)3), 19.3 (s, CH3–ArH), 21.1 (s, CH3–ArH), 46.2 (s, N(CH2CH3)3), 124.0 (s, CH aromatic), 128.9 (s, CH aromatic), 135.1 (s, C aromatic), 136.4 (s, C aromatic), 138.9 (s, C aromatic), 151.8 (s, C carbene). Anal. calcd. For C27H39Cl2N3Pd: C, 55.63; H, 6.74; N, 7.21. Found: C, 55.49; H, 6.47; N, 7.06.
(SIMes)PdCl2(TEA) (2b).
The general procedure afforded 321 mg (94%) of the title compound. 1H NMR (CDCl3, 300 MHz): δ 0.82 (t, J = 7.2 Hz, N(CH2CH3)3, 9H), 2.31 (s, p-Ar–CH3, 6H), 2.49 (q, J = 7.0 Hz, N(CH2CH3), 6H), 2.55 (s, o-Ar–CH3, 12H), 4.00 (s, CH2, 4H), 6.99 (s, ArH, 4H). 13C{1H} NMR (CDCl3, 75 MHz): δ 9.0 (s, N(CH2CH3)3), 19.5 (s, CH3–Ph), 21.0 (s, CH3–Ph), 46.4 (s, N(CH2CH3)3), 50.9 (s, CH2), 129.2 (s, CH aromatic), 134.9 (s, C aromatic), 137.4 (s, C aromatic) and 138.2 (s, C aromatic), 183.7 (s, C carbene) Anal. calcd. For C27H41Cl2N3Pd: C, 55.44; H, 7.06; N, 7.18. Found: C, 55.09; H, 7.15; N, 7.13.
General procedure for the telomerization of isoprene with alcohols catalyzed by (NHC)PdCl2(TEA)
0.015 mmol (0.1 mol% catalyst loading) of the corresponding [(NHC)PdCl2(TEA)] catalyst and 0.2 mmol of sodium methoxide (0.015 mmol) were dissolved in 15 mmol of alcohol with vigorous stirring for 10 minutes in a 5 mL vial equipped with a screw cap with a septum. The closed vial was flushed with N2 and 3 mL of isoprene (30 mmol) were injected. The mixture was stirred at room temperature for 72 h to obtain a pale yellow, turbid solution. After filtration, gas chromatography/mass spectrometry analysis using pentadecane as standard confirmed the presence and quantities of dimers, telomers and trimers/oligomers. The mixture of telomers were separated from the first filtrate by vacuum distillation and analyzed by 1H and 13C NMR (see ESI†).
Acknowledgements
We thank the EPSRC UK National Crystallography Service at the University of Southampton for the collection of the crystallographic data for 1b. O.N. thanks the National Science Foundation (CHE-0924324) for funding initial stages of this work.
Notes and references
-
L. F. Tietze, G. Brasche and K. M. Gericke, Domino Reactions in Organic Synthesis, Wiley-VCH, Weinheim, Germany, 2006 Search PubMed.
-
P. T. Anastas and J. C. Warner, Green Chemistry: Theory and Practice, Oxford University Press, New York, U.S.A., 1998 Search PubMed.
-
R. A. Sheldon, I. Arends and U. Hanefeld, Green Chemistry and Catalysis, Wiley-VCH, Weinheim, Germany, 2008 Search PubMed.
-
(a) A. Behr, M. Becker, T. Beckmenn, L. Johnen, J. Leschinski and S. Reyer, Angew. Chem., Int. Ed., 2009, 48, 3598 CrossRef CAS PubMed;
(b)
J. Tsuji, Palladium Reagents and Catalysis, John Wiley & Sons, Chichester, U.K., 2004 Search PubMed.
- E. J. Smutny, J. Am. Chem. Soc., 1967, 89, 6793 CrossRef CAS.
- S. Takahashi, T. Shibano and N. Hagihara, Tetrahedron Lett., 1967, 8, 2451 CrossRef.
- A. Behr, L. Jonen and A. J. Vorholt, ChemCatChem, 2010, 2, 1271 CrossRef CAS.
- A. Gordillo, L. Durán Pachón and G. Rothenberg, Adv. Synth. Catal., 2009, 351, 325 CrossRef CAS.
-
(a) M. S. Viciu, F. Kauer, E. D. Stevens and S. P. Nolan, Organometallics, 2003, 22, 3175 CrossRef CAS;
(b) S. M. Maddock and M. G. Finn, Organometallics, 2000, 19, 2684 CrossRef CAS.
