DOI:
10.1039/C4IB00025K
(Review Article)
Integr. Biol., 2014,
6, 486-510
Diagnostic morphology: biophysical indicators for iron-driven inflammatory diseases
Received
5th February 2014
, Accepted 16th March 2014
First published on 8th April 2014
Abstract
Most non-communicable diseases involve inflammatory changes in one or more vascular systems, and there is considerable evidence that unliganded iron plays major roles in this. Most studies concentrate on biochemical changes, but there are important biophysical correlates. Here we summarize recent microscopy-based observations to the effect that iron can have major effects on erythrocyte morphology, on erythrocyte deformability and on both fibrinogen polymerization and the consequent structure of the fibrin clots formed, each of which contributes significantly and negatively to such diseases. We highlight in particular type 2 diabetes mellitus, ischemic thrombotic stroke, systemic lupus erythematosus, hereditary hemochromatosis and Alzheimer's disease, while recognizing that many other diseases have co-morbidities (and similar causes). Inflammatory biomarkers such as ferritin and fibrinogen are themselves inflammatory, creating a positive feedback that exacerbates disease progression. The biophysical correlates we describe may provide novel, inexpensive and useful biomarkers of the therapeutic benefits of successful treatments.
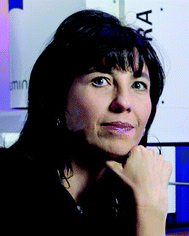
Etheresia Pretorius
| Resia Pretorius is a Research Professor in the Department of Physiology, Faculty of Health Sciences at the University of Pretoria, South Africa. Her interests lie in the ultrastructure and regulation of the human coagulation system, with particular focus on erythrocytes and fibrin networks, the role of iron metabolism and changes in the coagulation system due to inflammation. She is also Director of the Applied Morphology Research Centre of the University of Pretoria. She was chosen in 2011 as the winner of the African Union Kwame Nkrumah Scientific Awards Program: Women Scientist Regional Awards in the category Basic Science and Technology. |
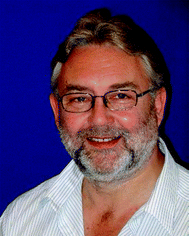
Douglas B. Kell
| Douglas Kell is a Research Professor in Bioanalytical Science at the University of Manchester, UK. His interests lie in systems biology, iron metabolism and dysregulation, cellular drug transporters, synthetic biology, e-science, chemometrics and cheminformatics. He was Director of the Manchester Centre for Integrative Systems Biology prior to a 5-year secondment (2008–2013) as Chief Executive of the UK Biotechnology and Biological Sciences Research Council. He is a Fellow of the Learned Society of Wales and of the American Association for the Advancement of Science, and was awarded a CBE for services to Science and Research in the New Year 2014 Honours list. |
Insight, innovation, integration
The Biological Insight of this manuscript is that morphological changes in both erythrocytes and fibrin clot structures are a significant accompaniment to a variety of diseases. The Technological Innovation is the use of advanced microscopy techniques (including atomic force microscopy) to measure these changes. The Benefit of Integration comes from the facts that (i) these readouts are at a much higher physiological or phenotypic level than the biochemical markers such as cytokines usually measured during inflammation, and (ii) by seeing their commonality across a range of inflammatory diseases we recognize this as genuinely Integrative Biology.
|
Introduction
Vascular and inflammatory diseases represent one of the major present medical burdens, and cardiovascular diseases (CVDs) are the number one cause of death globally.1–6 In 2008, the number of CVD deaths represented 30% of global deaths.1 CVDs are accompanied (and almost certainly partly caused) by various kinds of oxidative stress, and specific research studies have shown that oxidative stress is associated with the pathogenesis of diabetes, obesity, cancer, ageing, inflammation, neurodegenerative disorders, hypertension, apoptosis, cardiovascular diseases, heart failure, and so on (e.g.ref. 7–22). As their name implies, the various vascular diseases share a variety of properties, including raised levels of biochemical markers such as C-reactive protein,23–25 fibrinogen26 and leukocyte count,27 as well as many small molecules characteristic of exposure to ‘reactive oxygen species’.28,29 Also raised are various cytokines such as tumor necrosis factor alpha (TNFα) and interleukin-6 (IL-6) that might serve as early indicators of vascular dysfunction,30–32 and (most pertinently here) the iron storage protein ferritin.33–36 The enzyme heme oxygenase-1 (HO-1) catalyzes the degradation of heme, and is the major source of free iron released from heme metabolism.37–39 HO-1 may also be an important marker of inflammation, as upregulation of HO-1 occurs in a variety of situations that involve red blood cell lyses and release of heme; an example of this is in biomaterial–blood interaction during mechanical circulatory support.40 HO-1 activity is also increased systemically in inflammatory disorders like diabetes mellitus,41 rheumatoid arthritis42 and also pulmonary embolism.43 Markers like, e.g., HO-1 and cytokines, as well as iron-related inflammatory markers like ferritin, are therefore important from a biochemical standpoint. However, the scope of the current manuscript is to focus on the biophysical properties of RBCs and fibrin.
Although erythrocytes or red blood cells (RBCs) do not contain a nucleus or mitochondria,44,45 they are surprisingly complex and are probably the most studied cells in medical history.46 The dynamics of RBCs represent one of the most important aspects of the cardiovascular system47 and fundamental to this is RBC hemorheology, aggregation capabilities, deformability and viscosity.46,48–53 Bound up with this is the entire (intrinsic and extrinsic) clotting cascade,54,55 ending with the thrombin-catalysed formation of fibrin from fibrinogen.56 However, a cell-based model of coagulation is also now thought to be a more inclusive model to adopt when we study coagulation. This model incorporates the vital role of cells in coagulation processes,57 and is considered to correct deficiencies of the older largely cell-free cascade models.58–62Fig. 1 shows the traditional intrinsic and extrinsic pathways (waterfall pathway), as well as the cell-based pathway.
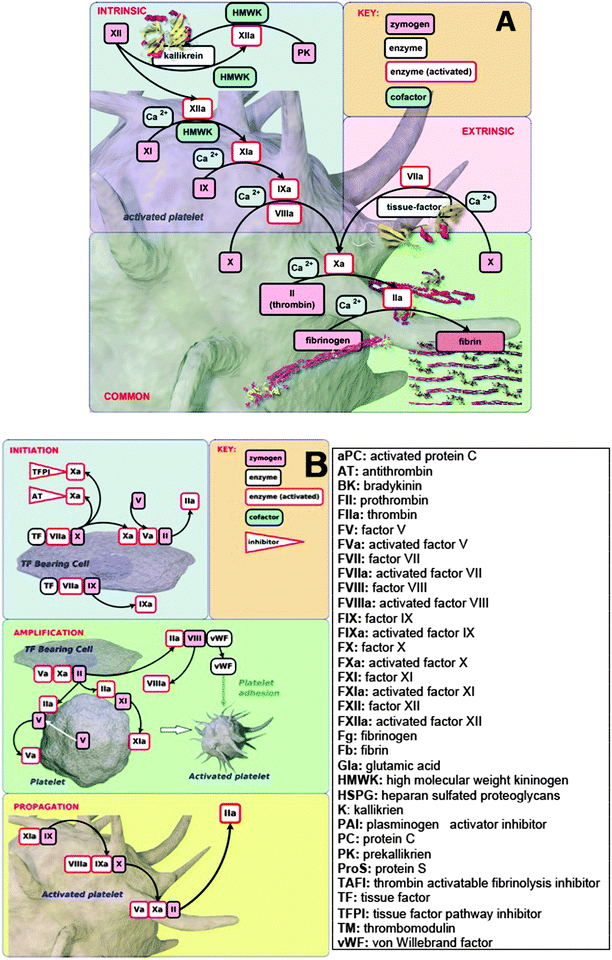 |
| Fig. 1 (A) Waterfall (traditional intrinsic and extrinsic pathways). (B) The cell-based coagulation pathway. The pictures are broadly based on ref. 58 and were rendered by Dr Steve O'Hagan. | |
RBCs are not only heme-iron-carrying sacks that facilitate the transport of respiratory gases, but they also play a variety of other roles:
• RBCs play a fundamental role in hemostasis and homeostasis, assuring our general well-being.63–65 Hemostasis and thrombus formation are integrally related to blood rheology and blood flow; furthermore,
• RBCs help in bringing platelets to the surface of an injured vessel wall, by random collisions between themselves and platelets, which allows platelets to move across flow streamlines in a form of “enhanced diffusion”.63
• RBCs have a role in binding inflammatory mediators to surface receptors.66,67
• RBCs play a fundamental role in the inflammatory process including having a changed deformability, rheology or sedimentation rate.53,65,67–80
Central to cardiovascular health is the optimal functioning of RBCs, and central to a variety of vascular and degenerative diseases is the presence of poorly liganded iron81–83 some of which comes from erythrocytes, and which leads to a variety of morphological and phenotypic manifestations. Therefore in this review, we will be taking an integrative approach that focuses on the use of biophysical properties of RBCs and fibrin(ogen) as diagnostic indicators of health. We shall be arguing that predictive, diagnostic morphology using RBCs may give us additional phenotypic information regarding the disease states and progression and that a “simple” finger prick might give us precise information regarding the health status of the individual. This manuscript is structured as shown in Fig. 2 (denoting the benefits84 of an overview figure of this type). Overall, we see the role of poorly liganded iron, largely released from ferritin, as having multiple effects on the morphology of both fibrin and erythrocytes, all of which contribute (negatively) to a variety of inflammatory vascular diseases (discussed later in the manuscript).
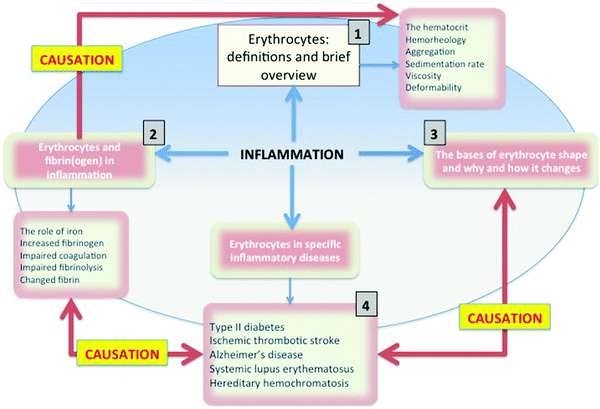 |
| Fig. 2 An overview figure summarizing the contents of this manuscript. | |
Definitions and a brief overview
The following terms will be used in this review:
The hematocrit: is also known as packed cell volume (PCV) or erythrocyte volume fraction (EVF). This is the volume of blood that contains the RBCs when packed by centrifugation, and is typically expressed as a fraction or % of blood. The hematocrit % may give us important information regarding the inflammatory state of an individual.79,85,86 A changed hematocrit (lower or higher values) is significantly correlated with higher cardiovascular risk87–89 and with inflammatory conditions like rheumatoid arthritis.89,90Table 1 shows some inflammatory diseases and associated hematocrit and hemoglobin levels.
Table 1 Some disease types and their hematocrit and hemoglobin counts (normal values of Hb: >13 g dl−1 for men; >12 g dl−1 for women; normal range for hematocrit: males: 40.7–50.3%; females: 36.1–44.3%)
Disease |
Hematocrit |
Coronary heart disease |
High hematocrit49 |
|
Diabetes |
High hematocrit91,92 |
Low hematocrit93 |
|
Hypertension |
High hematocrit94 |
|
Lupus erythematosus |
Low hematocrit95 |
Low Hb and low hematocrit96 |
|
Osteoarthritis |
Low hematocrit and low Hb97 |
|
Pre-diabetes |
High hematocrit98 |
|
Rheumatoid arthritis |
Low Hb99 |
Low Hb and hematocrit100 |
|
Stroke |
Low hematocrit101 |
|
Venous thrombosis in women (recurring) |
High hematocrit102 |
Hemorheology: is the study of blood flow or flow properties of blood in a vessel, with an emphasis on the behavior of RBCs as they interact with each other and as quantifiable biophysical patterns emerge from the interactions of the cells in the vascular system.48,49 These rheological properties are influenced by pathophysiological processes, thereby increasing the clinical relevance of blood rheology information.103 The oxygen flow depends,104 amongst other things, on the erythrocyte-to-blood volume ratio, which is represented by the hematocrit.105,106 The flow properties of blood represent a major field on its own,107–109 and for the scope of this paper, we will not discuss in much detail the rheological properties and patterns, although they follow from the various changes in cellular morphology and fibrin that we describe, and we recognize that they play a prominent role in all RBC properties discussed in the present paper.
Aggregation and sedimentation rate: aggregation110 can be defined as the reversible clumping of RBCs under low shear forces, while erythrocyte sedimentation rate (ESR) is based on the extent to which RBCs sediment, typically in 1 hour, and is expressed to the nearest mm in the first hour.111 Both aggregation and ESR are changed (typically increased) in disease, and are therefore known to be an indicator of the presence of disease (e.g.ref. 49 and 112–116). When inflammation is present, RBCs tend to stick to each other more readily and the RBCs form rows or stacks, where they fit into each other, due to their discoid shape; this is known as rouleau formation (Fig. 3). Both aggregation and ESR can influence rouleau formation,117 as can the presence of serum proteins.118 The most important plasma protein assisting in the creation of the rouleau is fibrinogen.119,120 A healthy plasma fibrinogen level is 2 to 4 mg mL−1,121–123 while an increased fibrinogen level will cause an increase in rouleau formation.50,51 Fibrinogen levels also influence the ESR124 and (by virtue of their sticking to RBCs and making them ‘heavier’) increases in fibrinogen will result in an increased sedimentation rate.
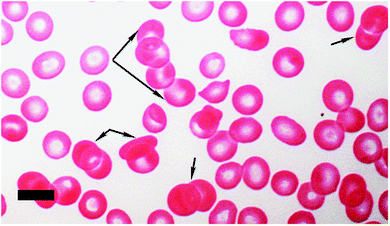 |
| Fig. 3 Rouleau formation (black arrows) in RBCs as seen with light microscopy (40× magnification); scale = 10 μm. | |
Viscosity: closely linked to aggregation125 is blood viscosity. RBC aggregation is considered to be the main determinant of blood viscosity (at low shear rate),49,51 but fibrinogen contributes significantly to plasma viscosity. The viscosity of a fluid represents the friction between a moving fluid and a stationary wall.48,126 The friction between the endothelial layer and the cellular components of blood will therefore be higher if there is an increased blood viscosity. It is well known that hemostatic–thrombotic mechanisms are influenced by hemodynamic factors127 and also that viscosity regulates how oxygen is transported to tissues and organs, and cardiovascular risks are also well-correlated with an increased viscosity.48,49,85,93,98,127–129
Deformability: RBC deformability is the ability to change shape, but to return to the original shape, which in the case of RBCs is a discoid shape. Deformability is an important determinant of blood viscosity.46 This deformability is typically needed when the cells squeeze through narrow blood vessels and the RBC shape change occurs because of the blood flow resistance in the vascular system.80 Therefore, deformability of RBCs, while they remain viable, is an important mechanical property of cells.46 A reduced deformability is known to be present in conditions such as stroke;130,131 it is linked directly to the RBC membrane properties including stiffness, rigidity and elasticity.73,129,132–136 One of the important properties used to look at membrane stiffness (not only restricted to RBCs) is the Young's modulus, which is a very particular measure of stiffness and elasticity.137,138 This can be measured using atomic force microscopy (AFM) technology, and is a measure of the stiffness of an elastic material,139 and can generally be defined as stress divided by the corresponding strain, with greater values indicating increased stiffness or decreased deformability.140 The method involves measuring the force as a probe is pushed (without membrane penetration) into the surface of and retracted from the sample in question. As each force curve's data can also be stored individually, it is possible to obtain quantitative measurements of the Young's modulus by fitting the slope of any force–distance curve of the image to an appropriate model.141–143 It is therefore a very informative method that reflects the deformability of RBCs.140
All of the above properties will reflect and be reflected in the dynamics of RBC function and their capability to deform naturally under shear forces. During inflammation, RBCs have a changed shape (losing their biconcave shape) (see below), as well as inadequate flow properties, and this is thought to have major influences on the development of cardiovascular disease.47 As we shall see, in addition to causing the biochemical changes alluded to above, inflammation also (and partly thereby) affects the mechanical properties of the RBC, the intrinsic cellular properties of the RBC membrane as well as the interactions of the RBCs with fibrin.72,144–147 The next paragraphs will discuss the role of RBCs and fibrinogen in inflammation.
Red blood cells and fibrin(ogen) in inflammation
Inflammation is both a biological response and a pathologic process consisting of a dynamic complex cascade of events; central to this response is fibrin(ogen) and the interactions of RBCs. We first look at the role of fibrin(ogen) in inflammation. Fibrinogen, a high molecular weight (340 kDa) clottable protein of human blood, plays a major role in hemostasis and thrombosis,148 by serving as a precursor of fibrin. Qualitative abnormalities of fibrinogen, also termed acquired dysfibrinogenemia, have been demonstrated in several disease states, mostly related to a prothrombotic tendency.149 Increased fibrinogen levels are well-known in the inflammatory response,86,87,150 and it is also well-known that increased fibrinogen is associated with cardiovascular events.151–155 Therefore, it follows that long before a thrombotic event, fibrinogen levels are increased in the inflammatory patient.152 Fibrin clot formation is part of the natural process of wound healing and tissue repair, and normally this is completed by removal of the clotted fibrin. Thus here we also have to look at fibrinolysis, as it is also known that fibrinolysis is impaired in inflammation and cardiovascular events.56,156–160 During a thrombotic event, the increased levels of fibrinogen, as well as other parameters (e.g. increased oxidative stress and iron levels – discussed later), cause abnormal fibrin fiber formation, visible as a denser coagulated mass or dense matted deposits (DMDs) (Fig. 4), and the resulting coagulum causes blood cells to change shape and to be trapped in the abnormal mesh.161 Therefore, the combination of a proneness to form a tighter fibrin network and impaired fibrinolysis is a feature of conditions like ischemic stroke,162 diabetes type II163,164 and numerous inflammatory diseases165–167 (see Table 2). This tighter clot is therefore due to a general “hypercoagulability” also known as a prothrombotic state, and an increased fibrinogen level is associated with hypercoagulability.168 Almost all inflammatory conditions are characterized by a hypercoagulable state, and this state may result in thrombotic events including thrombotic ischemic stroke.169 Hypercoagulability is also present in diabetes type II, rheumatoid arthritis, heart conditions and other inflammatory conditions.154,155,170–174 In other words, changed fibrinogen (whether it is increased fibrinogen levels or oxidative stress, etc.) is associated with hypercoagulability and results in a changed clotting profile.175 See Table 3 for diseases where increased fibrinogen and hypercoagulability are prevalent. There is evidence that fibrinogen synthesis in the liver is stimulated by the inflammatory cytokine IL-6, and that it can thus be lowered by an appropriate inhibitor.176,177 However, regardless of the concentration of fibrinogen, the nature of the clot that forms is particularly what matters, and Fig. 4 shows the terminal part of the typical cascade that results in the formation of either healthy or anomalous, diseased fibrin.
