DOI:
10.1039/C2NJ40687J
(Paper)
New J. Chem., 2013,
37, 188-194
Lewis acidity and sugar receptor activity of 3-amino-substituted benzoxaboroles and their ortho-aminomethylphenylboronic acid analogues†
Received
(in Montpellier, France)
3rd August 2012
, Accepted 27th September 2012
First published on 1st October 2012
Abstract
The pKas as well as apparent binding constants of several 3-amino-substituted benzoxaboroles with alizarin red S (ARS) and some biologically important sugars have been evaluated and compared with that of the parent aminomethylphenylboronic acids. The investigated benzoxaboroles reveal lower acidity than the corresponding boronic acids. All the studied boronic compounds display the usual order of apparent binding constant values with glucose, galactose and fructose. The X-ray structure of the model catechol ester of the morpholinylmethylphenylboronic acid has been determined. The molecules are monomeric zwitterions with a tetracoordinated boron atom. The intermolecular interactions have been described with the aid of the Hirshfeld surface analysis.
Introduction
Recently, there have been many efforts to establish selective receptors of biologically important molecules. These endeavors resulted in the design of various active species, although some of them suffering from disadvantages like low sensitivity, selectivity or insufficient solubility in aqueous solutions. The other substantial issue with boronic sugar receptors is their diminished binding ability at neutral or acidic pH.1,2 Introduction of a coordinating group into the vicinity of the boronic acid group partially solved the problem. However, the designed receptors often suffered from low solubility in aqueous media.3 New hope arose with the application of a water soluble benzoxaborole molecule that was found to present high affinity towards glucose, fructose and glycopyranosides under physiologically relevant pH.4 The sugar binding activity of that simple and easily available compound was reported to be higher than that of the well established ortho-aminomethylphenylboronic acids (Wulff-type receptors). The benzoxaborole structural motif has been applied for medicinal purposes,5,6 such as inhibition of HIV entry,7–9 antiprotozoal drugs,10 antibacterial or antifungal agents11 as well as a potential drug delivery scaffold.12 The diversity of the investigated benzoxaborole structures is quite broad, yet it mostly covers the phenyl ring substituted derivatives.13–22
The first 3-amino-substituted benzoxaborole was reported several years ago23 and some of its physicochemical properties have been recently studied.24 Experimental studies enabled the synthesis of several 3-amino-substituted benzoxaboroles,25 which combine structural motifs of benzoxaboroles and ortho-aminomethylphenylboronic acids (Wulff-type receptors) within one molecule. Such a structure was expected to result in exceptional properties, which is the subject of the present work.
Results and discussion
The synthesis of the unsubstituted (1) and the amino-substituted benzoxaboroles (2a–c) as well as their aminomethyl analogues (3a–c) has been carried out according to the recently reported procedures.25–27 The investigated compounds enable comparison of the properties of 3-amino-substituted benzoxaboroles (2a–c) with their unsubstituted analogue (1) as well as the traditional ortho-aminomethylphenylboronic acids (3a–c) (Fig. 1).
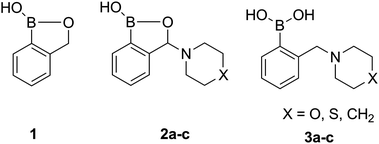 |
| Fig. 1 Unsubstituted benzoxaborole (1), 3-amino-substituted benzoxaboroles (2) and their aminomethylphenylboronic analogues (3). | |
Since the Lewis acidity of boronic acid receptors seems to play a crucial role in diol binding, as well as other biological actions,28,29 pKas of the investigated benzoxaboroles (1 and 2a–c) and their boronic acid analogues (3a–c) have been determined by a UV-Vis spectroscopy, according to the slightly modified method of Tomsho et al.30 The value obtained for 1 was consistent with the literature data.30 The measurements have been repeated three times with sufficient reproducibility of the results (see ESI†).
Benzoxaboroles usually display lower pKa (higher Lewis acidity) than the corresponding boronic acids. Owing to that, benzoxaboroles are prone to the tetragonal form in physiological solutions, which is crucial in the sensing of biomolecules. The pKas of various phenyl ring substituted benzoxaboroles were shown to follow the Hammett equation.30 Only one example of the compound with the substituted heterocyclic oxaborole ring has been reported so far (1a), displaying reasonably higher pKa than 1 (Fig. 2). Quite surprisingly, the pKas determined for the 3-amino-substituted benzoxaboroles (2a–c) are equal within the experimental error and almost the same as that for 1. Since the parent amines differ considerably in basicity, no difference in pKas of 2a–c means that the amino substituent is not involved in the acid–base equilibrium.
