DOI:
10.1039/C4OB01384K
(Communication)
Org. Biomol. Chem., 2014,
12, 7482-7485
Diastereoselective synthesis of functionalized pyrrolidines through N-bromosuccinimide-induced aziridine ring expansion cascade of cinnamylaziridine†
Received
2nd July 2014
, Accepted 6th August 2014
First published on 6th August 2014
Abstract
An efficient aziridine ring expansion cascade of cinnamylaziridine has been developed. N-Bromosuccinimide was used as the promoter. The resulting functionalized pyrrolidines are the fundamental units of many useful molecules.
Inefficient chemical synthesis does cause problems in pharmaceutical industry and obstructs the development of life-saving drugs.1 The negative impact of inefficient synthetic processes on the environment has also become an important concern in recent years.2 Electrophilic halogen-induced cascade reactions, which are considered as efficient and environmentally benign processes since multiple bonds can be formed (sometimes multiple stereocentres can also be obtained) in a single chemical operation,3,4 have been developed in recent years.5 For instance, transformations such as polyene cyclization (Scheme 1, eqn (1))6 and domino cyclization/cyclic ether ring expansion (Scheme 1, eqn (2)) have been documented.7 These reactions have proven to be highly valuable as a number of applications have been demonstrated. In contrast, the utilization of aziridine in such kind of cascade is less studied.8 Recently, we have reported a novel bromonium ion-initiated asymmetric aminocyclization–aziridine ring expansion cascade to afford substituted azepanes 2, which could be further transformed to other functional molecules (Scheme 1, eqn (3)).9 We reasoned that the same reaction protocol can be applied to the homolog cinnamylaziridine 3. Herein, we are pleased to report the diastereoselective synthesis of pyrrolidine 4,10 which contains three stereocenters through the electrophilic aminocyclization–ring expansion cascade using N-bromosuccinimide (NBS) as the halogen source (Scheme 1, eqn (4)).
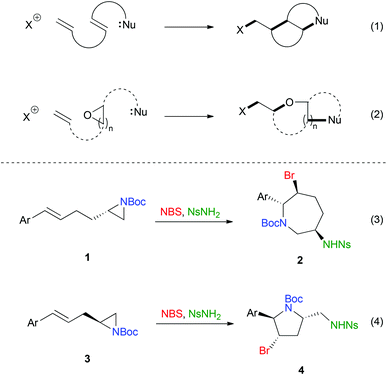 |
| Scheme 1 NBS-induced aminocyclization–aziridine ring expansion cascade. | |
It is noteworthy that functionalized pyrrolidines have been widely applied in various areas such as medicinal chemistry,11 organocatalysts,12 and chiral metal complexations.13 Some examples are shown in Fig. 1.
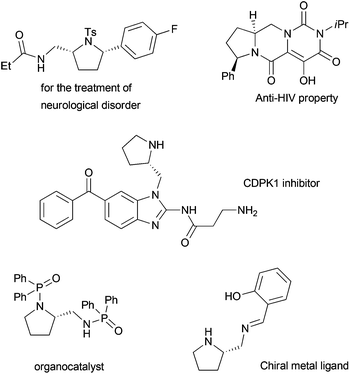 |
| Fig. 1 Examples of pyrrolidine-containing functional molecules. | |
3 was readily achievable by metathesis of L-aspartic acid-derived ethenylaziridine 6 and substituted styrene using Grubbs 2nd generation catalyst (Scheme 2).14
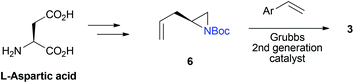 |
| Scheme 2 The synthesis of 3. | |
Initially, 3a was subjected to the investigation using p-nosyl amide as the nucleophilic partner. In this kind of cyclization cascade, two possible products, pyrrolidine and piperidine, can be obtained through path a and path b, respectively (vide infra, Scheme 3). Ethyl acetate, which was found to be a superior solvent medium for the cascade reaction of 1 in our previous study,9a gave poor selectivity of 4a
:
5a (1.1
:
1.0) despite the high overall reaction yield (Table 1, entry 1). The selectivity was slightly improved when reducing the reaction temperature to −20 °C (entry 3). After screening some common organic solvents, it was found that relatively non-polar solvents such as diethyl ether and toluene gave a sluggish reaction while polar solvents generally gave much better conversion (entries 4–12). Finally, the optimal solvent and temperature were found to be acetonitrile and −20 °C, respectively, which gave the selectivity of 4a
:
5a up to 3.2
:
1.0 (entry 7). Other halogenation sources, including N-chlorosuccinimide (NCS) and N-iodosuccinimide (NIS), were also examined under the optimal conditions and the reactions were found to be sluggish (entries 13 and 14). The structure of pyrrolidine 4a was determined by 2D-NMR analysis while piperidine 5a was confirmed by an X-ray crystallographic study on its tosylated derivative (CCDC 970435).15
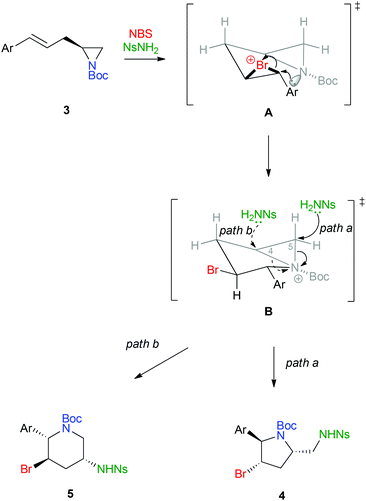 |
| Scheme 3 Proposed mechanism. | |
Table 1 Reaction optimization
Having identified the optimal conditions, we then explored the scope of the reaction and the results are listed in Table 2. In all cases, good yields of aziridine ring expansion products were obtained. No aromatic bromination was observed even for the electron-rich substituted systems (Table 2, entries 1–4). Compared with other substrates, ortho-CH3 phenyl system gave slightly lower reaction yield (72%), presumably due to the steric repulsion. Similar to our previous discovery,9a it appears that the electronic effect has no significant effect on the yield of the reaction. Generally, substrates with electron-rich substituents gave better regioselectivity, while substrates with relatively electron-poor substituents returned lower selectivity. The best selectivity (4e
:
5e = 4
:
1) was obtained with the tert-butyl phenyl substrate 3e (entry 4). It is noteworthy that only one diastereomer for both 4 and 5 was observed.
Table 2 NBS-induced aminocyclization–aziridine ring expansion cascade of 3
For the mechanism of this type of cascade, we believe that the cyclization might involve intermediate A through the bromination of 3 by the NBS/NsNH2 protocol (Scheme 3).9 Subsequently, the aziridine in A could react with the bromonium ion to give the aziridinium ion intermediate B.16 At this stage, NsNH2 could attack at either C-5 (path a) or C-4 (path b) position to give pyrrolidine 4 or piperidine 5, respectively. The preference on the formation of pyrrolidine 4 could be attributed to the substitution taken place at the less hindered C-5 position. The excellent diastereoselectivity in the formation of the cyclic amine products also suggests that the nucleophilic attacks in A and B occur in a SN2 manner.
Conclusions
In conclusion, we have developed an efficient bromonium ion-induced aziridine ring expansion cascade to afford functionalized pyrrolidines containing three stereocenters. These compounds are potential building blocks in various areas, and their possible synthetic applications are currently being studied.
Acknowledgements
We acknowledge the financial supports from the ASTAR-Public Sector Funding (grant no. 143-000-536-305), the NEA-ETRP (grant no. 143-000-547-490) and the GSK-EDB (grant no. 143-000-564-592). We acknowledge the receipt of a NUS Research Scholarship (to J.Z.).
Notes and references
- In a US Food and Drug Administration (FDA) report in 2004, it clearly stated that the problem of inefficient chemical synthesis has on the production of life-saving pharmaceuticals. For reference, see:
U.S. Food and Drug Administration, Innovation and Stagnation: Challenge and Opportunity on the Critical Path to New Medical Products, 2004 Search PubMed.
-
(a) D. J. C. Constable, P. J. Dunn, J. D. Hayler, G. R. Humphrey, J. L. Leazer, R. J. Linderman Jr., K. Lorenz, J. Manley, B. A. Pearlman, A. Wells, A. Zaks and T. Y. Zhang, Green Chem., 2007, 9, 411 RSC;
(b) D. J. C. Constable, A. D. Curzons, L. M. F. dos Santos, G. R. Geen, R. E. Hannah, J. D. Hayler, J. Kitteringham, M. A. McGuire, J. E. Richardson, P. Smith, R. L. Webb and M. Yu, Green Chem., 2001, 3, 7 RSC;
(c) A. D. Curzons, D. J. C. Constable, D. N. Mortimer and V. L. Cunningham, Green Chem., 2001, 3, 1 RSC;
(d) D. J. C. Constable, A. D. Curzons and V. L. Cunningham, Green Chem., 2002, 4, 521 RSC.