- A. Behr, P. Bahke, B. Klinger and M. Becker, J. Mol. Catal. A: Chem., 2007, 267, 149 CrossRef CAS.
- D. Rose and H. Lepper, J. Organomet. Chem., 1973, 49, 473 CrossRef CAS.
- C. U. Pittman, R. M. Hanes and J. J. Yang, J. Mol. Catal., 1982, 15, 377 CrossRef CAS.
-
(a) D. Holte, D. C. G. Götz and P. S. Baran, J. Org. Chem., 2012, 77, 825 CrossRef CAS PubMed;
(b) L. I. Zakharkin, V. V. Guseva and P. V. Petrovskii, Russ. Chem. Bull., 1995, 44, 1479 CrossRef.
-
E. Breitmaier, Terpenes: Flavors, Fragances, Pharmaca, Pheromones, Wiley-VCH, Weinheim, Germany, 2006 Search PubMed.
- R. C. Nunes, M. H. Araujo and E. N. dos Santos, Catal. Commun., 2007, 8, 1798 CrossRef CAS.
-
(a) F. Vollmüller, J. Krause, S. Klein, W. Mägerlein and M. Beller, Eur. J. Inorg. Chem., 2000, 8, 1825 CrossRef;
(b) P. W. Jolly, R. Mynott, B. Raspel, K.-P. Schick and G. Schroth, Organometallics, 1986, 5, 473 CrossRef CAS;
(c) P. Dani, J. Dupont and A. L. Monteiro, J. Braz. Chem. Soc., 1996, 7, 15 CrossRef CAS.
-
(a)
N-Heterocyclic Carbenes: Effective Tools for Organometallic Synthesis, ed. S. P. Nolan, Wiley-VCH, Weinheim, Germany, 2014 Search PubMed;
(b)
N-Heterocyclic Carbenes in Transition Metal Catalysis and Organocatalysis, ed. C. S. J. Cazin, Springer Science, Heidelberg, Germany, 2011 Search PubMed;
(c)
N-Heterocyclic Carbenes. From Laboratory Curiosities to Efficient Synthetic Tools, ed. S. Díez-González, RSC Publishing, London, U. K., 2010 Search PubMed.
- R. Jackstell, A. Grotevendt, D. Michalik, L. El Firdoussi and M. Beller, J. Organomet. Chem., 2007, 692, 4737 CrossRef CAS.
- R. Jackstell, S. Harkal, H. Jiao, A. Spannenberg, C. Borgmann, D. Röttger, F. Nierlich, M. Elliot, S. Niven, O. Navarro, M. S. Viciu, S. P. Nolan and M. Beller, Chem. – Eur. J., 2004, 10, 3891 CrossRef CAS PubMed.
- S. Harkal, R. Jackstell, F. Nierlich, D. Ortmann and M. Beller, Org. Lett., 2005, 7, 541 CrossRef CAS PubMed.
- L. Torrente-Murciano, A. Lapkin, D. J. Nielsen, I. Fallis and K. J. Cavell, Green Chem., 2010, 12, 866 RSC.
- M. T. Chen, D. A. Vicic, M. L. Turner and O. Navarro, Organometallics, 2011, 30, 5052 CrossRef CAS.
-
Metal-Catalyzed Cross-Coupling Reactions, ed. A. de Meijere and F. Diederich, Wiley-VCH, Weinheim, Germany, 2nd edn, 2004 Search PubMed.
- S. J. Coles and P. A. Gale, Chem. Sci., 2012, 3, 683 RSC.
- Catalyst loading referred to the mmol of nucleophile. In most cases in the literature the reactions are carried out using an excess of nucleophile and the catalyst loading is referred to the mmol of diene (see, for instance, ref. 20 and 21). Using that way of calculating the catalyst loading (for comparison), ours would be 0.05 mol%.
- L. Hinterman, Beilstein J. Org. Chem., 2007, 3, 22 CrossRef PubMed.
- G. A. Grasa, M. S. Viciu, J. Huang and S. P. Nolan, J. Org. Chem., 2001, 66, 7729 CrossRef CAS PubMed.
Footnote |
† Electronic supplementary information (ESI) available: 1H and 13C NMR spectra of 1b, 2b, characterization of telomers. CCDC 1039375 (1b) and 912794 (2b). For ESI and crystallographic data in CIF or other electronic format see DOI: 10.1039/c5cy00058k |
|
This journal is © The Royal Society of Chemistry 2015 |