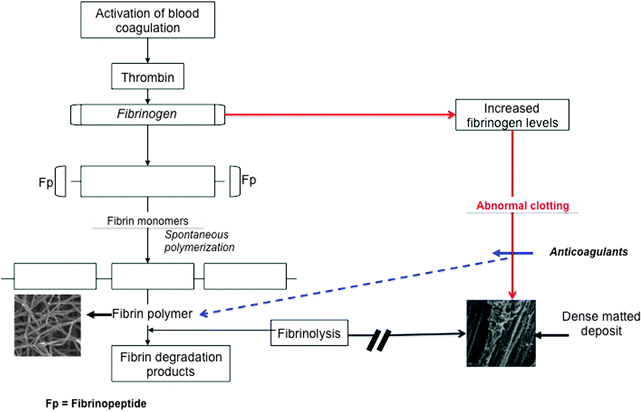 |
| Fig. 4 Activation of blood coagulation under normal conditions and where abnormal fibrinogen is present. During normal coagulation, thrombin acts on fibrinogen to form fibrin polymer fibers, and these fibers will dissociate easily under fibrinolytic conditions. When there is an increased fibrinogen level, abnormal clotting takes place that forms a dense matted deposit, resistant to typical fibrinolysis. However, in the presence of anticoagulants, the fibrin polymerized to again form a typical net, as seen in normal coagulation – anticoagulants, e.g. warfarin390 and aspirin,391 are known to decrease fibrinogen levels. Adjusted from Lipinski and Pretorius.167 | |
Table 2 Diseases where atypical fibrin fiber formation or an altered fibrin structure is prevalent – references particularly refer to a changed fibrin fiber structure as suggested by ultrastructure, scanning or transmission electron microscopy or laser-scanning confocal microscopy or clot lysis time
Disease |
Representative references |
Alzheimer's disease |
140, 178, 179
|
|
Antiphospholipid syndrome |
180
|
|
Arterial and venous thromboembolic diseases |
56
|
|
Chronic obstructive pulmonary disorder |
181
|
|
Coronary heart disease |
182, 183
|
|
Diabetes type II |
147, 184–188
|
|
Hereditary hemochromatosis |
145
|
|
Lupus erythematosus |
146, 189
|
|
Rheumatoid diseases |
190–192
|
|
Thromboembolic pulmonary hypertension-associated dysfibrinogenemias |
193
|
|
Thrombotic stroke and thrombotic disease |
72, 76, 144, 159–161, 194–198
|
Table 3 Selected diseases where increased fibrinogen and hypercoagulability are known to be present
Disease |
Representative references |
Antiphospholipid syndrome |
199–202
|
|
Alzheimer's disease |
172, 203
|
|
Atherosclerosis |
204–206
|
|
Cancer |
207–210
|
|
Chronic obstructive pulmonary disorder |
26, 86, 176, 177, 181, 211–230
|
|
Diabetes type II |
93, 98, 152, 163, 231–233
|
|
Heart disease and cardiovascular risk |
234–236
|
|
Lupus erythematosus |
189, 201, 237–240
|
|
Metabolic syndrome |
156, 232, 241–243
|
|
Rheumatoid diseases |
155, 244–246
|
|
Thrombotic stroke/thrombosis |
76, 148, 159, 160, 162, 194, 195, 247–250
|
We have previously shown visually how fibrin, generated during coagulation, is changed markedly in inflammatory conditions, e.g. stroke185,186,191,251 (Table 3 and Fig. 5A and B). Here, instead of a typical netted appearance seen in healthy individuals, the fibers form DMDs, with the individual fibers having a very much smaller diameter than that of normal fibrin fibers.250 Importantly, these DMDs may be the cause of an enhanced prevalence of thrombotic events.167 DMDs therefore reflect a hypercoagulability profile. Closely linked to this hypercoagulability of fibrin is the involvement of RBCs.147,180 When fibrin clots abnormally, RBCs are entrapped more tightly inside the clot72 (see Fig. 5B – RBC from a diabetic patient entrapped in fibrin). Previously, we have also found that fibrin fiber diameter is statistically significantly changed during thrombotic stroke where the fiber diameter becomes smaller and the fibers become netted.250 Typically there are thick and thin fibers in a healthy fibrin clot, with the thick fibers the prevailing fibers.250
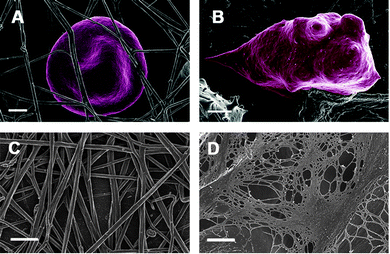 |
| Fig. 5 (A) RBC of a healthy individual where the cells keep a discoid shape when whole blood is coagulated with the addition of thrombin; (B) RBC of a diabetic patient where the RBC is entrapped in atypical fibrin fibers; (C) healthy fibrin network; (D) fibrin network in thrombotic stroke. Scale = 1 μm. | |
To understand how fibrin is packaged in health and disease, we need to look at the structures of both fibrinogen and the fibrin clot.56,181,197,198,252 Fibrinogen is a 340 kDa plasma glycoprotein consisting of two sets of three polypeptides (Aα, Bβ and γ) connected by 29 disulphide bonds253,254 that assemble to form more than 20 distinct independently folded domains.255 Thus, fibrinogen is a fibrous protein 45 nm in length with globular regions at each end and in the middle,256 and is composed of 2 Aα, 2 Bβ, and 2 γ-chains, arranged in a dimer of bilateral symmetry.257 It has a central E-region and D-regions, which are connected with the E-region by a coiled coil segment, which is composed of the β- and γ-chain C-termini, and the α-chain C-termini fold back on the coiled coil and interact with the E-region56 (see Fig. 6 – redrawn from ref. 56).
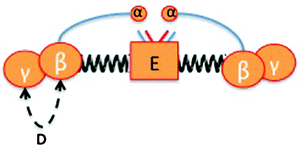 |
| Fig. 6 Schematic representation of fibrinogen, redrawn from ref. 56. Fibrinogen consists of 2 Aα, 2 Bβ, and 2 γ-chains, with a central E-region and D-regions, which are connected with the E-region by a coiled coil segment, which is composed of the β- and γ-chain C-termini, and the α-chain C-termini fold back on the coiled coil and interact with the E-region. Fibrinopeptides A (red) and B (blue) are released by the action of thrombin, and this initiates polymerization of the fibrin protofibrils. | |
When a fibrin net is formed in a healthy individual, thrombin catalyzes the hydrolytic removal of fibrinopeptides Aα and Bβ from fibrinogen, converting the molecule to fibrin, revealing binding sites at its central domain that interact with complementary sites at the end domains of other fibrin molecules.258 During the conversion of fibrinogen to fibrin, the αC domains dissociate from the central region, which allows them to interact intermolecularly, and this adds protofibrils to a fiber. The αC domain interactions appear to be for the enhancement of lateral aggregation during fibrin polymerization.256
These noncovalent interactions cause fibrin monomers to assemble in a half-staggered manner into two-stranded protofibrils.259 Upon growing to sufficient length, the protofibrils aggregate laterally to form fibers that branch into a three-dimensional network.260 After activation with thrombin, a pair of binding sites comprising Gly-Pro-Arg is exposed in the central nodule and combines with its complementary binding site α in the outer nodule of other molecules.260 The Aα group of Aα Gly-1 is juxtaposed between γ Asp-364 and γ Asp-330, and the guanidino group of Aα Arg-3 between the carboxyl group of γ Asp-364 and γ Gln-329 in the α site.260 Half molecule-staggered, double-stranded protofibrils are thus formed. Upon support of two adjacent D domains on the same strand, D–D self-association takes place involving Arg-275, Tyr-280, and Ser-300 of the γ-chain on the surface of the abutting two D domains. Thereafter, carboxy-terminal regions of the Aα-chains are released and interact with those of other protofibrils leading to the formation of thick fibrin bundles and networks,260 typically seen when a healthy fibrin fiber net forms259 (Fig. 5C) versus fibrin nets in thrombotic stroke (Fig. 5D). Yang and coworkers in 2000 mentioned that whether mature fibers are thick or thin depends on the relative contributions of different kinds of protofibril additions/interactions during fibrin assembly proteolyzed by thrombin.261
In the conversion from fibrinogen to fibrin there is therefore a series of large-scale conformational changes, and we have noted that the conversion of fibrinogen into the fibrin meshwork is changed significantly during stroke250 and other inflammatory conditions (e.g. diabetes185) where altered fibrin and fibrinogen are present (see Table 2). This changed packaging was also noted in thrombotic diseases, where there are abnormal plasma levels of γA/γ′ fibrinogen.253 Elevated levels have been associated with coronary artery disease262 and stroke.263 Also, the αC region is the origin of low modulus (1–10 MPa range), high extensibility, and strain stiffening in fibrin fibers.254 Nitration of fibrinogen (caused by the initial reaction of the hydroxyl radical with nitric oxide81,82) is a prothrombotic risk factor.264,265
Pathophysiological fibrin(ogen) not only causes abnormal clotting and hypercoagulability, but also affects RBC rheology; therefore, it is appropriate to return to RBCs, and look at what happens to the RBCs during inflammation, specifically under the influence of this altered fibrinogen profile. Various epidemiological studies have shown the associations between inflammation and blood rheology, viscosity, haematocrit, and RBC aggregation and inflammation.49,52,85,266–268 For a quick overview see Fig. 7, which shows the interactions between RBCs, inflammation, a prothrombotic state, and increased plasma fibrinogen levels, all causing pathorheology.
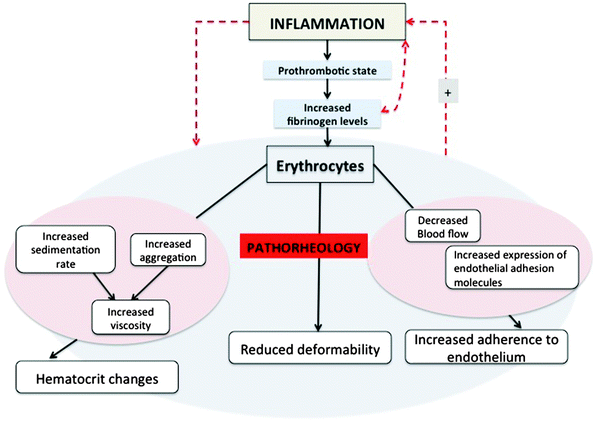 |
| Fig. 7 Pathorheology due to inflammation, and how feedback causes an “inflammatory loop”. Inflammation causes a prothrombotic state, which is associated with increased fibrinogen levels. Increased fibrinogen levels perpetuated inflammation. These processes cause pathorheology that involves major changes in RBCs (erythrocytes) – represented in the blue ellipse. | |
Under normal physiological conditions, RBCs are able to pass through narrow capillaries only as single cells rather than as aggregates.50 The protein fibrinogen is an important determinant of RBC aggregation, with an almost linear relationship between aggregate size and plasma fibrinogen concentration.85 Fibrinogen molecules adhere readily to the membrane surface of RBCs,269 encouraging erythrocyte aggregation.48,270,271 Therefore, it is not surprising that an increase in aggregation (under the control of increased fibrinogen levels in the plasma) is a well-known clinical manifestation in acute circulatory failure as well as acute coronary syndromes and stroke.75,85,103,131,272–275 Due to fibrinogen attachment to the RBC membrane, not only aggregation is influenced, but also RBC viscosity. This increased viscosity will ultimately cause the RBCs to struggle to pass through narrow capillaries.85 This increased viscosity is seen in several clinical states.49,51,98,127,128,133,276,277 It is thus appropriate to say that both RBC aggregation and fibrinogen interactions lead to a changed viscosity, and that this also plays an important role in optimal RBC functioning. It is therefore not surprising that increased blood viscosity and an increased fibrin concentration correlate with, and are strong predictors of, cardiovascular diseases and are important factors in the development of atherosclerosis.85,276,278
From the above paragraphs, it is clear that aggregation and viscosity are closely associated with (and indeed significantly due to) a changed fibrinogen profile, and that these parameters will ultimately influence flow dynamics.279 Flow dynamics or rheology is closely associated with RBC shape and deformability, and during inflammation, we see reduced RBC deformability.80
The question that now arises is what might be the cause of the changed fibrin(ogen) cross-linking, noted in the above paragraphs. One of the major culprits, albeit not the only one, is the presence of iron and its role in the production of hydroxyl radicals (HR).252,280–282 We have recently hypothesized that fibrinogen, as well as plasma proteins (in healthy individuals), can be converted into an insoluble, fibrin-like polymer by a non-enzymatic action of HR, following the addition of physiological levels of ferric iron.161 Electrostatic interactions of iron with fibrinogen and fibrin are also likely responsible, and would be expected to inhibit Arg-Glu binding, for instance. Similar phenomena are seen in diseases of ‘natural’ iron overload such as hereditary hemochromatosis.145,283–289 In inflammation, poorly liganded iron levels, and particularly serum ferritin levels, where the ferritin molecules have lost most of their iron en route from tissues, are increased in patients with diabetes type II, stroke, Alzheimer's disease, Parkinson's disease and many other inflammatory conditions.34,81,82,171,290–296
We have also recently shown that iron chelating and/or hydroxyl trapping agents are able to reverse the formation of this aberrant fibrin clot with its resultant excessive trapping of RBCs, even in the presence of iron overload.140,145 The possibility of the reversal of the changed fibrin clotting is consistent with the view that the aberrant fibrin morphology resulting in trapped RBCs in the presence of increased iron (particularly serum ferritin) is caused, at least in part, by unliganded (‘free’) iron, whether derived directly via raised ferritin levels or otherwise, and that lowering it or affecting the consequences of its action may be of therapeutic benefit.
We can summarize the information from the previous paragraphs as follows:
In inflammatory conditions we see
• increased iron levels and, particularly, increased serum ferritin levels
• enhanced RBC aggregation and blood viscosity
• hypercoagulability, implying a changed fibrin(ogen) profile
• trapping of RBCs in abnormally clotted fibrin.
The trapping of RBCs during a prothrombotic state, associated with increased iron levels in diabetes, is illustrated in Fig. 8.
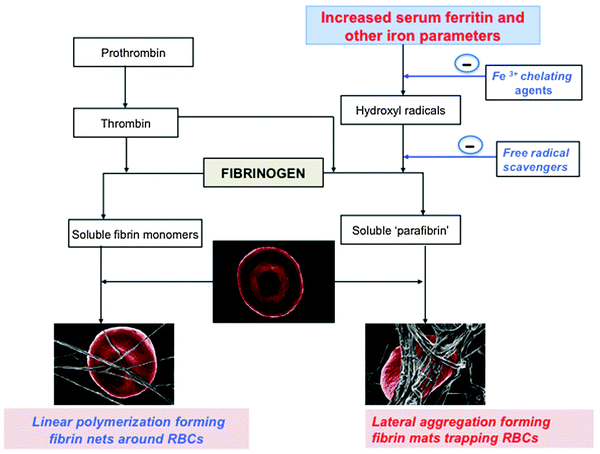 |
| Fig. 8 Trapping of RBCs in aberrant fibrin in diabetes due to increased serum ferritin and/or iron. Adjusted from Lipinski and Pretorius.392 | |
RBCs undergo a shape change during inflammation (which may be due to increased iron levels),147,297 associated with a change in the hematocrit, aggregation and viscosity of RBCs and an abnormal clotting profile. It is known that RBCs are characterized by a shape change in many disease states.46,73,266,298–300 Therefore, the next paragraphs will look more closely at RBC deformability properties and how and why they may change during the inflammatory process. Understanding the mechanics of RBC deformability under different pathophysiological conditions will lead to a better understanding of disease.
The bases of RBC shape and how and why it changes
Baskurt and Meiselman reviewed the role of RBC deformability in two comprehensive articles; they point out that RBCs are highly deformable, and that this physical property contributes significantly to aid blood flow, both under bulk flow conditions and in the microcirculation.85,301 They also argued that the ability of RBCs to undergo reversible aggregation is an important determinant of viscosity, because the size of RBC aggregates is inversely proportional to the magnitude of shear forces; the aggregates are dispersed with increasing shear forces, then reform under low-flow or static conditions.85 Recently, Dupire and co-workers showed that when RBCs ‘flip’ during flow, their orientation is determined by the shear rate. Under normal conditions, the cells have a “rolling” motion, and this motion permits the cells to avoid energetically costly deformations; it is a true signature of the cytoskeleton elasticity.299 The authors also showed that the biconcave cell shape is highly stable under moderate shear stresses. However, changes in the properties and associations of membrane skeletal proteins, the ratio of RBC membrane surface area to cell volume, cell morphology, and cytoplasmic viscosity all govern RBC deformability. It is therefore not surprising that RBC deformability is significantly altered by various pathophysiological conditions, and the alterations in RBC deformability in turn influence pathophysiology.302 Although the deformation of the RBC membrane is highly complex, it has three fundamental deformation modes – area expansion, shear, and bend of the membrane134 – and this extraordinary combination of membrane properties allows the RBC to undergo extensive deformation without cell fragmentation.136 We can therefore assume that when we have a changed RBC deformability, this will result in a changed erythrocyte physiology that can have a major adverse impact on blood flow. This will ultimately impact the homeostasis and hemostasis of the individual. However, to understand deformability, we need to look at the RBC membrane structure, and RBC's deformability may be a useful mechanical parameter for predicting the prognosis and for monitoring of patients.303
The membrane structure of RBCs consists of three layers:134
• an external carbohydrate-rich layer;
• the phospholipid bilayer of 4–5 nm thickness, embedded with transmembrane proteins;
• a 2-D triangular mesh-like spectrin–actin cytoskeleton network anchored to the phospholipid bilayer and ankyrin proteins. (Spectrin is a cytoskeletal protein that forms a hexagonal network that lines the intracellular side of the plasma membrane and interacts with short actin filaments that act as junctional complexes.)
The overlying asymmetric phospholipid bilayer membrane is supported by the underlying spectrin–actin cytoskeletal complex and it forms a simple hexagonal geometric matrix. Spectrins are flexible rods 0.2 microns in length with actin-binding sites at each end.304 Spectrins are assembled from α and β subunits, each comprised primarily of multiple copies of a 106-amino acid repeat. Spectrin α and β subunits are assembled antiparallel and side-to-side into heterodimers, which in turn are associated head-to-head to form tetramers.304 The principal proteins at the spectrin–actin junction are protein 4.1, adducin, tropomyosin, tropomodulin, and dematin. Spectrin is coupled to the inner surface of the RBC membrane primarily through association with ankyrin and the transmembrane proteins band 3 and 4.1.305,306 Band 3 is an abundant RBC integral membrane protein which has a membrane-spanning domain that catalyzes anion exchange and a cytoplasmic domain that binds proteins and thereby regulates the structure and function of the RBCs.80
Ankyrin proteins are adaptor proteins that mediate the attachment of the spectrin mesh to the integral membrane proteins, while band 3 is a transport protein responsible for mediating the exchange of chloride for bicarbonate across the membrane,307 and it is thus a carrier-mediated transport protein. Band 4.1 (also known as Beatty's protein) is an important structural element of the membrane skeleton and it regulates mechanical stability and deformability by stabilizing the spectrin–actin interactions by interacting with the spectrin and actin filaments to form the bulk of the membrane skeleton.308 The interaction of ankyrin and spectrin yields the major anchor between the membrane skeleton and the lipid bilayer and its optimal intact architecture is critical for RBC deformability and stability.309 Ankyrins therefore organize and stabilize protein networks in collaboration with other proteins including cytoskeletal proteins, cell adhesion molecules and large structural proteins.310 Cytoskeletal proteins such as β-spectrin contribute to the stability of the ankyrin protein network.310
Reduced deformability of RBCs is also an important feature in inflammation, also mediated by band 3, as well as by nitric oxide and ROS.80 ROS can lead to protein degradation in RBCs and in particular degradation of membrane proteins such as band 3 and spectrin,311 and in inflammation, a higher RBC band 3:α-spectrin ratio was associated with decreased RBC deformability. Importantly, RBC deformability was found to depend on the band 3 phosphorylation state.312 The plasma membrane together with its cytoskeletal support is therefore responsible for the maintenance of the shape and stability of the RBC and also for allowing extensive deformations when needed.184,305,313,314
Modifications of the lipid composition and the asymmetry of the bilayer have also been shown to affect the overall shape of the RBC and also the cell's deformability, impact on particularly the erythrocyte membrane integrity, when encountering shear stresses.315 Changes in the shape, mechanical characteristics or the integrity of RBCs have severe implications for the functionality of the cell, as can be seen in several dysfunctional states of the RBC, whether environmentally induced or due to hereditary defects or diseased states.184,313,316
Membrane roughness is another measure of interest. More specifically, it has been suggested that the cell-membrane skeleton integrity measured as surface roughness is well correlated with the functional status of the cell317 with a decrease of the membrane roughness seen in cells from diseased or ageing individuals.306,313,316,318–325
Roughness is simply measured by Rrms, which is defined as follows:
N is the total number of data points,
zi is the height of the
ith point and
zm is the mean height, as measured by atomic force microscopy (AFM).