- For selected reviews, see:
(a) I. Ugi, A. Dömling and W. Hörl, Endeavour, 1994, 18, 115 CrossRef CAS;
(b) A. Dömling and I. Ugi, Angew. Chem., Int. Ed., 2000, 39, 3168 CrossRef;
(c)
J. Zhu and H. Bienaymé, Multicomponent Reactions, Wiley-VCH, Weinheim, 2005 Search PubMed;
(d) A. Dömling, Chem. Rev., 2006, 106, 17 CrossRef PubMed;
(e) B. B. Touré and D. G. Hall, Chem. Rev., 2009, 109, 4439 CrossRef PubMed;
(f) J. D. Sunderhaus and S. F. Martin, Chem. – Eur. J., 2009, 15, 1300 CrossRef CAS PubMed;
(g) B. Ganem, Acc. Chem. Res., 2009, 42, 463 CrossRef CAS PubMed;
(h) D. J. Ramón and M. Yus, Angew. Chem., Int. Ed., 2005, 44, 1602 CrossRef PubMed.
-
(a) Y. A. Serguchev, M. V. Ponomarenko, L. F. Lourie and A. N. Chernega, J. Fluorine Chem., 2003, 123, 207 CrossRef CAS;
(b) V. Nair, R. S. Menon, P. B. Beneesh, V. Sreekumar and S. Bindu, Org. Lett., 2004, 6, 767 CrossRef CAS PubMed;
(c) V. Nair, P. B. Beneesh, V. Sreekumar, S. Bindu, R. S. Menon and A. Deepthi, Tetrahedron Lett., 2005, 46, 201 CrossRef CAS PubMed;
(d) Y.-Y. Yeung, X. Gao and E. J. Corey, J. Am. Chem. Soc., 2006, 128, 9644 CrossRef CAS PubMed;
(e) T. L. Church, C. M. Byrne, E. B. Lobkovsky and G. W. Coates, J. Am. Chem. Soc., 2007, 129, 8156 CrossRef CAS PubMed;
(f) S. Hajra, S. Bar, D. Sinha and B. Maji, J. Org. Chem., 2008, 73, 4320 CrossRef CAS PubMed;
(g) T. Abe, H. Takeda, Y. Miwa, K. Yamada, R. Yanada and M. Ishikura, Helv. Chim. Acta, 2010, 93, 233 CrossRef CAS PubMed;
(h) D. C. Braddock, Org. Lett., 2006, 8, 6055 CrossRef CAS PubMed;
(i) D. C. Braddock, D. S. Millan, Y. Pérez-Fuertes, R. H. Pouwer, R. N. Sheppard, S. Solanki and A. J. P. White, J. Org. Chem., 2009, 74, 1835 CrossRef CAS PubMed;
(j) K. J. Bonney, D. C. Braddock, A. J. P. White and M. Yaqoob, J. Org. Chem., 2011, 76, 97 CrossRef CAS PubMed;
(k) K. J. Bonney and D. C. Braddock, J. Org. Chem., 2012, 77, 9574 CrossRef CAS PubMed;
(l) S. A. Snyder, D. S. Treitler, A. P. Brucks and W. Sattler, J. Am. Chem. Soc., 2011, 133, 15898 CrossRef CAS PubMed;
(m) S. A. Snyder, A. P. Brucks, D. S. Treitler and I. Moga, J. Am. Chem. Soc., 2012, 134, 17714 CrossRef CAS PubMed;
(n) J. Tanuwidjaja, S.-S. Ng and T. F. Jamison, J. Am. Chem. Soc., 2009, 131, 12084 CrossRef CAS PubMed;
(o) H. Fujioka, Y. Ohba, H. Hirose, K. Murai and Y. Kita, Angew. Chem., Int. Ed., 2005, 44, 734 CrossRef CAS PubMed;
(p) C. Recsei, B. Chan and C. S. P. McErlean, J. Org. Chem., 2014, 79, 880 CrossRef CAS PubMed.