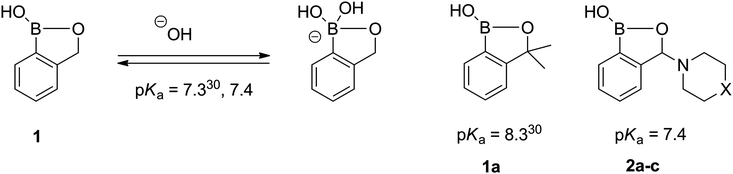 |
| Fig. 2 The acid–base equilibrium and pKa values of benzoxaboroles. | |
A different phenomenon can be observed in the case of the ortho-aminomethylphenylboronic acids (3), where amino groups interact with the boronic acid unit (Scheme 1), resulting in lowering the pKa of the boronic acid moiety. Due to the vicinity of the amino and boronic acid groups in 3 and sufficient flexibility of the amino group, formation of a N–B bond is possible, which considerably lowers the observed pKa29 as it was first described by S. L. Wiskur et al.31 At the same time, a single pKa value is observed instead of two as in the case of compounds with a distant boronic acid and an amino group like 3-pyridinium boronic acids investigated by S. Iwatsuki et al.32
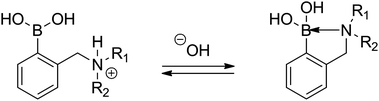 |
| Scheme 1 The acid–base equilibrium in ortho-aminomethylphenylboronic acids (3). | |
Despite a broad application of ortho-aminomethyl-substituted phenylboronic acids, only several pKa values of those compounds have been reported.28,29,31 Interestingly, in the case of the derivatives of aromatic amines (e.g.3f, Fig. 3), the pKas are considerably higher,29,33,34 due to the lower basicity of the aromatic amines.
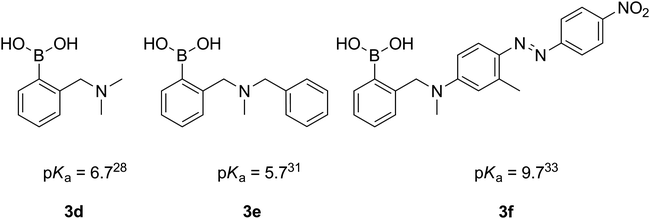 |
| Fig. 3 Reported pKa values of ortho-aminomethylphenylboronic acids. | |
The determined dissociation constants of 3a–c range from 4.8 to 6.3 (Fig. 4) and follow the pKas of the parent cyclic amines (8.39 for morpholine, 9.13 for thiomorpholine and 11.12 for piperidine), which confirms the expected N–B interaction and places those compounds amongst boronic acids of the lowest pKa.28
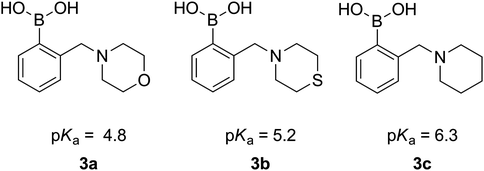 |
| Fig. 4 pKa values of 3a–3c. | |
Development of the competition binding assay with ARS by Springsteen and Wang35,36 enabled investigation of the sugar binding ability of non-fluorescent boronic acids with biologically important molecules, broadening the diagnostic potential of phenylboronic acid compounds. The boronic acid:ARS esters are also the basis of numerous indicator displacement assays, applied in sensing of various analytes.37–41
Since formation of the boronic acid–sugar complex in such systems takes place via transesterification, the values of the apparent binding constants of boronic acids with ARS may reasonably influence the evaluated apparent binding constants with sugars. Moreover, the determined values can give an idea of the trend in the binding of other aromatic 1,2-diols such as dopamine, noradrenaline or adrenaline (Fig. 5).
The values of the apparent binding constants of 1, 2a–c and 3a–c with ARS range from 659 for 1 to 3209 for 3c. No general tendency can be noticed within the determined values, yet both of the investigated piperidine derivatives 2c and 3c display relatively high binding constants (Fig. 6 and Table 1). 3-Thiomorpholinylbenzoxaborole (3b) displays surprisingly low KARS value, comparable with that determined for the unsubstituted benzoxaborole (1). Interestingly, its phenylboronic acid analogue (2b) displays relatively high KARS.