306
What do we know about red blood cells in specific inflammatory diseases?
As mentioned in the previous paragraphs, fibrin and RBC deformability play important roles in hypercoagulability. One of the reasons for this may be due to unliganded iron, particularly that derived from serum ferritin. We have shown previously that in the presence of increased iron levels (particularly coupled to serum ferritin levels), RBCs lose their typical spherical shape. This was also seen in a number of inflammatory conditions like diabetes type II, stroke72,144,147 and Alzheimer's disease.140 The following sections discuss these changes in selected inflammatory conditions. This said, we are aware of a plethora of other diseases and cardiovascular risk factors that might influence RBCs, including type 1 diabetes, cigarette smoking, arterial hypertension, familial history of atherosclerosis, and even iron depletion (including iron deregulation in various types of anemia-related conditions). It is also well known that various drugs at appropriate concentrations can effect alterations in the structure of RBC membranes.300,326,327 However, in the next sections, we will be focusing specifically on RBCs in inflammatory conditions, without drug-mediated interactions, and in the presence of increased iron. The conditions that will be discussed in the following paragraphs are type 2 diabetes, ischemic thrombotic stroke, Alzheimer's disease, systemic lupus erythematosus and hereditary hemochromatosis.
Type 2 diabetes mellitus
Diabetes mellitus is one of the most debilitating conditions that our society suffers from, and is regarded as a cardiovascular risk factor, as well as a cardiovascular disease, due to its ability to progress to a stage of cardiovascular co-morbidity.328 Furthermore, diabetes is associated with oxidative stress and particularly the RBCs are vulnerable to this oxidative stress.328 We also know that diabetes is associated with elevated serum ferritin levels.171,293,329–332 We also know that increased iron levels cause oxidative stress.81,82,295,333,334 RBCs are one of the cell types that are particularly vulnerable to oxidative stress found in this condition,232 and oxidative stress is known to cause eryptosis.335 Eryptosis is a form of suicidal cell death, similar to apoptosis, and is characterized by cell shrinkage, cell membrane blebbing, and cell membrane phospholipid scrambling. RBCs remain in a hyperglycemic environment throughout their lifespan, and this affects their flow properties through alteration of deformation at the individual cell level, as well as aggregation at a collective level.336 Recently, we have shown that RBCs in diabetes have a changed shape as well as a decreased membrane roughness.147,167,184 Atomic force microscopy (AFM) was used to study membrane roughness, and scanning electron microscopy (SEM) was used to study RBC shape at high magnifications. RBCs in diabetes have a decreased membrane roughness, as well as an elongated shape. Measurements of surface roughness indicated alterations in the first order surface of the cell, relating to the cells' macro parameters. The roughness of the second order surface was also decreased, indicating alterations in the cytoskeletal matrix and the connections between band 3 and 4 proteins and the matrix. A decrease in roughness measurements in the third order surface indicates superficial protein structure rearrangement. The cytoskeletal proteins of RBCs from diabetic patients are heavily glycosylated and spectrin is oxidatively damaged;73 also several lipids (free cholesterol, sphingomyelin and phosphatidylcholine) on the outer surface of the phospholipid bilayer are significantly decreased.337,338 This directly correlates with the ultrastructural roughness results seen by the AFM of the second and third order respectively.184 Previous studies suggest that RBC rheology is altered in diabetes type II,85,266 and the results from the roughness and shape change support this.
Ischemic thrombotic stroke
Thrombotic stroke is one of the major cardiovascular diseases and it is also closely associated with oxidative stress10,334 as well as an increase in fibrinogen levels and therefore hypercoagulability.247 It is also known that iron plays an important role in the pathogenesis of cardiovascular diseases,291,339 including stroke.340–344 During ischemic stroke, erythrocytes undergo oxidative and proteolytic changes resulting in a changed cellular rheology and inflammatory processes. RBC changes have also been noted in thrombotic stroke and this includes sedimentation rate changes,112,115 changes of erythrocyte aggregation,274 a raised blood viscosity,345 and an impaired plasticity of the RBCs.69,131 We have previously reported that in thrombo-embolic ischemic stroke patients, 92% of erythrocytes present in smears are abnormal as viewed by a SEM.72,76,144 We also noted that in stroke, RBCs change shape and form close interactions with the abnormal fibrin fibers typically associated with the diseased clot.72 In blood smears of each of more than 60 stroke patients viewed by an SEM, RBCs have a changed form showing membrane pseudopodia-like extensions and some produce elongated membrane extensions that might be associated and form interactions with the fibrin fibers during pathological clot formation.
Alzheimer's disease
Alzheimer's disease (AD) is a devastating neurodegenerative dementing illness, also closely associated with oxidative stress.346–348 Although the disease is typically seen as primarily affecting the brain, there is increasing evidence that vascular components, including RBCs and fibrinogen, play a fundamental role in the progression of the condition.349–351 It is well-known that iron also plays a fundamental role in the progression of the disease.81,82,290,291,296,329,352–358
Increased RBC sedimentation and/or their aggregation have been observed in degenerative diseases such as atherosclerosis and inflammation, which are known to be associated with AD.115,359 It is thought that the abnormalities in RBCs and their flow contribute to AD by obstructing oxygen delivery to the brain.70,360,361 It is well-known that an oxygen deficit causes hypoxia and that this may lead to chronic inflammation.362,363 Mohanty and co-workers in 2010 noted that 15% of RBCs in AD patients were elongated, and that there were alterations in the RBC membrane architecture.364 There are also known interactions between RBCs and beta-amyloid and between fibrin and beta-amyloid365,366 in AD patients and that these interactions may accelerate neurovascular damage.367 We have recently shown that AD individuals with increased iron levels possess a changed RBC morphology. This was shown with light microscopy, SEM, AFM and confocal microscopy.140 We have also shown that this subgroup of AD patients have a decreased RBC membrane elasticity, measured using force–distance curves, and that the axial ratios and shape of the RBCs differed markedly from those of healthy individuals. A changed RBC membrane deformability may therefore play an important role in AD pathology.
Systemic lupus erythematosus
Multisystem autoimmune rheumatic diseases of which systemic lupus erythematosus (SLE) forms a part are heterogeneous rare disorders associated with substantial morbidity and mortality,368 and patients present a high prevalence of thrombotic and arteriosclerotic disease239 as well as chronic inflammation.369,370 SLE is also associated with an increase in serum ferritin levels.289,371–374 Despite decades of extensive work on the understanding of the etiopathogenesis of SLE, few biomarkers have been validated and widely accepted for this disease.375,376 Patients with SLE have enhanced RBC aggregation,377 microparticle formation,378 and a change in rheology.240,379,380 Very little is known about the deformability of RBCs in this condition; however, we have recently shown that lupoid erythrocytes are fused and clumped.146 This was suggested to be associated with distinct inflammatory ultrastructural changes found in the condition, which include platelet blebbing, generation of platelet-derived microparticles146,190 and the spontaneous formation of massive fibrin networks. It was concluded345 that the concerted actions of platelets and RBCs, as well as white blood cells caught in the inflammatory fibrin network, predispose to pro-thrombotic states in patients with SLE.
Hereditary hemochromatosis
Hereditary hemochromatosis (HH) is now probably the most well-known genetic iron overload disease.109,381–383 RBC membranes in HH display an aggregation and enlargement of intramembrane particles in comparison with structures seen in membranes from healthy donors.384 It is also known that the mean values of hemoglobin (Hb) in HH are raised.385,386 Recently we have shown that in genetically typed HH individuals with increased iron levels, as well as in individuals without the iron overload mutations but with hyperferritinemia, RBCs have a changed ultrastructure.145 In iron overload conditions, the sufferers are more prone to thrombotic diseases, mainly due to the presence of unliganded iron, and thereby the increased oxidative stress.387,388 Very striking differences were observed, in that the erythrocytes from HH and HF individuals were distorted and had a much greater axial ratio compared to that accompanying the discoid appearance seen in the normal samples.145
Concluding remarks
In inflammatory conditions, we see a number of changes in the biophysical properties of red blood cells, including in their hematocrit, aggregation, sedimentation rate and deformability. Closely associated with this is an increased or changed fibrin profile associated with hypercoagulability. The literature also suggests that inflammation is associated with increased serum ferritin levels. In diabetes, AD, HH as well as stroke and SLE, all of these are involved and interlinked with each other. However, what seems to be clear is that iron and in particular serum ferritin levels seem to underlie or correlate with all the rest of the parameters, in that when high serum ferritin levels are present in inflammatory conditions we find hypercoagulability and fibrin changes, as well as increased RBC aggregation and sedimentation. We have also shown that unliganded iron changes RBC shape. All of the above suggests strongly that morphological techniques may play a fundamental, and comparatively inexpensive, phenotypic role389 in providing a quick overview of the general health of an individual. However, very few studies focus on the use of predictive RBC morphology under inflammatory conditions. RBCs are extremely adaptive cells due to their capability to deform quickly under shear forces. Predictive RBC morphology may therefore give us important insights regarding the health status of an individual, and may be used together with traditional biomarkers in inflammatory conditions. The efficacy of treatment regimes on the integrity, shape, elasticity and roughness (and therefore the general health status) of RBCs may be tracked using predictive morphology, as the health status of RBCs is crucial to the overall wellness of individuals with inflammatory conditions.
Ethical clearance and consent
Ethical clearance was obtained from the Health Sciences Ethical Committee from the University of Pretoria and informed consent was obtained from all individuals who donated blood samples.
Conflict of interest statement
The authors have declared that no competing interests exist.
Funding
Funding bodies: We would like to acknowledge the National Research Foundation of South Africa (NRF): E. Pretorius; as well as the Biotechnology and Biological Sciences Research Council (BBSRC) for support (BB/L025752/1). The funders had no role in study design, data collection and analysis, decision to publish, or preparation of the manuscript.
Acknowledgements
We thank Dr Steve O'Hagan for rendering figures.
References
- WHO, Global status report on noncommunicable diseases, Geneva, 2011.
- J. P. Després, B. J. Arsenault, M. Côté, A. Cartier and I. Lemieux, Abdominal obesity: the cholesterol of the 21st century?, Can. J. Cardiol., 2008, 24(Suppl D), 7D–12D CrossRef.
- C. Ballantyne, B. Arroll and J. Shepherd, Lipids and CVD management: towards a global consensus, Eur. Heart J., 2005, 26, 2224–2231 CrossRef PubMed.
- A. Neylon, C. Canniffe, S. Anand, C. Kreatsoulas, G. J. Blake, D. Sugrue and C. McGorrian, A global perspective on psychosocial risk factors for cardiovascular disease, Prog. Cardiovasc. Dis., 2013, 55, 574–581 CrossRef PubMed.
- B. Norrving and B. Kissela, The global burden of stroke and need for a continuum of care, Neurology, 2013, 80, S5–S12 CrossRef PubMed.
- S. van de Vijver, S. Oti, J. Addo, A. de Graft-Aikins and C. Agyemang, Review of community-based interventions for prevention of cardiovascular diseases in low- and middle-income countries, Ethn. Health, 2012, 17, 651–676 CrossRef PubMed.
- D. Pitocco, M. Tesauro, R. Alessandro, G. Ghirlanda and C. Cardillo, Oxidative stress in diabetes: implications for vascular and other complications, Int. J. Mol. Sci., 2013, 14, 21525–21550 CrossRef CAS PubMed.
- G. H. Kim, J. J. Ryan and S. L. Archer, The role of redox signaling in epigenetics and cardiovascular disease, Antioxid. Redox Signaling, 2013, 18, 1920–1936 CrossRef CAS PubMed.
- A. Bachi, I. Dalle-Donne and A. Scaloni, Redox proteomics: chemical principles, methodological approaches and biological/biomedical promises, Chem. Rev., 2013, 113, 596–698 CrossRef CAS PubMed.
- F. Bonomini, S. Tengattini, A. Fabiano, R. Bianchi and R. Rezzani, Atherosclerosis and oxidative stress, Histol. Histopathol., 2008, 23, 381–390 CAS.
- D. Giustarini, I. Dalle-Donne, D. Tsikas and R. Rossi, Oxidative stress and human diseases: origin, link, measurement, mechanisms, and biomarkers, Crit. Rev. Clin. Lab. Sci., 2009, 46, 241–281 CrossRef CAS PubMed.
- K. Jomova, S. Baros and M. Valko, Redox active metal-induced oxidative stress in biological systems., Transition Met. Chem., 2012, 37, 127–134 CrossRef CAS PubMed.
- P. I. Moreira, A. I. Duarte, M. S. Santos, A. C. Rego and C. R. Oliveira, An integrative view of the role of oxidative stress, mitochondria and insulin in Alzheimer's disease, J. Alzheimer's Dis., 2009, 16, 741–761 Search PubMed.
- A. Nunomura, R. J. Castellani, X. Zhu, P. I. Moreira, G. Perry and M. A. Smith, Involvement of oxidative stress in Alzheimer disease, J. Neuropathol. Exp. Neurol., 2006, 65, 631–641 CrossRef CAS PubMed.
- S. Orrenius, V. Gogvadze and B. Zhivotovsky, Mitochondrial oxidative stress: implications for cell death, Annu. Rev. Pharmacol. Toxicol., 2007, 47, 143–183 CrossRef CAS PubMed.
- A. Skoumalová and J. Hort, Blood markers of oxidative stress in Alzheimer's disease, J. Cell. Mol. Med., 2012, 16, 2291–2300 CrossRef PubMed.
- N. A. Strobel, R. G. Fassett, S. A. Marsh and J. S. Coombes, Oxidative stress biomarkers as predictors of cardiovascular disease, Int. J. Cardiol., 2011, 147, 191–201 CrossRef PubMed.
- R. M. Touyz, Reactive oxygen species, vascular oxidative stress, and redox signaling in hypertension: what is the clinical significance?, Hypertension, 2004, 44, 248–252 CrossRef CAS PubMed.
- G. Vitale, S. Salvioli and C. Franceschi, Oxidative stress and the ageing endocrine system, Nat. Rev. Endocrinol., 2013, 9, 228–240 CrossRef CAS PubMed.
- C. Webb and D. Twedt, Oxidative stress and liver disease, Vet. Clin. North Am. Small Anim. Pract., 2008, 38, 125–135 CrossRef PubMed.
- Y. H. Wei and H. C. Lee, Oxidative stress, mitochondrial DNA mutation, and impairment of antioxidant enzymes in aging, Exp. Biol. Med., 2002, 227, 671–682 CAS.
- X. Zhu, B. Su, X. Wang, M. A. Smith and G. Perry, Causes of oxidative stress in Alzheimer disease, Cell. Mol. Life Sci., 2007, 64, 2202–2210 CrossRef CAS PubMed.
- H. Koyama and Y. Nishizawa, AGEs/RAGE in CKD: irreversible metabolic memory road toward CVD?, Eur. J. Clin. Invest., 2010, 40, 623–635 CrossRef CAS PubMed.
- M. S. Karbiner, L. Sierra, C. Minahk, M. C. Fonio, M. P. Bruno and S. Jerez, The role of oxidative stress in alterations of hematological parameters and inflammatory markers induced by early hypercholesterolemia, Life Sci., 2013, 93, 503–508 CrossRef CAS PubMed.
- J. Zacho, A. Tybjaerg-Hansen, J. S. Jensen, P. Grande, H. Sillesen and B. G. Nordestgaard, Genetically elevated C-reactive protein and ischemic vascular disease, N. Engl. J. Med., 2008, 359, 1897–1908 CrossRef CAS PubMed.
- M. Thomsen, T. S. Ingebrigtsen, J. L. Marott, M. Dahl, P. Lange, J. Vestbo and B. G. Nordestgaard, Inflammatory Biomarkers and Exacerbations in Chronic Obstructive Pulmonary Disease, JAMA, J. Am. Med. Assoc., 2013, 309, 2353–2361 CrossRef CAS PubMed.
- K. Mochizuki, R. Miyauchi, Y. Misaki, N. Kasezawa, K. Tohyama and T. Goda, Associations between leukocyte counts and cardiovascular disease risk factors in apparently healthy Japanese men, J. Nutr. Sci. Vitaminol., 2012, 58, 181–186 CrossRef CAS.
- B. Uttara, A. V. Singh, P. Zamboni and R. T. Mahajan, Oxidative stress and neurodegenerative diseases: a review of upstream and downstream antioxidant therapeutic options, Curr. Neuropharmacol., 2009, 7, 65–74 CrossRef CAS PubMed.
-
B. Halliwell and J. M. C. Gutteridge, Free Radicals Biol. Med., Oxford University Press, Oxford, 4th edn, 2006 Search PubMed.
- M. Cortez-Cooper, E. Meaders, J. Stallings, S. Haddow, B. Kraj, G. Sloan, K. K. McCully and J. G. Cannon, Soluble TNF and IL-6 receptors: indicators of vascular health in women without cardiovascular disease, Vasc. Med., 2013, 18, 282–289 CrossRef PubMed.
- I. Jialal, B. A. Huet, H. Kaur, A. Chien and S. Devaraj, Increased toll-like receptor activity in patients with metabolic syndrome, Diabetes Care, 2012, 35, 900–904 CrossRef CAS PubMed.
- B. T. Baune, M. Rothermundt, K. H. Ladwig, C. Meisinger and K. Berger, Systemic inflammation (Interleukin 6) predicts all-cause mortality in men: results from a 9-year follow-up of the MEMO Study, Age, 2011, 33, 209–217 CrossRef CAS PubMed.
- P. Syrovatka, P. Kraml, J. Potockova, L. Fialova, M. Vejrazka, J. Crkovska and M. Andel, Relationship between increased body iron stores, oxidative stress and insulin resistance in healthy men, Ann. Nutr. Metab., 2009, 54, 268–274 CrossRef CAS PubMed.