-
(a) T. E. Müller and M. Beller, Chem. Rev., 1998, 98, 675 CrossRef PubMed;
(b) R. Severin and S. Doye, Chem. Soc. Rev., 2007, 36, 1407 RSC;
(c) O. Delacroix and A. C. Gaumont, Curr. Org. Chem., 2005, 9, 1851 CrossRef CAS.
-
(a) J. Barluenga, J. M. González, P. J. Campos and G. Asensio, Angew. Chem., Int., Ed. Engl., 1988, 27, 1546 CrossRef PubMed;
(b) J. Barluenga, M. Trincado, E. Rubio and J. M. González, J. Am. Chem. Soc., 2004, 126, 3416 CrossRef CAS PubMed;
(c) G. Stork and A. W. Burgstahler, J. Am. Chem. Soc., 1955, 77, 5068 CrossRef CAS;
(d) A. Eschenmoser and D. Arigoni, Helv. Chim. Acta, 2005, 88, 3011 CrossRef CAS PubMed;
(e) J. N. Carter-Franklin, J. D. Parrish, R. A. Tschirret-Guth, R. D. Little and A. Butler, J. Am. Chem. Soc., 2003, 125, 3688 CrossRef CAS PubMed;
(f) A. Sakakura, A. Ukai and K. Ishihara, Nature, 2007, 445, 900 CrossRef CAS PubMed;
(g) Y. Sawamura, H. Nakatsuji, A. Sakakura and K. Ishihara, Chem. Sci., 2013, 4, 4181 RSC.
-
(a) G. Haufe, J. Fluorine Chem., 1990, 46, 83 CrossRef CAS;
(b) H. Fujioka, H. Kitagawa, Y. Nagatomi and Y. Kita, J. Org. Chem., 1996, 61, 7309 CrossRef CAS PubMed;
(c) H. Fujioka, K. Nakahara, H. Hirose, K. Hirano, T. Oki and Y. Kita, J. Org. Chem., 2011, 47, 1060 CAS;
(d) N. Khan, H. Xiao, B. Zhang, X. Cheng and D. R. Mootoo, Tetrahedron, 1999, 55, 8303 CrossRef CAS;
(e) F. Bravo, F. E. McDonald, W. A. Neiwert and K. I. Hardcastle, Org. Lett., 2004, 6, 4487 CrossRef CAS PubMed;
(f) D. C. Braddock, D. S. Millan, Y. Pérez-Fuertes, R. H. Pouwer, R. N. Sheppard, S. Solanki and A. J. P. White, J. Org. Chem., 2009, 74, 1835 CrossRef CAS PubMed;
(g) S. A. Snyder and D. S. Treitler, Angew. Chem., Int. Ed., 2009, 48, 7899 CrossRef CAS PubMed;
(h) S. A. Snyder, D. S. Treitler and A. P. Brucks, J. Am. Chem. Soc., 2010, 132, 14303 CrossRef CAS PubMed;
(i) S. A. Snyder, D. S. Treitler and A. Schall, Tetrahedron, 2010, 66, 4796 CrossRef CAS PubMed;
(j) D. C. Braddock, S. A. Hermitage, L. Kwok, R. Pouwer, J. M. Redmond and A. J. P. White, Chem. Commun., 2009, 1082 RSC;
(k) D. C. Braddock, J. S. Marklew and A. J. F. Thomas, Chem. Commun., 2011, 47, 9051 RSC;
(l) D. C. Braddock, J. S. Marklew, K. M. Foote and A. J. P. White, Chirality, 2013, 25, 692 CrossRef CAS PubMed.
-
(a) M. D'hooghe, T. Vanlangendonck, K. W. Törnroos and N. De Kimpe, J. Org. Chem., 2006, 71, 4678 CrossRef PubMed;
(b) M. Sasaki and A. K. Yudin, J. Am. Chem. Soc., 2003, 125, 14242 CrossRef CAS PubMed.