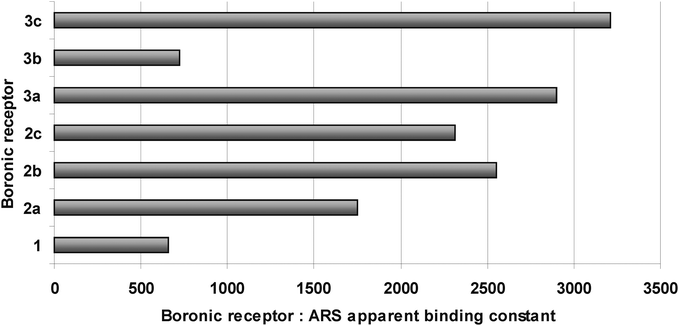 |
| Fig. 6 The apparent binding constants of 1, 2a–c and 3a–c with ARS, values for 3a and 3b have been reported previously.46 | |
|
1
|
2a
|
2b
|
2c
|
3a
a
|
3b
a
|
3c
|
Ref. 46.
|
K
ARS [M−1] |
659 |
1750 |
2556 |
2315 |
2900 |
720 |
3209 |
K
Fru [M−1] |
50 |
395 |
263 |
930 |
120 |
55 |
449 |
K
Gal [M−1] |
19 |
78 |
17 |
58 |
100 |
5 |
48 |
K
Glu [M−1] |
4 |
10 |
14 |
33 |
13 |
2 |
32 |
In order to get insight into the actual structure of the boronic acid:ARS complex, the model catechol ester was obtained by the esterification of 3a with 4. The reaction was carried out for 1 h at room temperature in a chloroform–methanol mixture (5
:
1, v/v) over Na2SO4 as a dehydrating agent (Scheme 2). The 1H NMR analysis of the resulting post-reaction mixture showed neither traces of the parent substances nor signal of the methoxy group. The monocrystal of compound 5a was obtained by slow evaporation of the solvents after removal of the drying agent.
Crystal structure of the obtained complex was determined by the X-ray diffraction technique. Similarly to Anslyn et al.42 findings, instead of a phenylboronic catechol ester with a three-coordinated boron center (5), a methoxylated boronate (5a) was detected in the crystalline state. Thus, the molecule of 5a can be described as a zwitterion with protonated amine and a deprotonated methanol molecule which binds to boron, and hence changing its coordination to sp3 (Fig. 7). Further, on the basis of some previous reports,42,43 the presence of such structure in the equilibrium solution containing methanol as a co-solvent should not be unexpected. The tetracoordinated boron center species should be less prone to transesterification with diols. As the competition assay relies upon transesterification of the boronic acid:ARS ester with sugars, the apparent binding constants of aminomethylphenylboronic acids with sugars can be reasonably underestimated if complexes similar to 5a are present in solution. The zwitterionic nature of the complexes may also have contributed to the low solubility of the complex in MeOH.43
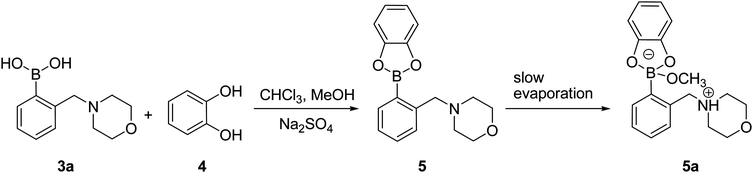 |
| Scheme 2 Synthesis of 5a. | |
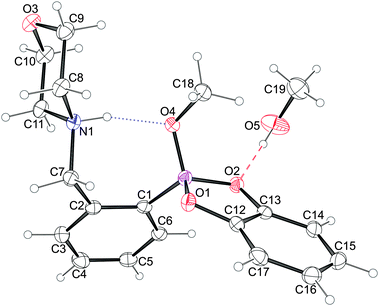 |
| Fig. 7 An Ortep-344 diagram of 5a showing the atoms’ thermal ellipsoids at 50% probability level together with their numbering scheme. The intramolecular N–H⋯O hydrogen bond is depicted with dotted lines while the O–H⋯O bond with the solvent molecule with dashed ones. | |
The molecules of 5a crystallize in a monoclinic system in P21/n space group symmetry with an additional methanol molecule. The solvent molecule is linked to the 5a molecule via a medium strong O–H⋯O hydrogen bond45 to one of the boronic acid oxygen atoms (Fig. 7). The morpholine ring shows the chair conformation with nitrogen and oxygen atoms lying on opposite sides of the ring. The similar conformation of the morpholine ring was observed in acid (3a)46 and benzoxaborole (2a)23 analogues. Moreover, the morpholine nitrogen atom serves as a donor to the methoxy oxygen atom, O4, forming the intramolecular hydrogen bond described with the graph S(7).47 The H-bond distances H⋯O, N⋯O are 1.75(2) and 2.625(1) Å, respectively, while the N–H⋯O angle equals 157(2)°, and these values are alike those observed for similar methoxylated boronic esters.42,43 It is worth noting that 5a is another example where the methoxy oxygen is a better hydrogen bond acceptor than the ring's oxygen atoms, which may be attributed to its higher Lewis basicity.