- W. Wang, M. A. Knovich, L. G. Coffman, F. M. Torti and S. V. Torti, Serum ferritin: Past, present and future, Biochim. Biophys. Acta, 2010, 1800, 760–769 CrossRef CAS PubMed.
- J. G. Wilson, J. H. Lindquist, S. C. Grambow, E. D. Crook and J. F. Maher, Potential role of increased iron stores in diabetes, Am. J. Med. Sci., 2003, 325, 332–339 CrossRef PubMed.
- M. Zegrean, Association of body iron stores with development of cardiovascular disease in the adult population: a systematic review of the literature, Can. J. Cardiovasc. Nurs., 2009, 19, 26–32 Search PubMed.
- A. Wilks and G. Heinzl, Heme oxygenation and the widening paradigm of heme degradation, Arch. Biochem. Biophys., 2014, 544, 87–95 CrossRef CAS PubMed.
- Z. A. Khan, Y. P. Barbin, M. Cukiernik, P. C. Adams and S. Chakrabarti, Heme-oxygenase-mediated iron
accumulation in the liver, Can. J. Physiol. Pharmacol., 2004, 82, 448–456 CrossRef CAS PubMed.
- M. E. Conrad and J. N. Umbreit, Iron absorption and transport-an update, Am. J. Hematol., 2000, 64, 287–298 CrossRef CAS.
- V. G. Nielsen, E. C. Pearson and M. C. Smith, Increased carbon monoxide production by hemeoxygenase-1 caused by device-mediated hemolysis: thrombotic phantom menace?, Artif. Organs, 2013, 37, 1008–1014 CrossRef CAS PubMed.
- W. Bao, F. Song, X. Li, S. Rong, W. Yang, M. Zhang, P. Yao, L. Hao, N. Yang, F. B. Hu and L. Liu, Plasma heme oxygenase-1 concentration is elevated in individuals with type 2 diabetes mellitus, PLoS One, 2010, 5, e12371 Search PubMed.
- A. Takahashi, M. Mori, T. Naruto, S. Nakajima, T. Miyamae, T. Imagawa and S. Yokota, The role of heme oxygenase-1 in systemic-onset juvenile idiopathic arthritis, Mod. Rheumatol., 2009, 19, 302–308 CrossRef CAS PubMed.
- D. T. Redford, J. L. Thompson, J. C. McCulloch and V. G. Nielsen, Left atrial myxoma presenting as pulmonary embolism: potential role of heme oxygenase-1, Blood Coagulation Fibrinolysis, 2014 Search PubMed , epub ahead of print.
- E. Daugas, C. Cande and G. Kroemer, Erythrocytes: death of a mummy, Cell Death Differ., 2001, 8, 1131–1133 CrossRef CAS PubMed.
- A. Lupescu, R. Bissinger, K. Jilani and F. Lang, Triggering of suicidal erythrocyte death by celecoxib, Toxins, 2013, 5, 1543–1554 CrossRef CAS PubMed.
- G. Minetti, S. Egee, D. Morsdorf, P. Steffen, A. Makhro, C. Achilli, A. Ciana, J. Wang, G. Bouyer, I. Bernhardt, C. Wagner, S. Thomas, A. Bogdanova and L. Kaestner, Red cell investigations: art and artefacts, Blood Rev., 2013, 27, 91–101 CrossRef CAS PubMed.
- T. D. AlMomani, S. C. Vigmostad, V. K. Chivukula, L. Al-zube, O. Smadi and S. BaniHani, Red blood cell flow in the cardiovascular system: a fluid dynamics perspective, Crit. Rev. Biomed. Eng., 2012, 40, 427–440 CrossRef.
- Y. I. Cho and D. J. Cho, Hemorheology and microvascular disorders, Korean Circ. J., 2011, 41, 287–295 CrossRef PubMed.
- J. Danesh, R. Collins, R. Peto and G. D. Lowe, Haematocrit, viscosity, erythrocyte sedimentation rate: meta-analyses of prospective studies of coronary heart disease, Eur. Heart J., 2000, 21, 515–520 CrossRef CAS PubMed.
- J. Tripette, T. Alexy, M. D. Hardy-Dessources, D. Mougenel, E. Beltan, T. Chalabi, R. Chout, M. Etienne-Julan, O. Hue, H. J. Meiselman and P. Connes, Red blood cell aggregation, aggregate strength and oxygen transport potential of blood are abnormal in both homozygous sickle cell anemia and sickle-hemoglobin C disease, Haematologica, 2009, 94, 1060–1065 CrossRef CAS PubMed.
- M. W. Rampling, H. J. Meiselman, B. Neu and O. K. Baskurt, Influence of cell-specific factors on red blood cell aggregation, Biorheology, 2004, 41, 91–112 CAS.
- G. Reggiori, G. Occhipinti, A. De Gasperi, J. L. Vincent and M. Piagnerelli, Early alterations of red blood cell rheology in critically ill patients, Crit. Care Med., 2009, 37, 3041–3046 CrossRef PubMed.
- S. K. Ballas and N. Mohandas, Sickle red cell microrheology and sickle blood rheology, Microcirculation, 2004, 11, 209–225 CrossRef CAS.
- B. C. Lechtenberg, S. M. Freund and J. A. Huntington, An ensemble view of thrombin allostery, Biol. Chem., 2012, 393, 889–898 CrossRef CAS PubMed.
- A. S. Wolberg and A. E. Mast, Tissue factor and
factor VIIa–hemostasis and beyond, Thromb. Res., 2012, 129(suppl 2), S1–S4 CrossRef CAS PubMed.
- A. Undas and R. A. S. Ariëns, Fibrin clot structure and function: a role in the pathophysiology of arterial and venous thromboembolic diseases, Arterioscler., Thromb., Vasc. Biol., 2011, 31, e88–e99 CrossRef CAS PubMed.
- R. A. Campbell, K. A. Overmyer, C. H. Selzman, B. C. Sheridan and A. S. Wolberg, Contributions of extravascular and intravascular cells to fibrin network formation, structure, and stability, Blood, 2009, 114, 4886–4896 CrossRef CAS PubMed.
- S. A. Smith, The cell-based model of coagulation, J. Vet. Emerg. Crit. Care, 2009, 19, 3–10 CrossRef PubMed.
- A. K. Vine, Recent advances in haemostasis and thrombosis, Retina, 2009, 29, 1–7 CrossRef PubMed.
- V. G. Nielsen, Beyond cell based models of coagulation: analyses of coagulation with clot “lifespan” resistance-time relationships, Thromb. Res., 2008, 122, 145–152 CrossRef CAS PubMed.
- G. Romney and M. Glick, An updated concept of coagulation with clinical implications, J. Am. Dent. Assoc., 2009, 140, 567–574 CrossRef CAS.
- H. R. Roberts, M. Hoffman and D. M. Monroe, A cell-based model of thrombin generation, Semin. Thromb. Hemostasis, 2006, 32(suppl 1), 32–38 CrossRef CAS PubMed.
- E. F. Grabowski, K. Yam and M. Gerace, Evaluation of hemostasis in flowing blood, Am. J. Hematol., 2012, 87(suppl 1), S51–S55 CrossRef PubMed.
- P. G. Gallagher, Disorders of red cell volume regulation, Curr. Opin. Hematol., 2013, 20, 201–207 CrossRef CAS PubMed.
- J. P. Lopes de Almeida, S. Oliveira and C. Saldanha, Erythrocyte as a biological sensor, Clin. Hemorheol. Microcirc., 2012, 51, 1–20 CAS.
- M. J. Telen, Red blood cell surface adhesion molecules: their possible roles in normal human physiology and disease, Semin. Hematol., 2000, 37, 130–142 CrossRef CAS.
- P. S. Walmet, J. R. Eckman and T. M. Wick, Inflammatory mediators promote strong sickle cell adherence to endothelium under venular flow conditions, Am. J. Hematol., 2003, 73, 215–224 CrossRef CAS PubMed.
- M. Montagnana, G. Cervellin, T. Meschi and G. Lippi, The role of red blood cell distribution width in cardiovascular and thrombotic disorders, Clin. Chem. Lab. Med., 2012, 50, 635–641 CAS.
- P. Kowal, Hemorheology in cerebral ischemia, Neurol. Neurochir. Pol., 1996, 30(suppl 2), 7–11 Search PubMed.
- J. G. Mohanty, D. M. Eckley, J. D. Williamson, L. J. Launer and J. M. Rifkind, Do red blood cell-beta-amyloid interactions alter oxygen delivery in Alzheimer's disease?, Adv. Exp. Med. Biol., 2008, 614, 29–35 CrossRef CAS.
- E. Nagy, J. W. Eaton, V. Jeney, M. P. Soares, Z. Varga, Z. Galajda, J. Szentmiklosi, G. Mehes, T. Csonka, A. Smith, G. M. Vercellotti, G. Balla and J. Balla, Red cells, hemoglobin, heme, iron, and atherogenesis, Arterioscler. Thromb. Vasc. Biol., 2010, 30, 1347–1353 CrossRef CAS PubMed.
- E. Pretorius and B. Lipinski, Thromboembolic ischemic stroke changes red blood cell morphology, Cardiovasc. Pathol., 2013, 22, 241–242 CrossRef PubMed.
- S. Shin, Y. Ku, N. Babu and M. Singh, Erythrocyte deformability and its variation in diabetes mellitus, Indian J. Exp. Biol., 2007, 45, 121–128 CAS.
- M. N. Starodubtseva, T. G. Kuznetsova, N. I. Yegorenkov and S. N. Cherenkevich, Structural and mechanical characteristics of erythrocyte membranes in patients with type 2 diabetes mellitus, Bull. Exp. Biol. Med., 2008, 145, 99–103 CrossRef CAS PubMed.
- A. Steinvil, S. Berliner, I. Shapira, O. Rogowski, D. Justo, J. George, A. Halkin, G. Keren, A. Finkelstein, S. Banai and Y. Arbel, Time to rheology
in acute myocardial infarction: inflammation and erythrocyte aggregation as a consequence and not necessarily as precursors of the disease, Clin. Res. Cardiol., 2010, 99, 651–656 CrossRef PubMed.
- A. C. Swanepoel and E. Pretorius, Scanning electron microscopy analysis of erythrocytes in thromboembolic ischemic stroke, Int. J. Lab. Hematol., 2012, 34, 185–191 CrossRef CAS PubMed.
- G. Erikssen, K. Liestol, J. V. Bjornholt, H. Stormorken, E. Thaulow and J. Erikssen, Erythrocyte sedimentation rate: a possible marker of atherosclerosis and a strong predictor of coronary heart disease mortality, Eur. Heart J., 2000, 21, 1614–1620 CrossRef CAS PubMed.
- S. Gangopadhyay, V. K. Vijayan and S. K. Bansal, Lipids of erythrocyte membranes of COPD patients: a quantitative and qualitative study, COPD, 2012, 9, 322–331 Search PubMed.
- M. Madjid and O. Fatemi, Components of the complete blood count as risk predictors for coronary heart disease: in-depth review and update, Tex. Heart Inst. J., 2013, 40, 17–29 Search PubMed.
- M. Straat, R. van Bruggen, D. de Korte and N. P. Juffermans, Red blood cell clearance in inflammation, Transfus. Med. Hemother., 2012, 39, 353–361 CrossRef PubMed.
- D. B. Kell, Iron behaving badly: inappropriate iron chelation as a major contributor to the aetiology of vascular and other progressive inflammatory and degenerative diseases, BMC Med. Genomics, 2009, 2, 2 CrossRef PubMed.
- D. B. Kell, Towards a unifying, systems biology understanding of large-scale cellular death and destruction caused by poorly liganded iron: Parkinson's, Huntington's, Alzheimer's, prions, bactericides, chemical toxicology and others as examples, Arch. Toxicol., 2010, 84, 825–889 CrossRef CAS PubMed.
- C. Funke, S. A. Schneider, D. Berg and D. B. Kell, Genetics and iron in the systems biology of Parkinson's disease and some related disorders, Neurochem. Int., 2013, 62, 637–652 CrossRef CAS PubMed.
- B. Wong, Points of view: the overview figure, Nat. Methods, 2011, 8, 365 CrossRef CAS PubMed.
- O. K. Baskurt and H. J. Meiselman, Erythrocyte aggregation: basic aspects and clinical importance, Clin. Hemorheol. Microcirc., 2013, 53, 23–37 Search PubMed.
- D. G. Yanbaeva, M. A. Dentener, E. C. Creutzberg, G. Wesseling and E. F. Wouters, Systemic effects of smoking, Chest, 2007, 131, 1557–1566 CrossRef CAS PubMed.
- C. G. Spencer, D. C. Felmeden, A. D. Blann and G. Y. Lip, Haemorheological, platelet and endothelial indices in relation to global measures of cardiovascular risk in hypertensive patients: a substudy of the Anglo-Scandinavian Cardiac Outcomes Trial, J. Intern. Med., 2007, 261, 82–90 CrossRef CAS PubMed.
- D. R. Gagnon, T. J. Zhang, F. N. Brand and W. B. Kannel, Hematocrit and the risk of cardiovascular disease–the Framingham study: a 34-year follow-up, Am. Heart J., 1994, 127, 674–682 CrossRef CAS.
- P. T. Skretteberg, J. Bodegård, S. E. Kjeldsen, G. Erikssen, E. Thaulow, L. Sandvik and J. E. Erikssen, Interaction between inflammation and blood viscosity predicts cardiovascular mortality, Scand. Cardiovasc. J., 2010, 44, 107–112 CrossRef CAS.
- B. S. Bull, W. C. Levy, J. C. Westengard, M. Farr, P. F. Smith, J. F. Apperley, P. A. Bacon and J. Stuart, Ranking of laboratory tests by consensus analysis, Lancet, 1986, 2, 377–380 CrossRef CAS.
- L. J. Tamariz, J. H. Young, J. S. Pankow, H. C. Yeh, M. I. Schmidt, B. Astor and F. L. Brancati, Blood viscosity and hematocrit as risk factors for type 2 diabetes mellitus: the atherosclerosis risk in communities (ARIC) study, Am. J. Epidemiol., 2008, 168, 1153–1160 CrossRef PubMed.
- S. G. Wannamethee, I. J. Perry and A. G. Shaper, Hematocrit and risk of NIDDM, Diabetes, 1996, 45, 576–579 CrossRef CAS.
- C. Irace, F. Scarinci, V. Scorcia, D. Bruzzichessi, R. Fiorentino, G. Randazzo, G. Scorcia and A. Gnasso, Association among low whole blood viscosity, haematocrit, haemoglobin and diabetic
retinopathy in subjects with type 2 diabetes, Br. J. Ophthalmol., 2011, 95, 94–98 CrossRef CAS PubMed.
- L. Paul, P. Jeemon, J. Hewitt, L. McCallum, P. Higgins, M. Walters, J. McClure, J. Dawson, P. Meredith, G. C. Jones, S. Muir, A. F. Dominiczak, G. Lowe, G. T. McInnes and S. Padmanabhan, Hematocrit predicts long-term mortality in a nonlinear and sex-specific manner in hypertensive adults, Hypertension, 2012, 60, 631–638 CrossRef CAS PubMed.
- G. Moroni, S. Quaglini, B. Gallelli, G. Banfi, P. Messa and C. Ponticelli, Progressive improvement of patient and renal survival and reduction of morbidity over time in patients with lupus nephritis (LN) followed for 20 years, Lupus, 2013, 22, 810–818 CrossRef CAS PubMed.
- B. Satirapoj, J. Wongchinsri, N. Youngprang, B. Laonapaporn, T. Chitrada, S. Lapkittichareonchai and J. Patumanon, Predictors of renal involvement in patients with systemic lupus erythematosus, Asian Pac. J. Allergy Immunol., 2007, 25, 17–25 CAS.
- A. Lanas, J. L. Goldstein, F. K. Chan, C. M. Wilcox, D. A. Peura, C. Li, G. H. Sands and J. M. Scheiman, Risk factors associated with a decrease >/= 2 g/dL in haemoglobin and/or >/= 10% haematocrit in osteoarthritis patients taking celecoxib or a nonselective NSAID plus a PPI in a large randomised controlled trial (CONDOR), Aliment. Pharmacol. Ther., 2012, 36, 485–492 CrossRef CAS PubMed.
- C. Irace, C. Carallo, F. Scavelli, M. S. De Franceschi, T. Esposito and A. Gnasso, Blood viscosity in subjects with normoglycemia and prediabetes, Diabetes care, 2014, 37, 488–492, DOI:10.2337/dc13-1374.
- D. E. Furst, H. Chang, J. D. Greenberg, V. K. Ranganath, G. Reed, Z. E. Ozturk and J. M. Kremer, Prevalence of low hemoglobin levels and associations with other disease parameters in rheumatoid arthritis patients: evidence from the CORRONA registry, Clin. Exp. Rheumatol., 2009, 27, 560–566 CAS.
- A. Kamanlı, M. Nazıroğlu, N. Aydilek and C. Hacıevlıyagil, Plasma lipid peroxidation and antioxidant levels in patients with rheumatoid arthritis, Cell Biochem. Funct., 2004, 22, 53–57 CrossRef PubMed.
- L. Kellert, C. Herweh, M. Sykora, P. Gussmann, E. Martin, P. A. Ringleb, T. Steiner and J. Bosel, Loss of Penumbra by Impaired Oxygen Supply? Decreasing Hemoglobin Levels Predict Infarct Growth after Acute Ischemic Stroke: Stroke: Relevant Impact of Hemoglobin, Hematocrit and Transfusion (STRAIGHT) - An Observational Study, Cerebrovasc. Dis. Extra, 2012, 2, 99–107 CrossRef CAS PubMed.
- L. Eischer, V. Tscholl, G. Heinze, L. Traby, P. A. Kyrle and S. Eichinger, Hematocrit and the risk of recurrent venous thrombosis: a prospective cohort study, PLoS One, 2012, 7, e38705 CAS.
- M. J. Simmonds, H. J. Meiselman and O. K. Baskurt, Blood rheology and aging, J. Geriatr. Cardiol., 2013, 10, 291–301 Search PubMed.
- N. N. Barvitenko, M. Aslam, J. Filosa, E. Matteucci, M. Nikinmaa, A. Pantaleo, C. Saldanha and O. K. Baskurt, Tissue oxygen demand in regulation of the behavior of the cells in the vasculature, Microcirculation, 2013, 20, 484–501 CrossRef CAS PubMed.
- S. Schuster and H. Stark, What can we learn from Einstein and Arrhenius about the optimal flow of our blood?, Biochim. Biophys. Acta, 2014, 1840, 271–276 CrossRef CAS PubMed.
- L. N. Toksvang and R. M. Berg, Using a classic paper by Robin Fahraeus and Torsten Lindqvist to teach basic hemorheology, Adv. Physiol. Educ., 2013, 37, 129–133 CrossRef PubMed.
- O. K. Baskurt and H. J. Meiselman, Blood rheology and hemodynamics, Semin. Thromb. Hemostasis, 2003, 29, 435–450 CrossRef CAS PubMed.
- O. K. Baskurt, O. Yalcin and H. J. Meiselman, Hemorheology and vascular control mechanisms, Clin. Hemorheol. Microcirc., 2004, 30, 169–178 Search PubMed.