-
(a) J. Zhou and Y.-Y. Yeung, Org. Lett., 2014, 16, 2134 CrossRef CAS PubMed. For other related efforts from our research group, see:
(b) J. Zhou and Y.-Y. Yeung, J. Org. Chem., 2014, 79, 4644 CrossRef CAS PubMed;
(c) D. W. Tay, I. T. Tsoi, J. C. Er, G. Y. C. Leung and Y.-Y. Yeung, Org. Lett., 2013, 15, 1310 CrossRef CAS PubMed;
(d) J. Zhou, L. Zhou and Y.-Y. Yeung, Org. Lett., 2012, 14, 5250 CrossRef CAS PubMed;
(e) J. Chen, S. Chng, L. Zhou and Y.-Y. Yeung, Org. Lett., 2011, 13, 6456 CrossRef CAS PubMed;
(f) L. Zhou, J. Chen, J. Zhou and Y.-Y. Yeung, Org. Lett., 2011, 13, 5804 CrossRef CAS PubMed;
(g) L. Zhou, J. Zhou, C. K. Tan, J. Chen and Y.-Y. Yeung, Org. Lett., 2011, 13, 2448 CrossRef CAS PubMed;
(h) L. Zhou, C. K. Tan, J. Zhou and Y.-Y. Yeung, J. Am. Chem. Soc., 2010, 132, 10245 CrossRef CAS PubMed.
-
(a) N. De Kimpe and M. Boelens, J. Chem. Soc., Chem. Commun., 1993, 916 RSC;
(b) J. Cossy, C. Dumas and D. G. Pardo, Eur. J. Org. Chem., 1999, 1693 CrossRef CAS;
(c) N. De Kimpe, M. Boelens, J. Piqueur and J. Baele, Tetrahedron Lett., 1994, 35, 1925 CrossRef CAS;
(d) H. A. Dondas and N. De Kimpe, Tetrahedron Lett., 2005, 46, 4179 CrossRef CAS PubMed;
(e) E. R. Alonso, K. A. Tehrani, M. Boelens and N. De Kimpe, Synlett, 2005, 1726 CAS.
-
(a) Z. Zhang, K. K. Ojo, S. M. Johnson, E. T. Larson, P. He, J. A. Geiger, A. Castellanos-Gonzalez, A. C. White Jr., M. Parsons, E. A. Merritt, D. J. Maly, C. L. M. J. Verlinde, W. C. Van Voorhis and E. Fan, Bioorg. Med. Chem. Lett., 2012, 22, 5264 CrossRef CAS PubMed;
(b)
V. Mutel and J. Wichmann, PCT Int. Appl, WO 2002002554, 2002 Search PubMed;
(c)
P. D. Williams, J. S. Wai, M. W. Embrey, D. D. Staas, L. Zhuang and H. M. Langford, PCT Int. Appl, WO 2005092099, 2005 Search PubMed.
-
(a) M. Bonsignore, M. Benaglia, F. Cozzi, A. Genoni, S. Rossi and L. Raimondi, Tetrahedron, 2012, 68, 8251 CrossRef CAS PubMed;
(b) S. Guizzetti and M. Benaglia, Eur. J. Org. Chem., 2010, 5529 CrossRef CAS PubMed;
(c) M. Bonsignore, M. Benaglia, R. Annunziata and G. Celentano, Synlett, 2011, 1085 CAS.
- Y. N. Belokon, V. I. Maleev, M. North, V. A. Larionov, T. F. Savel'yeva, A. Nijland and Y. V. Nelyubina, ACS Catal., 2013, 3, 1951 CrossRef CAS.
-
(a) M. Suhartono, A. E. Schneider, G. Durner and M. W. Gobel, Synthesis, 2010, 293 CAS;
(b) M. Venkataiah, G. Reddipalli, L. S. Jasti and N. W. Fadnavis, Tetrahedron: Asymmetry, 2011, 22, 1855 CrossRef CAS PubMed;
(c) P. Wessig and J. Schwarz, Synlett, 1997, 893 CrossRef CAS PubMed;
(d) A. K. Chatterjee, T.-L. Choi, D. P. Sanders and R. H. Grubbs, J. Am. Chem. Soc., 2003, 125, 11360 CrossRef CAS PubMed.
- The details appear in ESI.†.
- Different strategies for the synthesis of pyrrolidine from aziridine, see M. D'hooghe, W. Aelterman and N. De Kimpe, Org. Biomol. Chem., 2009, 7, 135 Search PubMed.
Footnote |
† Electronic supplementary information (ESI) available. CCDC 970435. For ESI and crystallographic data in CIF or other electronic format see DOI: 10.1039/c4ob01384k |
|
This journal is © The Royal Society of Chemistry 2014 |
Click here to see how this site uses Cookies. View our privacy policy here.