As all the strong hydrogen bond donors present in both the molecule in question and the solvent are engaged in either the intramolecular hydrogen bond or the H-bond denoted D according to Etter's notation,47 the only interactions that can lead to 3-D structure are the weak ones. The Hirshfeld surface analysis48 in the form of the decomposed fingerprint plots49 elucidated the presence of C–H⋯O as well as C–H⋯π intermolecular interactions only (Fig. 8). The C–H⋯O hydrogen bonds appear on the chart as long whiskers ending at the de + di ≈ 2.4 Å indicating the pronounced C–H⋯O bonds.50 The interactions with π-electrons show up as characteristic “wings”.49 The relatively short H⋯π contacts51 of 2.55 Å were found between the C(sp3)–H donor coming from morpholine, and the phenyl ring of the molecules related by 21 screw axes. All C–H⋯O hydrogen bonds found as well as the shortest C–H⋯π interaction join the 5a molecules into a layer on the (101) crystallographic plane. The substantially weaker C–H⋯π contacts combine the adjacent layers into 3-D structure (Fig. 3, ESI†).
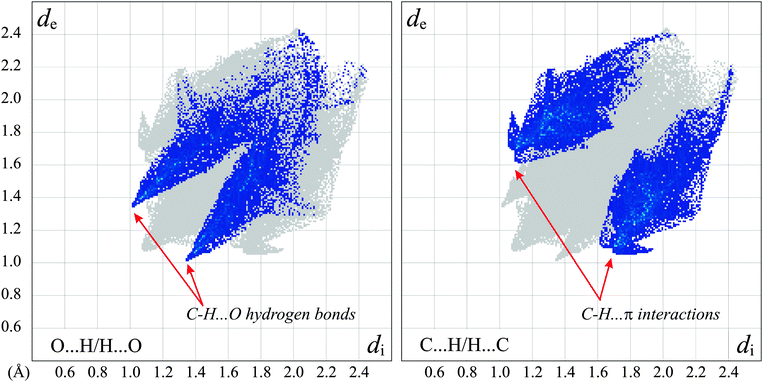 |
| Fig. 8 The 2-D representation of the Hirshfeld surface of the 5a molecule with H-bonded methanol. The decomposed fingerprint plots show the O⋯H/H⋯O (left) and C⋯H/H⋯C (right) contacts only with characteristic features for the prominent weak intermolecular interactions. The full fingerprint appears beneath each decomposed plot as a grey shadow. | |
The apparent binding constants of 1, the 3-amino-substituted benzoxaboroles (2a–c) and piperidinylmethylphenylboronic acid (3c) with glucose, galactose and fructose have been quantitatively evaluated using the Springsteen and Wang method.36 The determined K values have been compared with the previously reported binding constants of 3a and 3b.45 As differences in solution composition may strongly influence the binding constant values, all the measurements have been carried out under the same conditions (methanol–0.1 M aqueous phosphate buffer pH = 7.4 (1
:
1, v/v) + 1 vol% DMSO), close to the physiological ones. All the determined apparent binding constants together with KARS are given in Table 1.
The introduction of the amino substituent into the heterocyclic benzoxaborole ring influences sugar binding reasonably, resulting generally in the increase in the observed K. Binding of 2b with galactose is an exception, with a small drop in the apparent KGal value in comparison with that for 1. All the considered boronic acid molecules bind fructose preferably (the highest K values), which is a common case for most of the mono-phenylboronic acids. The KFru values are reasonably higher for the 3-amino-substituted benzoxaboroles (2a–c) in comparison with those of their boronic acid analogues (3a–c). No such general conclusion can be drawn for KGal or KGlu. Interestingly, both piperidine derivatives 2c and 3c display reasonably high KGlu of 33 and 32 respectively.