- M. L. Bassett, Haemochromatosis: iron still matters, Intern. Med. J., 2001, 31, 237–242 CrossRef CAS.
- H. J. Meiselman, B. Neu, M. W. Rampling and O. K. Baskurt, RBC aggregation: laboratory data and models, Indian J. Exp. Biol., 2007, 45, 9–17 CAS.
- A. Miller, M. Green and D. Robinson, Simple rule for calculating normal erythrocyte sedimentation rate, Br. Med. J., 1983, 286, 266 CrossRef CAS.
- C. J. McMahon, S. Hopkins, A. Vail, A. T. King, D. Smith, K. J. Illingworth, S. Clark, N. J. Rothwell and P. J. Tyrrell, Inflammation as a predictor for delayed cerebral ischemia after aneurysmal subarachnoid haemorrhage, J. Neurointerv. Surg., 2013, 5, 512–517 CrossRef PubMed.
- M. L. Brigden, Clinical utility of the erythrocyte sedimentation rate, Am. Fam. Physician, 1999, 60, 1443–1450 CAS.
- J. Bathon, J. Graves, P. Jens, R. Hamrick and M. Mayes, The erythrocyte sedimentation rate in end-stage renal failure, Am. J. Kidney Dis, 1987, 10, 34–40 CAS.
- M. B. Andresdottir, N. Sigfusson, H. Sigvaldason and V. Gudnason, Erythrocyte sedimentation rate, an independent predictor of coronary heart disease in men and women: The Reykjavik Study, Am. J. Epidemiol., 2003, 158, 844–851 CrossRef PubMed.
- T. Skogh, D. Gustafsson, M. Kjellberg and M. Husberg, Twenty eight joint count disease activity score in recent onset rheumatoid arthritis using C reactive protein instead of erythrocyte sedimentation rate, Ann. Rheum. Dis., 2003, 62, 681–682 CrossRef CAS.
- R. Skalak, P. R. Zarda, K. M. Jan and S. Chien, Mechanics of Rouleau formation, Biophys. J., 1981, 35, 771–781 CrossRef CAS.
- H. Beving, L. E. Eriksson, C. L. Davey and D. B. Kell, Dielectric properties of human blood and erythrocytes at radio frequencies (0.2–10 MHz); dependence on cell volume fraction and medium composition, Eur. Biophys. J., 1994, 23, 207–215 CrossRef CAS.
- M. Musielak and J. Koscielak, Different RBC aggregating properties of the Aalpha2Bbeta2gammaA2 and Aalpha2Bbeta2gammaAgamma' subpopulations of human fibrinogen, Clin. Hemorheol. Microcirc., 2006, 35, 301–303 CAS.
- R. Ben Ami, G. Barshtein, D. Zeltser, Y. Goldberg, I. Shapira, A. Roth, G. Keren, H. Miller, V. Prochorov, A. Eldor, S. Berliner and S. Yedgar, Parameters of red blood cell aggregation as correlates of the inflammatory state, Am. J. Physiol.: Heart Circ. Physiol., 2001, 280, H1982–H1988 CAS.
- C. J. Schlimp and H. Schöchl, The role of fibrinogen in trauma-induced coagulopathy, Hamostaseologie, 2014, 34, 29–39, DOI:10.5482/HAMO-13-07-0038.
- M. A. Meyer, S. R. Ostrowski, N. A. Windelov and P. I. Johansson, Fibrinogen concentrates for bleeding trauma patients: what is the evidence?, Vox Sang., 2011, 101, 185–190 CrossRef CAS PubMed.
- D. Fries and W. Z. Martini, Role of fibrinogen in trauma-induced coagulopathy, Br. J. Anaesth., 2010, 105, 116–121 CrossRef CAS PubMed.
- M. Pavlovic, D. Babic, P. Rastovic and I. Ljevak, Association of erythrocyte sedimentation rate and fibrinogen concentration with metabolic syndrome in schizophrenic patients, Psychiatr. Danubina, 2013, 25(suppl 1), 51–55 CAS.
-
O. N. Baskurt, B. Neu and H. J. Meiselman, Red Blood Cell Aggregation, CRC Press, Boca Raton, 2011 Search PubMed.
- G. Késmárky, P. Kenyeres, M. Késmárky and K. Tóth, Plasma viscosity: a forgotten variable, Clin. Hemorheol. Microcirc., 2008, 39, 243–246 Search PubMed.
- O.
K. Baskurt and H. J. Meiselman, Iatrogenic hyperviscosity and thrombosis, Semin. Thromb. Hemostasis, 2012, 38, 854–864 CrossRef CAS PubMed.
- D. Cairncross, G. M. Collins, G. Kostalas and J. Ludbrook, Blood viscosity and erythrocyte sedimentation rate in patients with thrombotic arterial disorders, Med. J. Aust., 1969, 1, 1348–1352 CAS.
- C. Y. Chang, H. J. Liang, S. Y. Chow, S. M. Chen and D. Z. Liu, Hemorheological mechanisms in Alzheimer's disease, Microcirculation, 2007, 14, 627–634 CrossRef CAS PubMed.
- S. S. Pollock and M. J. Harrison, Red cell deformability is not an independent risk factor in stroke, J. Neurol., Neurosurg. Psychiatry, 1982, 45, 369–371 CrossRef CAS.
- I. A. Tikhomirova, A. O. Oslyakova and S. G. Mikhailova, Microcirculation and blood rheology in patients with cerebrovascular disorders, Clin. Hemorheol. Microcirc., 2011, 49, 295–305 Search PubMed.
- R. M. Johnson, Y. Ravindranath, M. S. ElAlfy and G. Goyette, Jr., Oxidant damage to erythrocyte membrane in glucose-6, phosphate, dehydrogenase, deficiency: correlation with in vivo reduced glutathione concentration and membrane protein oxidation, Blood, 1994, 83, 1117–1123 CAS.
- M. Ju, S. S. Ye, H. T. Low, J. Zhang, P. Cabrales, H. L. Leo and S. Kim, Effect of deformability difference between two erythrocytes on their aggregation, Phys. Biol., 2013, 10, 036001 CrossRef PubMed.
-
Y. Kim, K. Kim and K. Park, Measurement Techniques for Red Blood Cell Deformability: Recent Advances, in Blood Cell – An Overview of Studies in Hematology, InTech, 2012, pp. 167–195 Search PubMed.
- M. Lekka, M. Fornal, G. Pyka-Fosciak, K. Lebed, B. Wizner, T. Grodzicki and J. Styczen, Erythrocyte stiffness probed using atomic force microscope, Biorheology, 2005, 42, 307–317 Search PubMed.
- N. Mohandas and J. A. Chasis, Red blood cell deformability, membrane material properties and shape: regulation by transmembrane, skeletal and cytosolic proteins and lipids, Semin. Hematol., 1993, 30, 171–192 CAS.
- I. Sokolov, M. E. Dokukin and N. V. Guz, Method for quantitative measurements of the elastic modulus of biological cells in AFM indentation experiments, Methods, 2013, 60, 202–213 CrossRef CAS PubMed.
- Z. Zhou, C. Zheng, S. Li, X. Zhou, Z. Liu, Q. He, N. Zhang, A. Ngan, B. Tang and A. Wang, AFM nanoindentation detection of the elastic modulus of tongue squamous carcinoma cells with different metastatic potentials, Nanomedicine, 2013, 9, 864–874 CrossRef CAS PubMed.
- Y. W. Chiou, H. K. Lin, M. J. Tang, H. H. Lin and M. L. Yeh, The influence of physical and physiological cues on atomic force microscopy-based cell stiffness assessment, PLoS One, 2013, 8, e77384 CAS.
- J. Bester, A. V. Buys, B. Lipinski, D. B. Kell and E. Pretorius, High ferritin levels have major effects on the morphology of erythrocytes in Alzheimer's disease., Front. Aging Neurosci., 2013, 5, 88, DOI:10.3389/fnagi.2013.00088 . eCollection 2013.
- B. Derjaguin, V. Muller and Y. Toporov, Effect of contact deformations on the adhesion of particles., J. Colloid Interface Sci., 1975, 53, 314–326 CrossRef CAS.
- S. Park and Y. J. Lee, Nano-mechanical compliance of Muller cells investigated by atomic force microscopy, Int. J. Biol. Sci., 2013, 9, 702–706 CrossRef PubMed.
- M. E. Dokukin, N. V. Guz and I. Sokolov, Quantitative study of the elastic modulus of loosely attached cells in AFM indentation experiments, Biophys. J., 2013, 104, 2123–2131 CrossRef CAS PubMed.
- B. Lipinski, E. Pretorius, H. M. Oberholzer and W. J. van der Spuy, Interaction of fibrin with red blood cells: the role of iron, Ultrastruct. Pathol., 2012, 36, 79–84 CrossRef PubMed.
- E. Pretorius, J. Bester, N. Vermeulen, B. Lipinski, G. S. Gericke and D. B. Kell, Profound morphological changes in the erythrocytes and fibrin networks of patients with hemochromatosis or with hyperferritinemia, and their normalization by iron chelators and other agents, PLoS One, 2014, 9, e85271, DOI:10.1371/journal.pone.0085271 . eCollection 2014.
- E. Pretorius, J. du Plooy, P. Soma and A. Y. Gasparyan, An ultrastructural analysis of platelets, erythrocytes, white blood cells, and fibrin network in systemic lupus erythematosus, Rheumatol. Int., 2013 Search PubMed , epub ahead of print.
- E. Pretorius and B. Lipinski, Iron alters red blood cell morphology, Blood, 2013, 121, 9 CrossRef.
- P. de Moerloose, F. Boehlen and M. Neerman-Arbez, Fibrinogen and the risk of thrombosis, Semin. Thromb. Hemostasis, 2010, 36, 7–17 CrossRef CAS PubMed.
- A. Undas, Acquired dysfibrinogenemia in atherosclerotic vascular disease, Pol. Arch. Med. Wewn., 2011, 121, 310–319 CAS.
- N. Muradashvili and D. Lominadze, Role of fibrinogen in cerebrovascular dysfunction after traumatic brain injury, Brain Inj., 2013, 27, 1508–1515, DOI:10.3109/02699052.2013.823562.
- R. A. Adams, C. Schachtrup, D. Davalos, I. Tsigelny and K. Akassoglou, Fibrinogen signal transduction as a mediator and therapeutic target in inflammation: lessons from multiple sclerosis, Curr. Med. Chem., 2007, 14, 2925–2936 CrossRef CAS.
- N. Azad, L. Agrawal, N. V. Emanuele, R. Klein, G. D. Bahn, M. McCarren, P. Reaven, R. Hayward and W. Duckworth, Association of PAI-1 and Fibrinogen with Diabetic Retinopathy in the Veterans Affairs Diabetes Trial (VADT), Diabetes Care, 2014, 37, 501–506, DOI:10.2337/dc13-1193.
- A. Bini and B. J. Kudryk, Fibrinogen and fibrin in the arterial wall, Thromb. Res., 1994, 75, 337–341 CrossRef CAS.
- M. Cortes-Canteli, J. Paul, E. H. Norris, R. Bronstein, H. J. Ahn, D. Zamolodchikov, S. Bhuvanendran, K. M. Fenz and S. Strickland, Fibrinogen and beta-amyloid association alters thrombosis and fibrinolysis: a possible contributing factor to Alzheimer's disease, Neuron, 2010, 66, 695–709 CrossRef CAS PubMed.
- D. Dávalos and K. Akassoglou, Fibrinogen as a key regulator of inflammation in disease, Semin. Immunopathol., 2012, 34, 43–62 CrossRef PubMed.
- M. Kostapanos, M. Florentin, M. S. Elisaf and D. P. Mikhailidis, Hemostatic Factors and the Metabolic Syndrome, Curr. Vasc. Pharmacol., 2013, 11, 880–905 CrossRef CAS.
- B. Hoppe and T. Dörner, Coagulation and the fibrin network in rheumatic disease: a role beyond haemostasis, Nat. Rev. Rheumatol., 2012, 8, 738–746 CrossRef CAS PubMed.
- G. Lippi, C. Mattiuzzi and E. J. Favaloro, Novel and emerging therapies: thrombus-targeted fibrinolysis, Semin. Thromb. Hemostasis, 2013, 39, 48–58 CAS.
- R. A. Ariëns, Elevated fibrinogen causes thrombosis, Blood, 2011, 117, 4687–4688 CrossRef PubMed.
- R. A. Ariëns, Fibrin(ogen) and thrombotic disease, J. Thromb. Haemostasis, 2013, 11(suppl 1), 294–305 CrossRef PubMed.
- B. Lipinski, E. Pretorius, H. M. Oberholzer and W. J. Van Der Spuy, Iron enhances generation of fibrin fibers in human blood: Implications for pathogenesis of stroke, Microsc. Res. Tech., 2012, 75, 1185–1190 CrossRef CAS PubMed.
- E. Rooth, N. H. Wallen, M. Blomback and S. He, Decreased fibrin network permeability and impaired fibrinolysis in the acute and convalescent phase of ischemic stroke, Thromb. Res., 2011, 127, 51–56 CrossRef CAS PubMed.
- R. A. Ajjan, T. Gamlen, K. F. Standeven, S. Mughal, K. Hess, K. A. Smith, E. J. Dunn, M. M. Anwar, N. Rabbani, P. J. Thornalley, H. Philippou and P. J. Grant, Diabetes is associated with posttranslational
modifications in plasminogen resulting in reduced plasmin generation and enzyme-specific activity, Blood, 2013, 122, 134–142 CrossRef CAS PubMed.
- M. Cucuianu and M. Coca, Thrombotic tendency in diabetes mellitus. Revisiting and revising a study initiated 30 years ago, Rom. J. Intern. Med., 2012, 50, 107–115 CAS.
- I. O. Blokhin and S. R. Lentz, Mechanisms of thrombosis in obesity, Curr. Opin. Hematol., 2013, 20, 437–444 CrossRef CAS PubMed.
- M. Zbczyk, J. Majewski and J. Lelakowski, Thromboembolic events are associated with prolonged clot lysis time in patients with permanent atrial fibrillation, Pol. Arch. Med. Wewn., 2011, 121, 400–407 Search PubMed.
- B. Lipinski and E. Pretorius, Novel pathway of ironinduced blood coagulation: implications for diabetes mellitus and its complications, Pol. Arch. Med. Wewn., 2012, 122, 115–122 CAS.
- R. Lassila, Role and management of coagulation disorders in peripheral arterial disease, Scand. J. Surg., 2012, 101, 94–99 CrossRef CAS PubMed.
- E. L. Grove and S. Gregersen, Antiplatelet therapy in patients with diabetes mellitus, Curr. Vasc. Pharmacol., 2012, 10, 494–505 CrossRef CAS.
- G. J. Brewer, Iron and copper toxicity in diseases of aging, particularly atherosclerosis and Alzheimer's disease, Exp. Biol. Med., 2007, 232, 323–335 CAS.
- X. Chen, T. O. Scholl and T. P. Stein, Association of elevated serum ferritin levels and the risk of gestational diabetes mellitus in pregnant women: The Camden study, Diabetes Care, 2006, 29, 1077–1082 CrossRef CAS.
- A. Gupta, A. Watkins, P. Thomas, R. Majer, N. Habubi, G. Morris and K. Pansari, Coagulation and inflammatory markers in Alzheimer's and vascular dementia, Int. J. Clin. Pract., 2005, 59, 52–57 CrossRef CAS PubMed.
- M. Y. Chan, F. Andreotti and R. C. Becker, Hypercoagulable states in cardiovascular disease, Circulation, 2008, 118, 2286–2297 CrossRef PubMed.
- J. B. Duguid, Thrombosis as a factor in the pathogenesis of coronary atherosclerosis, J. Pathol. Bacteriol., 1946, 58, 207–212 CrossRef CAS PubMed.
- E. B. Smith, Fibrinogen, fibrin and fibrin degradation products in relation to atherosclerosis, Clin. Haematol., 1986, 15, 355–370 CAS.
- D. A. Lomas, D. A. Lipson, B. E. Miller, L. Willits, O. Keene, H. Barnacle, N. C. Barnes and R. Tal-Singer, An oral inhibitor of p38 MAP kinase reduces plasma fibrinogen in patients with chronic obstructive pulmonary disease, J. Clin. Pharmacol., 2012, 52, 416–424 CrossRef CAS PubMed.
- W. MacNee, R. J. Allan, I. Jones, M. C. De Salvo and L. F. Tan, Efficacy and safety of the oral p38 inhibitor PH-797804 in chronic obstructive pulmonary disease: a randomised clinical trial, Thorax, 2013, 68, 738–745 CrossRef PubMed.
- D. Zamolodchikov and S. Strickland, Abeta delays fibrin clot lysis by altering fibrin structure and attenuating plasminogen binding to fibrin, Blood, 2012, 119, 3342–3351 CrossRef CAS PubMed.
- H. J. Ahn, D. Zamolodchikov, M. Cortes-Canteli, E. H. Norris, J. F. Glickman and S. Strickland, Alzheimer's disease peptide beta-amyloid interacts with fibrinogen and induces its oligomerization, Proc. Natl. Acad. Sci. U. S. A., 2010, 107, 21812–21817 CrossRef CAS PubMed.
- E. Pretorius, N. Vermeulen and J. Bester, Atypical erythrocytes and platelets in a patient with a pro-thrombin mutation, Platelets, 2013 Search PubMed , epub ahead of print.
- A. Undas, P. Kaczmarek, K. Sladek, E. Stpień, W. Skucha, M. Rzeszutko, I. Gorkiewicz-Kot and W. Tracz, Fibrin clot properties are altered in patients with chronic obstructive pulmonary disease. Beneficial effects of simvastatin treatment, Thromb. Haemostasis, 2009, 102, 1176–1182 CAS.
- M. Pieters, R. C. Kotze, J. C. Jerling, A. Kruger and R. A. Ariëns, Evidence that fibrinogen gamma' regulates plasma clot structure and lysis and relationship to cardiovascular risk factors in black Africans, Blood, 2013, 121, 3254–3260 CrossRef CAS PubMed.
- J. P. Collet, Y. Allali, C. Lesty, M. L. Tanguy, J. Silvain, A. Ankri, B. Blanchet, R. Dumaine, J. Gianetti, L. Payot, J. W. Weisel and G. Montalescot, Altered fibrin architecture is associated with hypofibrinolysis and premature coronary atherothrombosis, Arterioscler. Thromb. Vasc. Biol., 2006, 26, 2567–2573 CrossRef CAS PubMed.
- A. V. Buys, M. J. Van Rooy, P. Soma, D. Van Papendorp, B. Lipinski and E. Pretorius, Changes in red blood cell membrane structure in type 2 diabetes: a scanning electron and atomic force microscopy study, Cardiovasc. Diabetol., 2013, 12, 25 CrossRef PubMed.
- E. Pretorius, H. M. Oberholzer, W. J. van der Spuy, A. C. Swanepoel and P. Soma, Qualitative scanning electron microscopy analysis of fibrin networks and platelet abnormalities in diabetes, Blood Coagulation Fibrinolysis, 2011, 22, 463–467 CrossRef CAS PubMed.
- E. Pretorius, B. Lipinski, J. Bester, N. Vermeulen and P. Soma, Albumin stabilizes fibrin fiber ultrastructure in low serum albumin type 2 diabetes, Ultrastruct. Pathol., 2013, 37, 254–257 CrossRef PubMed.