Conclusions
The acidity and sugar receptor activity of 3-amino-substituted benzoxaboroles were determined for the first time. The seemingly analogously arranged amino and boronic groups in 3-amino-substituted benzoxaboroles (2a–c) and their ortho-aminomethylphenylboronic analogues (3a–c) display totally different dissociation behaviour. The lack of flexibility of the amino group in 2 precludes any N–B interaction, resulting in considerably higher pKa. Interestingly, the introduction of the amino group into the heterocyclic benzoxaborole ring does not influence the pKa. Consequently, the investigated benzoxaboroles (1 and 2a–c) reveal considerably lower Lewis acidity than the corresponding phenylboronic acids (3a–c). The model catechol ester (5a) forms a zwitterionic structure with a tetracoordinated boron center in the solid state. Its formation confirms high Lewis acidity of the catechol ester (5). All the investigated receptors (1, 2 and 3) follow the usual order of apparent binding constants for monoboronic acids and glucose, galactose and fructose (KGlu < KGal < KFru). None of the determined apparent binding constants correlates with pKa of the investigated boronic acid compounds.
Experimental
Chemicals
Saccharides were purchased from Fluka assay >98.0% (HPLC, sum of enantiomers). The investigated compounds have been obtained according to the previously reported methodologies 1,272a–c25 and 3a–c.27 The identity and purity of the samples were confirmed on the basis of the elemental analysis: 1 (found: C, 62.7; H, 5.2. Calc. for C7H7BO2: C, 62.8; H, 5.3%), 2a (found: C, 60.2; H, 6.4; N 6.35. Calc. for C11H14BNO3: C, 60.2; H, 6.3; N, 6.4%), 2b (found: C, 56.3; H, 5.9; N, 5.9. Calc. for C11H14BNO2S: C, 56.2; H, 6.0; N, 6.0%), 2c (found: C, 66.4; H, 7.5, N, 6.5. Calc. for C12H16BNO2: C, 66.4, H, 7.3, N, 6.45%), 3a (found: C, 59.7; H, 7.4; N, 6.3. Calc. for C11H16BNO3: C, 59.8; H: 7.2, N: 6.2%), 3b (found: C, 56.0; H, 6.0; N, 5.8. Calc. for C11H16BNO2S: C, 55.6; H, 6.7; N, 5.8), 3c (found: C, 65.7; H, 8.1; N, 6.2. Calc. for C12H18BNO2: C, 65.8; H, 8.3; N, 6.4%). The 1H NMR spectra have been consistent with the standard samples.
Synthesis of 5
Chloroform (50 mL), methanol (10 mL), morpholinylmethyl phenylboronic acid (3a) (0.771 g, 3.5 × 10−3 mol) and catechol (4) (0.385 g, 3.5 × 10−3 mol) were placed in a 250 mL round bottomed flask. Anhydrous Na2SO4 (1.5 g) was added and left for 1 h at room temperature. The drying agent was filtered off and clear solution left to slowly evaporate. After several hours, colorless crystals of the tetracoordinated methoxy salt of the ester appeared. The volatiles have been evaporated at reduced pressure resulting in the formation of a white powder (1.001 g, 97%). 1H NMR (CDCl3, 400 MHz), δ [ppm]: 7.57–7.52 (m, 1H, Ar); 7.35–7.29 (m, 2H, Ar); 7.17–7.15 (m, 1H, Ar); 6.90–6.86 (m, 2H, Ar); 6.82–6.78 (m, 2H, Ar); 4.24 (s, 2H, CH2); 3.75 (br, 4H, 2 × CH2); 3.26 (br, 2H, CH2); 2.82 (br, 2H, CH2).
Determination of pKa
The analyte compounds were dissolved in a 0.1 M phosphate buffer to the final concentration of 1.0 × 10−3 M. The pH of the solution was adjusted with sodium hydroxide. The pH measurements were performed using a Mettler Toledo pH meter. Spectrophotometric measurements for the determination of the acid dissociation constants were carried out using an Analytik Jena SPECORD S600 spectrophotometer. Solutions having the proper pH were placed in quartz cuvettes, and UV-Vis absorption scans were performed. The spectra were analyzed by calculating the spectral difference between the acid spectra and the spectra obtained at other pH. The wavelengths of maximum positive and negative deviations were determined graphically, and the absolute values of the absorbance difference at these wavelengths were summed. The total absorbance difference was then plotted vs. pH, and the data was fit to the Henderson–Hasselbalch equation52 to obtain the pKa value.
Determination of the apparent binding constants with ARS and sugars
A Varian Cary Eclipse fluorometer was used for the fluorescence studies. All compounds have been dissolved in the mixture: methanol–0.1 M aqueous phosphate buffer pH = 7.400 (1
:
1, v/v) + 1 vol% DMSO. Fluorescence spectra were collected for a solution with constant concentration of ARS (10−4 M) and proper boronic receptor. All the spectra have been smoothed using Savitzky–Golay function.53 The maximum fluorescence intensity was then read from the spectra. The association constant of the ARS–boronic acid (KARS) was calculated using the modified Benesi–Hildebrand equation.36 Then a 1.0 × 10−4 M solution of ARS with an appropriate boronic acid concentration was prepared, keeping ca. 20% of ARS in its free form. The solution was transferred into a quartz cuvette where fluorometric titration was performed.
X-ray studies
Single crystals of 5a suitable for X-ray measurements were crystallized from methanol. The data was collected at 100 K on a Gemini A Ultra diffractometer (Agilent Technologies) using mirror monochromated Cu-Kα radiation (λ = 1.54184 Å). The CrysAlisPro program54 was used for data collection, cell refinement, data reduction and the empirical absorption corrections using spherical harmonics, implemented in the multi-scan scaling algorithm. Using Olex2 suite,55 the structure was solved with the ShelXS program56 using direct methods and refined with the ShelXL refinement package56 using least-squares minimisation. All non-hydrogen atoms were refined with anisotropic temperature factors. The positions of hydrogen atoms bonded to oxygen and nitrogen atoms were refined freely with a fixed isotropic displacement parameter equal to 1.5 and 1.2 × Ueq of the parent atom, respectively. The remaining hydrogen atoms were refined with a fixed geometry, riding on their carrier atoms, with a fixed isotropic displacement parameter. The decomposed 2-D fingerprint plots presented in this paper in Fig. 8 were generated using Crystal Explorer 3.0 program.57
Crystal data: C19H26BNO5, M = 359.22, monoclinic, a = 13.1083(3) Å, b = 9.45522(19) Å, c = 15.1358(4) Å, β = 99.016(2)°, V = 1852.80(7) Å3, T = 100.15 K, space group P21/n (no. 14), Z = 4, μ(Cu Kα) = 0.747, 23
639 reflections measured, 3316 unique (Rint = 0.0390) which were used in all calculations. The final wR2 was 0.0894 (all data) and R1 was 0.0335 (I > 2σ(I)).
Acknowledgements
This work has been supported by the European Union in the framework of the European Social Fund through the Warsaw University of Technology Development Programme by the scholarship awarded by the Center of Advanced Studies for Agnieszka Adamczyk-Woźniak and Kamil Żukowski. K.M.B., I.D.M. and E.T. acknowledge financial support from Warsaw University of Technology.
References
-
T. D. James, M. D. Phillips and S. Shinkai, Boronic Acids in Saccharide Recognition, RSC Publishing, Cambridge, 2006 Search PubMed.
-
(a)
T. D. James, in Boronic acids. Preparation, applications in organic synthesis and medicine, ed. D. G. Hall, Wiley-VCH, Weinheim, Germany, 2006, ch. 12, pp. 441–474 Search PubMed;
(b)
N. Ni and B. Wang, in Boronic acids. Preparation and applications in organic synthesis, medicine and materials, ed. D. G. Hall, Wiley-VCH, Weinheim, Germany, 2nd edn, 2011, ch. 13, vol. 2, pp. 591–617 Search PubMed.
- H. Li, H. Wang, Y. Liu and Z. Liu, Chem. Commun., 2012, 48, 4115 RSC.
- M. Bérubé, M. Dowlut and D. G. Hall, J. Org. Chem., 2008, 73, 6471 CrossRef CAS.
- A. Adamczyk-Woźniak, M. K. Cyrański, A. Żubrowska and A. Sporzyński, J. Organomet. Chem., 2009, 694, 3533 CrossRef CAS.
- V. M. Dembitsky, A. A. A. A. Quntar and M. Srebnik, Chem. Rev., 2011, 111, 209 CrossRef CAS.
- J. I. Jay, B. E. Lai, D. G. Myszka, A. Mahalingam, K. Langheinrich, D. F. Katz and P. F. Kiser, Mol. Pharmaceutics, 2010, 7, 116 CrossRef CAS.