- M. Bochenek, J. Zalewski, J. Sadowski and A. Undas, Type 2 diabetes as a modifier of fibrin clot properties in patients with coronary artery disease, J. Thromb. Thrombolysis, 2013, 35, 264–270 CrossRef CAS PubMed.
- E. J. Dunn, H. Philippou, R. A. Ariëns and P. J. Grant, Molecular mechanisms involved in the resistance of fibrin to clot lysis by plasmin in subjects with type 2 diabetes mellitus, Diabetologia, 2006, 49, 1071–1080 CrossRef CAS PubMed.
- P. K. Dhillon and M. J. Adams, Thrombosis in systemic lupus erythematosus: role of impaired fibrinolysis, Semin. Thromb. Hemostasis, 2013, 39, 434–440 CrossRef CAS PubMed.
- A. Y. Gasparyan, L. Ayvazyan, E. Pretorius and G. D. Kitas, Platelets in Rheumatic Diseases: Friend or Foe?, Curr. Pharm. Des., 2013 Search PubMed , epub ahead of print.
- E. Pretorius, H. M. Oberholzer, W. J. van der Spuy, A. C. Swanepoel and P. Soma, Scanning electron microscopy of fibrin networks in rheumatoid arthritis: a qualitative analysis, Rheumatol. Int., 2012, 32, 1611–1615 CrossRef CAS PubMed.
- B. Kwasny-Krochin, P. Gluszko and A. Undas, Unfavorably altered fibrin clot properties in patients with active rheumatoid arthritis, Thromb. Res., 2010, 126, e11–e16 CrossRef CAS PubMed.
- J. J. Marsh, P. G. Chiles, N. C. Liang and T. A. Morris, Chronic thromboembolic pulmonary hypertension-associated dysfibrinogenemias exhibit disorganized fibrin structure, Thromb. Res., 2013, 132, 729–734, DOI:10.1016/j.thromres.2013.09.024.
- B. Lipinski and E. Pretorius, Hydroxyl radical-modified fibrinogen as a marker of thrombosis: the role of iron, Hematology, 2012, 17, 241–247 CrossRef CAS PubMed.
- B. Lipinski and E. Pretorius, Iron-induced fibrin in cardiovascular disease, Curr. Neurovasc. Res., 2013, 10, 269–274 CrossRef CAS.
- E. Pretorius, Quantifying changes in fibrin fiber network morphology, Ultrastruct. Pathol., 2011, 35, 150–154 CrossRef PubMed.
- A. Undas, A. Slowik, P. Wolkow, A. Szczudlik and W. Tracz, Fibrin clot properties in acute ischemic stroke: relation to neurological deficit, Thromb. Res., 2010, 125, 357–361 CrossRef CAS PubMed.
- A. Undas, P. Podolec, K. Zawilska, M. Pieculewicz, I. Jedliński, E. Stepień, E. Konarska-Kuszewska, P. Weglarz, M. Duszyńska, E. Hanschke, T. Przewlocki and W. Tracz, Altered fibrin clot structure/function in patients with cryptogenic ischemic stroke, Stroke, 2009, 40, 1499–1501 CrossRef CAS PubMed.
- M. Stalc, M. Tomsic, M. K. Jezovnik and P. Poredos, Endothelium-dependent and independent dilation capability of peripheral arteries in patients with systemic lupus erythematosus and antiphospholipid syndrome, Clin. Exp. Rheumatol., 2011, 29, 616–623 CAS.
- P. R. Ames, L. Iannaccone, J. D. Alves, A. Margarita, L. R. Lopez and V. Brancaccio, Factor XIII in primary antiphospholipid syndrome, J. Rheumatol., 2005, 32, 1058–1062 CAS.
- P. de Pablo, A. Ramirez, E. Cortina, A. de la Pena, J. Zamora, R. Izaguirre and M. C. Amigo, Increased fibrin polymerization rate in patients with primary antiphospholipid syndrome and systemic lupus erythematosus, Clin. Appl. Thromb./Hemostasis, 2003, 9, 221–225 CrossRef CAS PubMed.
- P. R. Ames, J. Alves, A. F. Pap, P. Ramos, M. A. Khamashta and G. R. Hughes, Fibrinogen in systemic lupus erythematosus: more than an acute phase reactant?, J. Rheumatol., 2000, 27, 1190–1195 CAS.
- M. van Oijen, J. C. Witteman, A. Hofman, P. J. Koudstaal and M. M. Breteler, Fibrinogen is associated with an increased risk of Alzheimer disease and vascular dementia, Stroke, 2005, 36, 2637–2641 CrossRef CAS PubMed.
- N. Papageorgiou, D. Tousoulis, A. Miliou, G. Hatzis, M. Kozanitou, E. Androulakis, M. Charakida, A. Antonopoulos, C. Antoniades, A. Briasoulis, A. Giolis, G. Bouras, Z. Pallantza and C. Stefanadis, Combined effects of fibrinogen genetic variability on atherosclerosis in patients with or without stable angina pectoris: Focus on the coagulation cascade and endothelial function, Int. J. Cardiol., 2013, 168, 4602–4607 CrossRef PubMed.
- K. Oszajca, K. Wronski, G. Janiszewska, M. Bienkiewicz, M. Panek, J. Bartkowiak and J. Szemraj, Association analysis of genetic polymorphisms of factor V, factor VII and fibrinogen beta chain genes with human abdominal aortic aneurysm, Exp. Ther. Med., 2012, 4, 514–518 CAS.
- P. M. Ridker, C. P. Howard, V. Walter, B. Everett, P. Libby, J. Hensen and T. Thuren, Effects of interleukin-1beta inhibition with canakinumab on hemoglobin A1c, lipids, C-reactive protein, interleukin-6, and fibrinogen: a phase IIb randomized, placebo-controlled trial, Circulation, 2012, 126, 2739–2748 CrossRef CAS PubMed.
- J. Kolodziejczyk and M. B. Ponczek, The role of fibrinogen, fibrin and fibrin(ogen) degradation products (FDPs) in tumor progression, Contemp. Oncol., 2013, 17, 113–119 CAS.
- A. T. Toriola, J. A. Laukkanen, S. Kurl, K. Nyyssonen, K. Ronkainen and J. Kauhanen, Prediagnostic circulating markers of inflammation and risk of prostate cancer, Int. J. Cancer, 2013, 133, 2961–2967 CAS.
- L. Sheng, M. Luo, X. Sun, N. Lin, W. Mao and D. Su, Serum fibrinogen is an independent prognostic factor in operable nonsmall cell lung cancer, Int. J. Cancer, 2013, 133, 2720–2725 CrossRef CAS PubMed.
- T. Yoneyama, S. Ohtsuki, M. Ono, K. Ohmine, Y. Uchida, T. Yamada, M. Tachikawa and T. Terasaki, Quantitative targeted absolute proteomics-based large-scale quantification of proline-hydroxylated alpha-fibrinogen in plasma for pancreatic cancer diagnosis, J. Proteome Res., 2013, 12, 753–762 CrossRef CAS PubMed.
- A. Agustí, L. D. Edwards, S. I. Rennard, W. MacNee, R. Tal-Singer, B. E. Miller, J. Vestbo, D. A. Lomas, P. M. Calverley, E. Wouters, C. Crim, J. C. Yates, E. K. Silverman, H. O. Coxson, P. Bakke, R. J. Mayer and B. Celli, Persistent systemic inflammation is associated with poor clinical outcomes in COPD: a novel phenotype, PLoS One, 2012, 7, e37483 Search PubMed.
- P. J. Barnes and B. R. Celli, Systemic manifestations and comorbidities of COPD, Eur. Respir. J., 2009, 33, 1165–1185 CrossRef CAS PubMed.
- M. Dahl, A. Tybjærg-Hansen, J. Vestbo, P. Lange and B. G. Nordestgaard, Elevated plasma fibrinogen associated with reduced pulmonary function and increased risk of chronic obstructive pulmonary disease, Am. J. Respir. Crit. Care Med., 2001, 164, 1008–1011 CrossRef CAS PubMed.
- G. C. Donaldson, T. A. Seemungal, I. S. Patel, A. Bhowmik, T. M. Wilkinson, J. R. Hurst, P. K. Maccallum and J. A. Wedzicha, Airway and systemic inflammation and decline in lung function in patients with COPD, Chest, 2005, 128, 1995–2004 CrossRef PubMed.
- A. Duvoix, J. Dickens, I. Haq, D. Mannino, B. Miller, R. Tal-Singer and D. A. Lomas, Blood fibrinogen as a biomarker of chronic obstructive pulmonary disease, Thorax, 2013, 68, 670–676 CrossRef PubMed.
- W. Q. Gan, S. F. P. Man, A. Senthilselvan and D. D. Sin, Association between chronic obstructive pulmonary disease and systemic inflammation: a systematic review and a meta-analysis, Thorax, 2004, 59, 574–580 CrossRef CAS.
- F. Garcia-Rio, M. Miravitlles, J. B. Soriano, L. Muñoz, E. Duran-Tauleria, G. Sánchez, V. Sobradillo and J. Ancochea, Systemic inflammation in chronic obstructive pulmonary disease: a population-based study, Respir. Res., 2010, 11, 63 CrossRef PubMed.
- K. H. Groenewegen, D. S. Postma, W. C. Hop, P. L. Wielders, N. J. Schlosser and E. F. Wouters, Increased systemic inflammation is a risk factor for COPD exacerbations, Chest, 2008, 133, 350–357 CrossRef CAS PubMed.
- B. Lazovic, Correlation of CRP and serum level of fibrinogen with severity of disease in chronic obstructive pulmonary disease patients, Med. Arh., 2012, 66, 159–160 CrossRef PubMed.
- D. M. Mannino, D. Valvi, H. Mullerova and R. Tal-Singer, Fibrinogen, COPD and mortality in a nationally representative U.S. cohort, COPD, 2012, 9, 359–366 Search PubMed.
- B. L. Nicholas, Search for biomarkers in chronic obstructive pulmonary disease: current status, Curr. Opin. Pulm. Med., 2013, 19, 103–108 CrossRef CAS PubMed.
- E. Pizzichini, M. M. Pizzichini, P. Gibson, K. Parameswaran, G. J. Gleich, L. Berman, J. Dolovich and F. E. Hargreave, Sputum eosinophilia predicts benefit from prednisone in smokers with chronic obstructive bronchitis, Am. J. Respir. Crit. Care Med., 1998, 158, 1511–1517 CrossRef CAS PubMed.
- M. Polatli, A. Cakir, O. Cildag, A. Z. Bolaman, C. Yenisey and Y. Yenicerioglu, Microalbuminuria, von Willebrand factor and fibrinogen levels as markers of the severity in COPD exacerbation, J. Thromb. Thrombolysis, 2008, 26, 97–102 CrossRef CAS PubMed.
- T. Seemungal, R. Harper-Owen, A. Bhowmik, I. Moric, G. Sanderson, S. Message, P. Maccallum, T. W. Meade, D. J. Jeffries, S. L. Johnston and J. A. Wedzicha, Respiratory viruses, symptoms, and inflammatory markers in acute exacerbations and stable chronic obstructive pulmonary disease, Am. J. Respir. Crit. Care Med., 2001, 164, 1618–1623 CrossRef CAS PubMed.
- Y. Shibata, S. Abe, S. Inoue, A. Igarashi, K. Yamauchi, Y. Aida, H. Kishi, K. Nunomiya, H. Nakano, M. Sato, K. Sato, T. Kimura, T. Nemoto, T. Watanabe, T. Konta, Y. Ueno, T. Kato, T. Kayama and I. Kubota, Relationship between plasma fibrinogen levels and pulmonary function in the Japanese population: the Takahata study, Int. J. Med. Sci., 2013, 10, 1530–1536 CrossRef PubMed.
- D. D. Sin and S. F. P. Man, Why are patients with chronic obstructive pulmonary disease at increased risk of cardiovascular diseases? The potential role of systemic inflammation in chronic obstructive pulmonary disease, Circulation, 2003, 107, 1514–1519 CrossRef.
- N. J. Sinden and R. A. Stockley, Systemic inflammation and comorbidity in COPD: a result of ‘overspill’ of inflammatory mediators from the lungs? Review of the evidence, Thorax, 2010, 65, 930–936 CrossRef PubMed.
- B. Thyagarajan, D. R. Jacobs, G. G. Apostol, L. J. Smith, C. E. Lewis and O. D. Williams, Plasma fibrinogen and lung function: the CARDIA Study, Int. J. Epidemiol., 2006, 35, 1001–1008 CrossRef PubMed.
- D. Valvi, D. M. Mannino, H. Mullerova and R. Tal-Singer, Fibrinogen chronic obstructive pulmonary disease (COPD) and outcomes in two United States cohorts, Int. J. Chronic Obstruct. Pulm. Dis., 2012, 7, 173–182 CAS.
- J. A. Wedzicha, T. A. R. Seemungal, P. K. MacCallum, E. A. Paul, G. C. Donaldson, A. Bhowmik, D. J. Jeffries and T. W. Meade, Acute exacerbations of chronic obstructive pulmonary disease are accompanied by elevations of plasma fibrinogen and serum IL-6 levels, Thromb. Haemostasis, 2000, 84, 210–215 CAS.
- O. Morel, L. Jesel, M. Abbas and N. Morel, Prothrombotic Changes in Diabetes Mellitus, Semin. Thromb. Hemostasis, 2013, 39, 477–488, DOI:10.1055/s-0033-1343888.
- P. Gyawali, R. S. Richards and E. Uba Nwose, Erythrocyte morphology in metabolic syndrome, Expert Rev. Hematol., 2012, 5, 523–531 CrossRef CAS PubMed.
- X. Zhang, S. C. McGeoch, A. M. Johnstone, G. Holtrop, A. A. Sneddon, S. M. Macrury, I. L. Megson, D. W. Pearson, P. Abraham, B. De Roos, G. E. Lobley and N. O'Kennedy, Platelet-derived microparticle count and surface molecule expression differ between subjects with and without type 2 diabetes, independently of obesity status, J. Thromb. Thrombolysis, 2013 Search PubMed , epub ahead of print.
- T. M. Okwuosa, O. Klein, C. Chan, N. S. Jenny, P. Schreiner, D. Green and K. Liu, 13-year long-term associations between changes in traditional cardiovascular risk factors and changes in fibrinogen levels: the Coronary Artery Risk Development in Young Adults (CARDIA) study, Atherosclerosis, 2013, 226, 214–219 CrossRef CAS PubMed.
- A. N. Kul, S. Ozdemir, A. Helvaci, C. Bulut and S. Dursun, The Relationship of Acute Myocardial Infarction With or Without ST-Segment Elevation and Viscosity, Clin. Appl. Thromb./Hemostasis, 2013 Search PubMed , epub ahead of print.
- O. Akgul, H. Uyarel, H. Pusuroglu, M. Gul, N. Isiksacan, S. Turen, M. Erturk, O. Surgit, M. Cetin, U. Bulut, O. F. Baycan and N. Uslu, Predictive value of elevated D-dimer in patients undergoing primary angioplasty for ST elevation myocardial infarction, Blood Coagulation Fibrinolysis, 2013, 24, 704–710 CrossRef CAS PubMed.
- R. Kaiser, Y. Li, M. Chang, J. Catanese, A. B. Begovich, E. E. Brown, J. C. Edberg, G. McGwin, Jr., G. S. Alarcon, R. Ramsey-Goldman, J. D. Reveille, L. M. Vila, M. A. Petri, R. P. Kimberly, K. E. Taylor and L. A. Criswell, Genetic risk factors for thrombosis in systemic lupus erythematosus, J. Rheumatol., 2012, 39, 1603–1610 CrossRef PubMed.
- G. Lippi, E. J. Favaloro and M. Franchini, Paradoxical thrombosis part 1: factor replacement therapy, inherited clotting factor deficiencies and prolonged APTT, J. Thromb. Thrombolysis, 2012, 34, 360–366 CrossRef CAS PubMed.
- A. Vayá, J. Calvo, C. Alcalá, L. Micó, J. Todolí and J. M. Ricart, Rheological alterations and thrombotic events in patients with systemic lupus erythematosus, Clin. Hemorheol. Microcirc., 2012, 51, 51–58 Search PubMed.
- A. Vayá, J. Murado, M. Santaolaria, M. Simo, L. Mico, J. Calvo, J. Todolí and J. M. Ricart, Haemorheological
changes in patients with systemic lupus erythematosus do not seem to be related to thrombotic events, Clin. Hemorheol. Microcirc., 2008, 38, 23–29 Search PubMed.
- E. Corrado, M. Rizzo, G. Caccamo, G. Coppola, I. Muratori, R. Tantillo, S. Dell'oglio, C. Nugara, F. Macaione, G. Novo, R. Alcamo, A. Mignano, F. Ferrara and S. Novo, Prevalence of metabolic syndrome: a comparative analysis in an unselected sample of mediterranean subjects, Int. Angiol., 2012, 31, 572–578 CAS.
- M. Maksimović, H. Vlajinac, D. Radak, J. Marinković, J. Maksimović and J. Jorga, Relationship between abdominal obesity and other cardiovascular risk factors: cross sectional study of patients with symptomatic carotid disease, Srp. Arh. Celok. Lek., 2013, 141, 460–465 CrossRef.
- G. G. Korkmaz, E. Altinoglu, S. Civelek, V. Sozer, F. Erdenen, O. Tabak and H. Uzun, The association of oxidative stress markers with conventional risk factors in the metabolic syndrome, Metab., Clin. Exp., 2013, 62, 828–835 CrossRef CAS PubMed.
- B. E. Gilliam, M. R. Reed, A. K. Chauhan, A. B. Dehlendorf and T. L. Moore, Evidence of fibrinogen as a target of citrullination in IgM rheumatoid factor-positive polyarticular juvenile idiopathic arthritis, Pediatr. Rheumatol. Online J., 2011, 9, 8 CrossRef PubMed.
- F. Lv, L. J. Song and X. F. Li, Combined measurement of multiple acute phase reactants to predict relapse of rheumatoid arthritis, Int. J. Rheum. Dis., 2013 DOI:10.1111/1756-185X.12186.
- K. Shin, S. Hong, E. H. Choi, M. K. Lim, S. C. Shim, J. H. Ju and S. H. Lee, Role of citrullinated fibrinogen peptides in the activation of CD4 T cells from patients with rheumatoid arthritis, Immune Netw., 2013, 13, 116–122 CrossRef PubMed.
- W. B. Kannel, P. A. Wolf, W. P. Castelli and R. B. D'Agostino, Fibrinogen and risk of cardiovascular disease. The Framingham Study, JAMA, J. Am. Med. Assoc., 1987, 258, 1183–1186 CrossRef CAS.
- J. Zhang, L. Yu, Y. Yin, Q. Lu, L. Lei, J. Xiao, J. Guo, J. Zhao, Y. Wang, G. He, Y. Xu and L. He, Association between two functional fibrinogen-related polymorphisms and ischemic stroke: a case-control study, Genet. Test. Mol. Biomarkers, 2013, 17, 789–793 CrossRef CAS PubMed.
- A. Lasek-Bal, P. Puz and Z. Kazibutowska, Elevated factor VIII level and stroke in patients without traditional risk factors associated with cardiovascular diseases, Neuropsychiatr. Dis. Treat., 2013, 9, 847–852 CrossRef PubMed.
- E. Pretorius, H. Steyn, M. Engelbrecht, A. C. Swanepoel and H. M. Oberholzer, Differences in fibrin fiber diameters in healthy individuals and thromboembolic ischemic stroke patients, Blood Coagulation Fibrinolysis, 2011, 22, 696–700 CrossRef PubMed.
- E. Pretorius and B. Lipinski, Differences in Morphology of Fibrin Clots Induced with Thrombin and Ferric Ions and Its Pathophysiological Consequences, Heart, Lung Circ., 2012, 22, 447–449 CrossRef PubMed.
- A. Undas, K. Szuldrzynski, E. Stepien, J. Zalewski, J. Godlewski, W. Tracz, M. Pasowicz and K. Zmudka, Reduced clot permeability and susceptibility to lysis in patients with acute coronary syndrome: effects of inflammation and oxidative stress, Atherosclerosis, 2008, 196, 551–557 CrossRef CAS PubMed.
- P. Allan, S. Uitte de Willige, R. H. Abou-Saleh, S. D. Connell and R. A. Ariëns, Evidence that fibrinogen gamma' directly interferes with protofibril growth: implications for fibrin structure and clot stiffness, J. Thromb. Haemostasis, 2012, 10, 1072–1080 CrossRef CAS PubMed.
- J. R. Houser, N. E. Hudson, L. Ping, E. T. O'Brien, 3rd, R. Superfine, S. T. Lord and M. R. Falvo, Evidence that alphaC region is origin of low modulus, high extensibility,
and strain stiffening in fibrin fibers, Biophys. J., 2010, 99, 3038–3047 CrossRef CAS PubMed.
- L. Medved, S. Litvinovich, T. Ugarova, Y. Matsuka and K. Ingham, Domain structure and functional activity of the recombinant human fibrinogen gamma-module (gamma148-411), Biochemistry, 1997, 36, 4685–4693 CrossRef CAS PubMed.
- J. P. Collet, J. L. Moen, Y. I. Veklich, O. V. Gorkun, S. T. Lord, G. Montalescot and J. W. Weisel, The alphaC domains of fibrinogen affect the structure of the fibrin clot, its physical properties, and its susceptibility to fibrinolysis, Blood, 2005, 106, 3824–3830 CrossRef CAS PubMed.
- L. Medved and J. W. Weisel, Recommendations for nomenclature on fibrinogen and fibrin, J. Thromb. Haemostasis, 2009, 7, 355–359 CrossRef CAS PubMed.
- E. A. Ryan, L. F. Mockros, J. W. Weisel and L. Lorand, Structural origins of fibrin clot rheology, Biophys. J., 1999, 77, 2813–2826 CrossRef CAS.
- E. Pretorius, S. Briedenhann, J. Marx and R. C. Franz, Structural changes in the fibrin network of a pretoria family with dysfibrinogenemia: a scanning electron microscopical study, Ultrastruct. Pathol., 2006, 30, 167–176 CrossRef CAS PubMed.
- M. Matsuda and T. Sugo, Hereditary disorders of fibrinogen, Ann. N. Y. Acad. Sci., 2001, 936, 65–88 CrossRef CAS.
- Z. Yang, I. Mochalkin and R. F. Doolittle, A model of fibrin formation based on crystal structures of fibrinogen and fibrin fragments complexed with synthetic peptides, Proc. Natl. Acad. Sci. U. S. A., 2000, 97, 14156–14161 CrossRef CAS PubMed.
- R. S. Lovely, L. A. Falls, H. A. Al-Mondhiry, C. E. Chambers, G. J. Sexton, H. Ni and D. H. Farrell, Association of gammaA/gamma' fibrinogen levels and coronary artery disease, Thromb. Haemostasis, 2002, 88, 26–31 CAS.
- E. Y. Cheung, S. Uitte de Willige, H. L. Vos, F. W. Leebeek, D. W. Dippel, R. M. Bertina and M. P. de Maat, Fibrinogen gamma' in ischemic stroke: a case-control study, Stroke, 2008, 39, 1033–1035 CrossRef CAS PubMed.
- I. Parastatidis, L. Thomson, A. Burke, I. Chernysh, C. Nagaswami, J. Visser, S. Stamer, D. C. Liebler, G. Koliakos, H. F. Heijnen, G. A. Fitzgerald, J. W. Weisel and H. Ischiropoulos, Fibrinogen beta-chain tyrosine nitration is a prothrombotic risk factor, J. Biol. Chem., 2008, 283, 33846–33853 CrossRef CAS PubMed.
- C. Vadseth, J. M. Souza, L. Thomson, A. Seagraves, C. Nagaswami, T. Scheiner, J. Torbet, G. Vilaire, J. S. Bennett, J. C. Murciano, V. Muzykantov, M. S. Penn, S. L. Hazen, J. W. Weisel and H. Ischiropoulos, Pro-thrombotic state induced by post-translational modification of fibrinogen by reactive nitrogen species, J. Biol. Chem., 2004, 8820–8828 CrossRef CAS PubMed.
- M. Piagnerelli, K. Zouaoui Boudjeltia, D. Brohee, A. Vereerstraeten, P. Piro, J. L. Vincent and M. Vanhaeverbeek, Assessment of erythrocyte shape by flow cytometry techniques, J. Clin. Pathol., 2007, 60, 549–554 CrossRef CAS PubMed.
- M. Piagnerelli, F. Cotton, M. Van Nuffelen, J. L. Vincent and B. Gulbis, Modifications in erythrocyte membrane protein content are not responsible for the alterations in rheology seen in sepsis, Shock, 2012, 37, 17–21 CrossRef CAS PubMed.
- A. P. DeFilippis, K. Law, S. Curtin and J. R. Eckman, Blood is thicker than water: the management of hyperviscosity in adults with cyanotic heart disease, Cardiol. Rev., 2007, 15, 31–34 CrossRef PubMed.
- D. Pulanic and I. Rudan, The past decade: fibrinogen, Coll. Antropol., 2005, 29, 341–349 Search PubMed.
- P. M. Moriarty and C. A. Gibson, Association between hematological parameters and high-density lipoprotein cholesterol, Curr. Opin. Cardiol., 2005, 20, 318–323 CrossRef.
- G. D. Sloop and D. E. Mercante, Opposite effects of low-density and high-density lipoprotein on blood viscosity in fasting subjects, Clin. Hemorheol. Microcirc., 1998, 19, 197–203 CAS.
- R. B. Ami, G. Barshtein, D. Zeltser, Y. Goldberg, I. Shapira, A. Roth, G. Keren, H. Miller, V. Prochorov, A. Eldor, S. Berliner and S. Yedgar, Parameters of red blood cell aggregation as correlates of the inflammatory state, Am. J. Physiol.: Heart Circ. Physiol., 2001, 280, H1982–H1988 CAS.
- D. Justo, N. Mashav, Y. Arbel, M. Kinori, A. Steinvil, M. Swartzon, B. Molat, A. Halkin, A. Finkelstein, R. Heruti and S. Banai, Increased erythrocyte aggregation in men with coronary artery disease and erectile dysfunction, Int. J. Impotence Res., 2009, 21, 192–197 CrossRef CAS PubMed.
- N. Bolokadze, I. Lobjanidze, N. Momtselidze, R. Shakarishvili and G. McHedlishvili, Comparison of erythrocyte aggregability changes during ischemic and hemorrhagic stroke, Clin. Hemorheol. Microcirc., 2006, 35, 265–267 CAS.
- M. A. Elblbesy, A. R. Hereba and M. M. Shawki, Effects of aspirin on rheological properties of erythrocytes in vitro, Int. J. Biomed. Sci., 2012, 8, 188–193 CAS.
- A. G. Atici, S. Kayhan, D. Aydin and Y. A. Yilmaz, Plasma viscosity levels in pulmonary thromboembolism, Clin. Hemorheol. Microcirc., 2013, 55, 313–320, DOI:10.3233/CH-2012-1637.
- F. Bajd, J. Vidmar, A. Blinc and I. Sersa, Analysis of blood clot degradation fragment sizes in relation to plasma flow velocity, Gen. Physiol. Biophys., 2012, 31, 237–245 CrossRef PubMed.
- R. Holsworth, Jr., Y. Cho, J. J. Weidman, G. Sloop and J. St Cyr, Cardiovascular benefits of phlebotomy: relationship to changes in hemorheological variables, Perfusion, 2014, 29, 102–116, DOI:10.1177/0267659113505637.
- A. Somani, M. E. Steiner and R. P. Hebbel, The dynamic regulation of microcirculatory conduit function: features relevant to transfusion medicine, Transfus. Apher. Sci., 2010, 43, 61–68 CrossRef PubMed.
- R. M. Olinescu and F. A. Kummerow, Fibrinogen is an efficient antioxidant, J. Nutr. Biochem., 2001, 12, 162–169 CrossRef CAS.
- Y. H. Feng and G. Hart, In vitro oxidative damage to tissue-type plasminogen activator: a selective modification of the biological functions, Cardiovasc. Res., 1995, 30, 255–261 CrossRef CAS.
- G. R. Upchurch Jr, N. Ramdev, M. T. Walsh and J. Loscalzo, Prothrombotic Consequences of the Oxidation of Fibrinogen and their Inhibition by Aspirin, J. Thromb. Thrombolysis, 1998, 5, 9–14 CrossRef.
- P. Kanwar and K. V. Kowdley, Diagnosis and treatment of hereditary hemochromatosis: an update, Expert Rev. Gastroenterol. Hepatol., 2013, 7, 517–530 CrossRef CAS PubMed.
- J. K. Limdi and J. R. Crampton, Hereditary haemochromatosis, QJM, 2004, 97, 315–324 CrossRef CAS.
- S. Lynch and G. Soslau, Iron levels found in hemochromatosis patients inhibit gamma-thrombin-induced platelet aggregation, Platelets, 2012, 23, 611–616 CrossRef CAS PubMed.
- G. D. McLaren and V. R. Gordeuk, Hereditary hemochromatosis: insights from the Hemochromatosis and Iron Overload Screening (HEIRS) Study, Hematology Am. Soc. Hematol. Educ. Program, 2009, 195–206 CrossRef PubMed.
- P. Nielsen, U. Günther, M. Dürken, R. Fischer and J. Düllmann, Serum ferritin iron in iron overload and liver damage: correlation to body iron stores and diagnostic relevance, J. Lab. Clin. Med., 2000, 135, 413–418 CrossRef CAS PubMed.
- J. Waalen, V. J. Felitti, T. Gelbart and E. Beutler, Screening for hemochromatosis by measuring ferritin levels: a more effective approach, Blood, 2008, 111, 3373–3376 CrossRef CAS PubMed.
- G. Zandman-Goddard and Y. Shoenfeld, Hyperferritinemia in autoimmunity, Isr. Med. Assoc. J., 2008, 10, 83–84 Search PubMed.
- P. Adams, Management of elevated serum ferritin levels, Gastroenterol. Hepatol., 2008, 4, 333–334 Search PubMed.
- N. Ahluwalia, A. Genoux, J. Ferrieres, B. Perret, M. Carayol, L. Drouet and J. B. Ruidavets, Iron status is associated with carotid atherosclerotic plaques in middle-aged adults, J. Nutr., 2010, 140, 812–816 CrossRef CAS PubMed.
- R. G. Depalma, V. W. Hayes, B. K. Chow, G. Shamayeva, P. E. May and L. R. Zacharski, Ferritin levels, inflammatory biomarkers, and mortality in peripheral arterial disease: a substudy of the Iron (Fe) and Atherosclerosis Study (FeAST) Trial, J. Vasc. Surg., 2010, 51, 1498–1503 CrossRef PubMed.
- K. Goot, S. Hazeldine, P. Bentley, J. Olynyk and D. Crawford, Elevated serum ferritin - what should GPs know?, Aust. Fam. Physician, 2012, 41, 945–949 Search PubMed.
- L. Sun, O. H. Franco, F. B. Hu, L. Cai, Z. Yu, H. Li, X. Ye, Q. Qi, J. Wang, A. Pan, Y. Liu and X. Lin, Ferritin concentrations, metabolic syndrome, and type, 2, diabetes in middle-aged and elderly chinese, J. Clin. Endocrinol. Metab., 2008, 93, 4690–4696 CrossRef CAS PubMed.
- K. Jomova and M. Valko, Advances in metal-induced oxidative stress and human disease, Toxicology, 2011, 283, 65–87 CrossRef CAS PubMed.
- K. Jomova, D. Vondrakova, M. Lawson and M. Valko, Metals, oxidative stress and neurodegenerative disorders, Mol. Cell. Biochem., 2010, 345, 91–104 CrossRef CAS PubMed.
- E. Pretorius, N. Vermeulen, J. Bester, B. Lipinski and D. B. Kell, A novel method for assessing the role of iron and its functional chelation in fibrin fibril formation: the use of scanning electron microscopy, Toxicol. Mech. Methods, 2013, 23, 352–359 CrossRef CAS PubMed.
- I. Dulińska, M. Targosz, W. Strojny, M. Lekka, P. Czuba, W. Balwierz and M. Szymoński, Stiffness of normal and pathological erythrocytes studied by means of atomic force microscopy, J. Biochem. Biophys. Methods, 2006, 66, 1–11 CrossRef PubMed.
- J. Dupire, M. Socol and A. Viallat, Full dynamics of a red blood cell in shear flow, Proc. Natl. Acad. Sci. U. S. A., 2012, 109, 20808–20813 CrossRef CAS PubMed.
- M. P. Sheetz, R. G. Painter and S. J. Singer, Biological membranes as bilayer couples. III. Compensatory shape changes induced in membranes, J. Cell Biol., 1976, 70, 193–203 CrossRef CAS.
- O. K. Baskurt and H. J. Meiselman, Comparative hemorheology, Clin. Hemorheol. Microcirc., 2013, 53, 61–70 Search PubMed.
- J. Delaunay, The molecular basis of hereditary red cell membrane disorders, Blood Rev., 2007, 21, 1–20 CrossRef CAS PubMed.
- A. G. Moutzouri, A. T. Skoutelis, C. A. Gogos, Y. F. Missirlis and G. M. Athanassiou, Red blood cell deformability in patients with sepsis: a marker for prognosis and monitoring of severity, Clin. Hemorheol. Microcirc., 2007, 36, 291–299 Search PubMed.
- V. Bennett and J. Healy, Membrane domains based on ankyrin and spectrin associated with cell-cell interactions, Cold Spring Harbor Perspect. Biol., 2009, 1, a003012 Search PubMed.
- E. K. Kozlova, A. M. Chernysh, V. V. Moroz and A. N. Kuzovlev, Analysis of nanostructure of red blood cells membranes by space Fourier transform of AFM images, Micron, 2013, 44, 218–227, DOI:10.1016/j.micron.2012.06.012.
- M. Girasole, S. Dinarelli and G. Boumis, Structure and function in native and pathological erythrocytes: a quantitative view from the nanoscale, Micron, 2012, 43, 1273–1286 CrossRef CAS PubMed.
- E. van den Akker, T. J. Satchwell, R. C. Williamson and A. M. Toye, Band 3 multiprotein complexes in the red cell membrane; of mice and men, Blood Cells, Mol., Dis., 2010, 45, 1–8 CrossRef CAS PubMed.
- A. J. Baines, P. M. Bennett, E. W. Carter and C. Terracciano, Protein 4.1 and the control of ion channels, Blood Cells, Mol., Dis., 2009, 42, 211–215 CrossRef CAS PubMed.
- A. Czogalla and A. F. Sikorski, Do we already know how spectrin attracts ankyrin?, Cell. Mol. Life Sci., 2010, 67, 2679–2683 CrossRef CAS PubMed.
- S. R. Cunha and P. J. Mohler, Ankyrin protein networks in membrane formation and stabilization, J. Cell. Mol. Med., 2009, 13, 4364–4376 CrossRef CAS PubMed.
- N. Uyesaka, S. Hasegawa, N. Ishioka, R. Ishioka, H. Shio and A. N. Schechter, Effects of superoxide anions on red cell deformability and membrane proteins, Biorheology, 1992, 29, 217–229 CAS.
- J. P. Lopes de Almeida, T. Freitas-Santos and C. Saldanha, Erythrocyte deformability dependence on band 3 protein in an in-vitro model of hyperfibrinogenemia, Clin. Hemorheol. Microcirc., 2012, 50, 213–219 CAS.
- M. Girasole, G. Pompeo, A. Cricenti, A. Congiu-Castellano, F. Andreola, A. Serafino, B. H. Frazer, G. Boumis and G. Amiconi, Roughness of the plasma membrane as an independent morphological parameter to study RBCs: a quantitative atomic force microscopy investigation, Biochim. Biophys. Acta, 2007, 1768, 1268–1276 CrossRef CAS PubMed.
- V. V. Moroz, A. M. Chernysh, E. K. Kozlova, P. Y. Borshegovskaya, U. A. Bliznjuk, R. M. Rysaeva and O. Y. Gudkova, Comparison of red blood cell membrane microstructure after different physicochemical influences: atomic force microscope research, J. Crit. Care, 2010, 25, 539 CrossRef PubMed e531–e512.
- E. Bennett-Guerrero, T. H. Veldman, A. Doctor, M. J. Telen, T. L. Ortel, T. S. Reid, M. A. Mulherin, H. Zhu, R. D. Buck, R. M. Califf and T. J. McMahon, Evolution of adverse changes in stored RBCs, Proc. Natl. Acad. Sci. U. S. A., 2007, 104, 17063–17068 CrossRef CAS PubMed.
- E. Pretorius, J. N. du Plooy, P. Soma, I. Keyser and A. V. Buys, Smoking and fluidity of erythrocyte membranes: A high resolution scanning electron and atomic force microscopy investigation, Nitric Oxide, 2013, 35, 42–46 CrossRef CAS PubMed.
- A. S. Kamruzzahan, F. Kienberger, C. M. Stroh, J. Berg, R. Huss, A. Ebner, R. Zhu, C. Rankl, H. J. Gruber and P. Hinterdorfer, Imaging morphological details and pathological differences of red blood cells using tapping-mode AFM., Biol. Chem., 2004, 385, 955–956 CrossRef CAS PubMed.
- M. Girasole, S. Dinarelli and G. Boumis, Structural, morphological and nanomechanical characterisation of intermediate states in the ageing of erythrocytes, J. Mol. Recognit., 2012, 25, 285–291 CrossRef CAS PubMed.
- M. Girasole, G. Pompeo, A. Cricenti, G. Longo, G. Boumis, A. Bellelli and S. Amiconi, The how, when, and why of the aging signals appearing on the human erythrocyte membrane: an atomic force microscopy study of surface roughness, Nanomedicine, 2010, 6, 760–768 CrossRef CAS PubMed.
- A. M. Chernysh, E. K. Kozlova, V. V. Moroz, V. A. Sergunova, O. Y. Gudkova and M. S. Fedorova, Reversible zinc-induced injuries to erythrocyte membrane nanostructure, Bull. Exp. Biol. Med., 2012, 154, 84–88 CrossRef CAS.
- Y. Cui, Z. Guo, Y. Zhao, Y. Zheng, Y. Qiao, J. Cai and S. Liu, Reactive effect of low intensity He-Ne laser upon damaged ultrastructure of human erythrocyte membrane in Fenton system by atomic force microscopy, Acta Biochim. Biophys. Sin., 2007, 39, 484–489 CrossRef.