- A. Mahalingam, A. R. Geonnotti, J. Balzarini and P. F. Kiser, Mol. Pharmaceutics, 2011, 8, 2465 Search PubMed.
- J. N. Cambre and B. S. Sumerlin, Polymer, 2011, 52, 4631 CrossRef CAS.
- R. T. Jacobs, J. J. Plattner and M. Keenan, Curr. Opin. Infect. Dis., 2011, 24, 586 Search PubMed.
- A. Adamczyk-Woźniak, O. Komarovska-Porokhnyavets, B. Misterkiewicz, V. P. Novikov and A. Sporzyński, Appl. Organomet. Chem., 2012, 26, 390 Search PubMed.
- G. A. Ellis, M. J. Palte and R. T. Raines, J. Am. Chem. Soc., 2012, 134, 3631 Search PubMed.
- A. Ricardo, F. Frye, M. A. Carrigan, J. D. Tipton, D. H. Powell and S. A. Benner, J. Org. Chem., 2006, 71, 9503 Search PubMed.
- S. Schumacher, M. Katterle, C. Hettrich, B. R. Paulke, A. Pal, D. G. Hall, F. W. Scheller and N. Gajovic-Eichelmann, Chem. Sens., 2011, 1, 1 Search PubMed.
- S. Schumacher, F. Grüneberger, M. Katterle, C. Hettrich, D. G. Hall, F. W. Scheller and N. Gajovic-Eichelmann, Polymer, 2011, 52, 2485 Search PubMed.
- L. Ye, D. Ding, Y. Feng, D. Xie, P. Wu, H. Guo, Q. Meng and H. Zhou, Tetrahedron, 2009, 65, 8738 Search PubMed.
- Y. Xia, K. Cao, Y. Zhou, M. R. K. Alley, F. Rock, M. Mohan, M. Meewan, S. J. Baker, S. Lux, C. Z. Ding, G. Jia, M. Kully and J. J. Plattner, Bioorg. Med. Chem. Lett., 2011, 21, 2533 Search PubMed.
- X. Li, S. Zhang, Y.-K. Zhang, Y. Liu, C. Z. Ding, Y. Zhou, J. J. Plattner, S. J. Baker, W. Bu, L. Liu, W. M. Kazmierski, M. Duan, R. M. Grimes, L. L. Wright, G. K. Smith, R. L. Jarvest, J.-J. Ji, J. P. Cooper, M. D. Tallant, R. M. Crosby, K. Creech, Z.-J. Ni, W. Zou and J. Wright, Bioorg. Med. Chem. Lett., 2011, 21, 2048 Search PubMed.
- Y.-K. Zhang, J. J. Plattner, T. Akama, S. J. Baker, V. S. Hernandez, V. Sanders, Y. Freund, R. Kimura, W. Bu, K. M. Hold and X.-S. Lu, Bioorg. Med. Chem. Lett., 2010, 20, 2270 Search PubMed.
- Y.-K. Zhang, J. J. Plattner, E. E. Easom, D. Waterson, M. Ge, Z. Li, L. Li and Y. Jian, Tetrahedron Lett., 2011, 52, 3909 Search PubMed.
- Y.-K. Zhang, J. J. Plattner, Y. R. Freund, E. E. Easom, Y. Zhou, J. Gut, P. J. Rosenthal, D. Waterson, F.-J. Gamo, I. Angulo-Barturen, M. Ge, Z. Li, L. Li, Y. Jian, H. Cui, H. Wang and J. Yang, Bioorg. Med. Chem. Lett., 2011, 21, 644 Search PubMed.
- A. Pal, M. Bérubé and D. G. Hall, Angew. Chem., Int. Ed., 2010, 49, 1492 CAS.
- A. Sporzyński, M. Lewandowski, P. Rogowska and M. K. Cyrański, Appl. Organomet. Chem., 2005, 19, 1202 CrossRef CAS.
- A. Jezierska, J. J. Panek, G. Z. Żukowska and A. Sporzyński, J. Phys. Org. Chem., 2010, 23, 451 Search PubMed.
- A. Adamczyk-Woźniak, I. Madura, A. H. Velders and A. Sporzyński, Tetrahedron Lett., 2010, 51, 6181 Search PubMed.
- A. Adamczyk-Woźniak, M. K. Cyrański, M. Jakubczyk, P. Klimentowska, A. Koll, J. Kołodziejczak, G. Pojmaj, A. Żubrowska, Z. Żukowska and A. Sporzyński, J. Phys. Chem. A, 2010, 114, 2324 Search PubMed.