- V. V. Moroz, A. K. Kirsanova, I. S. Novodergkina, V. V. Alexandrin, A. M. Chernysh and E. K. Kozlova, Macro- and microstructure of erythrocyte membranes under acute massive hemorrhage and subsequent blood reinfusion, Semin. Cardiothorac. Vasc. Anesth., 2010, 14, 248–255 CrossRef PubMed.
- M. N. Starodubtseva, T. G. Kuznetsova, S. A. Chizhik and N. I. Yegorenkov, Atomic force microscopy observation of peroxynitrite-induced erythrocyte cytoskeleton reorganization, Micron, 2007, 38, 782–786 CrossRef CAS PubMed.
- A. H. Swihart, J. M. Mikrut, J. B. Ketterson and R. C. Macdonald, Atomic force microscopy of the erythrocyte membrane skeleton, J. Microsc., 2001, 204, 212–225 CrossRef CAS.
- M. Takeuchi, H. Miyamoto, Y. Sako, H. Komizu and A. Kusumi, Structure of the erythrocyte membrane skeleton as observed by atomic force microscopy, Biophys. J., 1998, 74, 2171–2183 CrossRef CAS.
- M. P. Sheetz and S. J. Singer, Biological membranes as bilayer couples. A molecular mechanism of drug-erythrocyte interactions, Proc. Natl. Acad. Sci. U. S. A., 1974, 71, 4457–4461 CrossRef CAS.
- M. P. Sheetz and J. Dai, Modulation of membrane dynamics and cell motility by membrane tension, Trends Cell Biol., 1996, 6, 85–89 CrossRef CAS.
- E. U. Nwose, H. F. Jelinek, R. S. Richards and P. G. Kerr, Erythrocyte oxidative stress in clinical management of diabetes and its cardiovascular complications, Br. J. Biomed. Sci., 2007, 64, 35–43 CAS.
- R. T. Acton, J. C. Barton, L. V. Passmore, P. C. Adams, M. R. Speechley, F. W. Dawkins, P. Sholinsky, D. M. Reboussin, G. D. McLaren, E. L. Harris, T. C. Bent, T. M. Vogt and O. Castro, Relationships of serum ferritin, transferrin saturation, and HFE mutations and self-reported diabetes in the Hemochromatosis and Iron Overload Screening (HEIRS) study, Diabetes care, 2006, 29, 2084–2089 CrossRef CAS PubMed.
- E. S. Ford and M. E. Cogswell, Diabetes and serum ferritin concentration among U.S. adults, Diabetes care, 1999, 22, 1978–1983 CrossRef CAS.
- N. G. Forouhi, A. H. Harding, M. Allison, M. S. Sandhu, A. Welch, R. Luben, S. Bingham, K. T. Khaw and N. J. Wareham, Elevated serum ferritin levels predict new-onset type 2 diabetes: results from the EPIC-Norfolk prospective study, Diabetologia, 2007, 50, 949–956 CrossRef CAS PubMed.
- S. N. Rajpathak, J. P. Crandall, J. Wylie-Rosett, G. C. Kabat, T. E. Rohan and F. B. Hu, The role of iron in type 2 diabetes in humans, Biochim. Biophys. Acta, 2009, 1790, 671–681 CrossRef CAS PubMed.
- D. Galaris and K. Pantopoulos, Oxidative stress and iron homeostasis: mechanistic and health aspects, Crit. Rev. Clin. Lab. Sci., 2008, 45, 1–23 CrossRef CAS PubMed.
- K. Jomova and M. Valko, Importance of iron chelation in free radical-induced oxidative stress and human disease, Curr. Pharm. Des., 2011, 17, 3460–3473 CrossRef CAS.
- E. Lang, S. M. Qadri and F. Lang, Killing me softly - suicidal erythrocyte death, Int. J. Biochem. Cell Biol., 2012, 44, 1236–1243 CrossRef CAS PubMed.
- M. Singh and S. Shin, Changes in erythrocyte aggregation and deformability in diabetes mellitus: a brief review, Indian J. Exp. Biol., 2009, 47, 7–15 Search PubMed.
- M. Garnier, J. R. Attali, P. Valensi, E. Delatour-Hanss, F. Gaudey and D. Koutsouris, Erythrocyte deformability in diabetes and erythrocyte membrane lipid composition, Metab., Clin. Exp., 1990, 39, 794–798 CrossRef CAS.
- S. Labrouche, G. Freyburger, H. Gin, M. R. Boisseau and C. Cassagne, Changes in phospholipid composition of blood cell membranes (erythrocyte, platelet, and polymorphonuclear) in different types of diabetes–clinical and biological correlations, Metab., Clin. Exp., 1996, 45, 57–71 CrossRef CAS.
- M. C. Corti, M. Gaziano and C. H. Hennekens, Iron status and risk of cardiovascular disease, Ann. Epidemiol., 1997, 7, 62–68 CrossRef CAS.
- A. Dávalos, J. M. Fernandez-Real, W. Ricart, S. Soler, A. Molins, E. Planas and D. Genis, Iron-related damage in acute ischemic stroke, Stroke, 1994, 25, 1543–1546 CrossRef.
- A. K. Erdemoglu and S. Ozbakir, Serum ferritin levels and early prognosis of stroke, Eur. J. Neurol., 2002, 9, 633–637 CrossRef CAS.
- E. Millerot, A. S. Prigent-Tessier, N. M. Bertrand, P. J. Faure, C. M. Mossiat, M. E. Giroud, A. G. Beley and C. Marie, Serum ferritin in stroke: a marker of increased body iron stores or stroke severity?, J. Cereb. Blood Flow Metab., 2005, 25, 1386–1393 CrossRef CAS PubMed.
- A. D. van der, D. E. Grobbee, M. Roest, J. J. Marx, H. A. Voorbij and Y. T. van der Schouw, Serum ferritin is a risk factor for stroke in postmenopausal women, Stroke, 2005, 36, 1637–1641 CrossRef PubMed.
- K. H. Choi, M. S. Park, J. T. Kim, T. S. Nam, S. M. Choi, B. C. Kim, M. K. Kim and K. H. Cho, The serum ferritin level is an important predictor of hemorrhagic transformation in acute ischaemic stroke, Eur. J. Neurol., 2012, 19, 570–577 CrossRef PubMed.
- T. Reffelmann, T. Ittermann, M. Dorr, H. Volzke, M. Reinthaler, A. Petersmann and S. B. Felix, Low serum magnesium concentrations predict cardiovascular and all-cause mortality, Atherosclerosis, 2011, 219, 280–284 CrossRef CAS PubMed.
- W. R. Markesbery and J. M. Carney, Oxidative alterations in Alzheimer's disease, Brain Pathol., 1999, 9, 133–146 CrossRef CAS.
- P. I. Moreira, K. Honda, Q. Liu, M. S. Santos, C. R. Oliveira, G. Aliev, A. Nunomura, X. Zhu, M. A. Smith and G. Perry, Oxidative stress: the old enemy in Alzheimer's disease pathophysiology, Curr. Alzheimer Res., 2005, 2, 403–408 CrossRef CAS.
- A. Nunomura, G. Perry, G. Aliev, K. Hirai, A. Takeda, E. K. Balraj, P. K. Jones, H. Ghanbari, T. Wataya, S. Shimohama, S. Chiba, C. S. Atwood, R. B. Petersen and M. A. Smith, Oxidative damage is the earliest event in Alzheimer disease, J. Neuropathol. Exp. Neurol., 2001, 60, 759–767 CAS.
- J. C. Kovacic and V. Fuster, Atherosclerotic risk factors, vascular cognitive impairment, and Alzheimer disease, Mt. Sinai J. Med., 2012, 79, 664–673 CrossRef PubMed.
- M. A. Kling, J. Q. Trojanowski, D. A. Wolk, V. M. A. Lee and S. E. Arnold, Vascular disease and dementias: paradigm shifts to drive research in new directions, Alzheimer's Dementia, 2013, 9, 76–92 CrossRef PubMed.
- M. Diomedi and G. Misaggi, Vascular contribution to Alzheimer disease: predictors of rapid progression, CNS Neurol. Disord.: Drug Targets, 2013, 12, 532–537 CAS.
- M. T. Núñez, P. Urrutia, N. Mena, P. Aguirre, V. Tapia and J. Salazar, Iron toxicity in neurodegeneration, 2012, 25, 761–776 Search PubMed.
- W. Y. Ong and A. A. Farooqui, Iron, neuroinflammation, and Alzheimer's disease, J. Alzheimer's Dis., 2005, 8, 183–200 CAS , discussion 209–115.
- E. Adeghate, T. Donath and A. Adem, Alzheimer Disease and Diabetes Mellitus: Do They Have Anything In Common?, Curr. Alzheimer Res., 2013, 10, 609–617 CrossRef CAS.
- O. R. Aderibigbe, P. T. Pisa, R. L. Mamabolo, H.
S. Kruger and H. H. Vorster, The relationship between indices of iron status and selected anthropometric cardiovascular disease risk markers in an African population: the THUSA study, Cardiovasc. J. Afr., 2011, 22, 249–256 CrossRef CAS PubMed.
- P. A. Adlard and A. I. Bush, Metals and Alzheimer's disease, J. Alzheimer's Dis., 2006, 10, 145–163 Search PubMed.
- O. A. Azizova, A. G. Shvachko and A. V. Aseichev, Effect of iron ions on functional activity of thrombin, Bull. Exp. Biol. Med., 2009, 148, 776–779 CrossRef CAS.
- K. J. Barnham and A. I. Bush, Metals in Alzheimer's and Parkinson's diseases, Curr. Opin. Chem. Biol., 2008, 12, 222–228 CrossRef CAS PubMed.
- D. Robinson, J. Bucci, H. Fenn, T. Miller, J. Tinklenberg and J. A. Yesavage, Erythrocyte sedimentation rate in Alzheimer's dementia, J. Am. Geriatr. Soc., 1995, 43, 1177–1178 CAS.
- N. Tateishi, Y. Suzuki, I. Cicha and N. Maeda, O(2) release from erythrocytes flowing in a narrow O(2)-permeable tube: effects of erythrocyte aggregation, Am. J. Physiol.: Heart Circ. Physiol., 2001, 281, H448–H456 CAS.
- D. Tripathy, A. Sanchez, X. Yin, J. Luo, J. Martinez and P. Grammas, Thrombin, a mediator of cerebrovascular inflammation in AD and hypoxia, Front. Aging Neurosci., 2013, 5, 19 CAS.
- H. K. Eltzschig and P. Carmeliet, Hypoxia and inflammation, N. Engl. J. Med., 2011, 364, 656–665 CrossRef CAS PubMed.
- T. Wyss-Coray and J. Rogers, Inflammation in Alzheimer disease-a brief review of the basic science and clinical literature, Cold Spring Harbor Perspect. Med., 2012, 2, a006346 Search PubMed.
- J. G. Mohanty, H. D. Shukla, J. D. Williamson, L. J. Launer, S. Saxena and J. M. Rifkind, Alterations in the red blood cell membrane proteome in alzheimer's subjects reflect disease-related changes and provide insight into altered cell morphology, Proteome Sci., 2010, 8, 11 CrossRef PubMed.
- J. Choi, C. A. Malakowsky, J. M. Talent, C. C. Conrad and R. W. Gracy, Identification of oxidized plasma proteins in Alzheimer's disease, Biochem. Biophys. Res. Commun., 2002, 293, 1566–1570 CrossRef CAS.
- D. L. Merkle, C. H. Cheng, F. J. Castellino and B. A. Chibber, Modulation of fibrin assembly and polymerization by the beta-amyloid of Alzheimer's disease, Blood Coagulation Fibrinolysis, 1996, 7, 650–658 CrossRef CAS PubMed.
- J. Paul, S. Strickland and J. P. Melchor, Fibrin deposition accelerates neurovascular damage and neuroinflammation in mouse models of Alzheimer's disease, J. Exp. Med., 2007, 204, 1999–2008 CrossRef CAS PubMed.
- F. Goldblatt and S. G. O'Neill, Clinical aspects of autoimmune rheumatic diseases, Lancet, 2013, 382, 797–808 CrossRef CAS.
- B. Parker and I. Bruce, SLE and metabolic syndrome, Lupus, 2013, 22, 1259–1266 CrossRef CAS PubMed.
- N. K. Tyagi and R. G. Lahita, Therapeutic modalities in systemic lupus erythematosus, Saudi Med. J., 2013, 34, 887–895 Search PubMed.
- S. Vilaiyuk, N. Sirachainan, S. Wanitkun, K. Pirojsakul and J. Vaewpanich, Recurrent macrophage activation syndrome as the primary manifestation in systemic lupus erythematosus and the benefit of serial ferritin measurements: a case-based review, Clin. Rheumatol., 2013, 32, 899–904 CrossRef PubMed.
- S. Y. Lee, S. W. Lee and W. T. Chung, Severe inflammation may be caused by hyperferritinemia of pseudo-pseudo Meigs' syndrome in lupus patients: two cases reports and a literature review, Clin. Rheumatol., 2013, 32, 1823–1826 CrossRef PubMed.
- G. Zandman-Goddard, H. Orbach, N. Agmon-Levin, M. Boaz, H. Amital, Z. Szekanecz, G. Szucs, J. Rovensky, E. Kiss, N. Corocher, A. Doria, L. Stojanovich, F. Ingegnoli, P. L. Meroni, B. Rozman, J. Gomez-Arbesu, M. Blank and Y. Shoenfeld, Hyperferritinemia is associated with serologic antiphospholipid syndrome in SLE patients, Clin. Rev. Allergy Immunol., 2013, 44, 23–30 CrossRef CAS PubMed.
- W. Conca, M. Al-Hakim, N. Moussa, S. Al-Salam and P. Corr, Hyperferritinemia in a woman with systemic lupus erythematosus, severe nephritis and an iron-rich intraspinal schwannoma mimicking lupus myelopathy, Clin. Exp. Rheumatol., 2009, 27, 834–837 CAS.
- C. C. Liu, S. Manzi and J. M. Ahearn, Biomarkers for systemic lupus erythematosus: a review and perspective, Curr. Opin. Rheumatol., 2005, 17, 543–549 CrossRef CAS.
- G. Murphy, L. Lisnevskaia and D. Isenberg, Systemic lupus erythematosus and other autoimmune rheumatic diseases: challenges to treatment, Lancet, 2013, 382, 809–818 CrossRef CAS.
- M. I. Spengler, M. J. Svetaz, M. B. Leroux, S. M. Bertoluzzo, P. Carrara, F. Van Isseldyk, D. Petrelli, F. M. Parente and P. Bosch, Erythrocyte aggregation in patients with systemic lupus erythematosus, Clin. Hemorheol. Microcirc., 2011, 47, 279–285 CAS.
- B. Parker, A. Al-Husain, P. Pemberton, A. P. Yates, P. Ho, R. Gorodkin, L. S. Teh, M. Y. Alexander and I. N. Bruce, Suppression of inflammation reduces endothelial microparticles in active systemic lupus erythematosus, Ann. Rheum. Dis., 2013 Search PubMed , epub ahead of print.
- E. Ernst, A. Hein, M. Meurer and T. Ruzicka, Blood rheology in lupus erythematosus, Ann. Rheum. Dis., 1991, 50, 710–712 CrossRef CAS.
- M. J. Santos, L. M. Pedro, H. Canhao, E. F. J. Fernandes, J. Canas da Silva, J. E. Fonseca and C. Saldanha, Hemorheological parameters are related to subclinical atherosclerosis in systemic lupus erythematosus and rheumatoid arthritis patients, Atherosclerosis, 2011, 219, 821–826 CrossRef CAS PubMed.
- K. J. Allen, L. C. Gurrin, C. C. Constantine, N. J. Osborne, M. B. Delatycki, A. J. Nicoll, C. E. McLaren, M. Bahlo, A. E. Nisselle, C. D. Vulpe, G. J. Anderson, M. C. Southey, G. G. Giles, D. R. English, J. L. Hopper, J. K. Olynyk, L. W. Powell and D. M. Gertig, Iron-overload-related disease in HFE hereditary hemochromatosis, N. Engl. J. Med., 2008, 358, 221–230 CrossRef CAS PubMed.
- P. Clark, L. J. Britton and L. W. Powell, The diagnosis and management of hereditary haemochromatosis, Clin. Biochem. Rev., 2010, 31, 3–8 Search PubMed.
- L. C. Gurrin, N. J. Osborne, C. C. Constantine, C. E. McLaren, D. R. English, D. M. Gertig, M. B. Delatycki, M. C. Southey, J. L. Hopper, G. G. Giles, G. J. Anderson, J. K. Olynyk, L. W. Powell and K. J. Allen, The natural history of serum iron indices for HFE C282Y homozygosity associated with hereditary hemochromatosis, Gastroenterology, 2008, 135, 1945–1952 CrossRef CAS PubMed.
- V. R. Akoev, S. P. Shcherbinina, A. V. Matveev, S. Tarakhovskii Iu, A. A. Deev and V. L. Shnyrov, Study of structural transitions in erythrocyte membranes during hereditary hemochromatosis, Biull. Eksp. Biol. Med., 1997, 123, 279–284 CrossRef CAS.
- J. C. Barton, L. F. Bertoli and B. E. Rothenberg, Peripheral blood erythrocyte parameters in hemochromatosis: evidence for increased erythrocyte hemoglobin content, J. Lab. Clin. Med., 2000, 135, 96–104 CrossRef CAS.
- R. E. Fleming, R. S. Britton, A. Waheed, W. S. Sly and B. R. Bacon, Pathophysiology of hereditary hemochromatosis, Semin. Liver Dis., 2005, 25, 411–419 CrossRef CAS PubMed.
- D. Prá, S. I. Franke, J. A. Henriques and M. Fenech, Iron and genome stability: an update, Mutat. Res., 2012, 733, 92–99 CrossRef PubMed.
- F. Ezquer, M. T. Núñez, A. Rojas, J. Asenjo and Y. Israel, Hereditary hemochromatosis: an opportunity for gene therapy, Biol. Res., 2006, 39, 113–124 CAS.
- D. B. Kell, Finding novel pharmaceuticals in the systems biology era using multiple effective drug targets, phenotypic screening and knowledge of transporters: where drug discovery went wrong and how to fix it, FEBS J., 2013, 280(23), 5957–5980, DOI:10.1111/febs.12268.
- F. L. Li-Saw-Hee, A. D. Blann and G. Y. Lip, Effects of fixed low-dose warfarin, aspirin-warfarin combination therapy, and dose-adjusted warfarin on thrombogenesis in chronic atrial fibrillation, Stroke, 2000, 31, 828–833 CrossRef CAS.
- J. Vekasi, K. Koltai, V. Gaal, A. Toth, I. Juricskay and G. Kesmarky, The effect of aspirin on hemorheological parameters of patients with diabetic retinopathy, Clin. Hemorheol. Microcirc., 2008, 39, 385–389 CAS.
- B. Lipinski and E. Pretorius, The Role of Iron-Induced Fibrin in the Pathogenesis of Alzheimer's Disease and the Protective Role of Magnesium, Front. Hum. Neurosci., 2013, 7, 735 Search PubMed.
|
This journal is © The Royal Society of Chemistry 2014 |
Click here to see how this site uses Cookies. View our privacy policy here.