- A. Adamczyk-Woźniak, I. Madura, A. Pawełko, A. Sporzyński, A. Żubrowska and J. Żyła, Cent. Eur. J. Chem., 2011, 9, 199 Search PubMed.
- J. Yan, G. Springsteen, S. Deeter and B. Wang, Tetrahedron, 2004, 60, 11205 CAS.
- X. Zhang, L. Chi, S. Ji, Y. Wu, P. Song, K. Han, H. Guo, T. D. James and J. Zhao, J. Am. Chem. Soc., 2009, 131, 17452 CrossRef CAS.
- J. W. Tomsho, A. Pal, D. G. Hall and S. J. Benkovic, ACS Med. Chem. Lett., 2012, 3, 48 Search PubMed.
- S. L. Wiskur, J. J. Lavigne, H. Ait-Haddou, V. Lynch, Y. H. Chiu, J. W. Canary and E. V. Anslyn, Org. Lett., 2001, 3, 1311 CrossRef CAS.
- S. Iwatsuki, Y. Kanamitsu, H. Ohara, E. Watanabe and K. Ishihara, J. Phys. Org. Chem., 2012, 25, 760 Search PubMed.
- C. J. Ward, P. Patel and T. D. James, J. Chem. Soc., Perkin Trans. 1, 2002, 462 RSC.
- K. R. A. S. Sandanayake and S. Shinkai, J. Chem. Soc., Chem. Commun., 1994, 1083 RSC.
- G. Springsteen and B. Wang, Chem. Commun., 2001, 1608 RSC.
- G. Springsteen and B. Wang, Tetrahedron, 2002, 58, 5291 CrossRef CAS.
- S. Zhang and T. E. Glass, Tetrahedron Lett., 2010, 51, 112 CrossRef CAS.
- K. Ngamdee, T. Noipa, S. Martwiset, T. Tuntulani and W. Ngeontae, Sens. Actuators, B, 2011, 160, 129 Search PubMed.
- A. J. Cross, M. G. Davidson, D. García-Vivó and T. D. James, RSC Adv., 2012, 2, 5954 RSC.
- L. Gu, Y. Liang, T. Zhou, X. Tanga and G. Shi, Anal. Methods, 2012, 4, 492 RSC.
- J. W. Tomsho and S. J. Benkovic, J. Org. Chem., 2012, 77, 2098 Search PubMed.
-
(a) L. Zhu, S. H. Shabbir, M. Gray, V. M. Lynch, S. Sorey and E. V. Anslyn, J. Am. Chem. Soc., 2006, 128, 1222 CrossRef CAS;
(b) B. E. Collins, S. Sorey, A. E. Hargrove, S. H. Shabbir, V. M. Lynch and E. V. Anslyn, J. Org. Chem., 2009, 74, 4055 CrossRef CAS.
- L. Zhang, J. A. Kerszulis, R. J. Clark, T. Ye and L. Zhu, Chem. Commun., 2009, 2151 RSC.
- L. J. Farrugia, J. Appl. Crystallogr., 1999, 32, 837 CrossRef.
- P. Gilli, V. Bertolasi, V. Ferretti and G. Gilli, J. Am. Chem. Soc., 1994, 116, 909 CrossRef CAS.
- A. Adamczyk-Woźniak, Z. Brzózka, M. K. Cyrański, A. Filipowicz-Szymańska, P. Klimentowska, A. Żubrowska, K. Żukowski and A. Sporzyński, Appl. Organomet. Chem., 2008, 22, 427 Search PubMed.
- M. C. Etter, Acc. Chem. Res., 1990, 23, 120 CrossRef CAS.
- M. A. Spackman and P. G. Byrom, Chem. Phys. Lett., 1997, 267, 215 CrossRef CAS.
- J. J. McKinnon, D. Jayatilaka and M. A. Spackman, Chem. Commun., 2007, 3814 RSC.
- T. Steiner, Angew. Chem., Int. Ed., 2002, 41, 48 CrossRef CAS.
- M. Nishio, Phys. Chem. Chem. Phys., 2011, 13, 13873 RSC.
- S. Soundararajan, M. Badawi, C. M. Kohlrust and J. H. Hageman, Anal. Biochem., 1989, 178, 125 CAS.
- A. Savitzky and M. J. E. Golay, Anal. Chem., 1964, 36, 1627 CrossRef CAS.
- CrysAlisPro, Agilent Technologies, Version 1.171.35.7 (release 14-02-2011 CrysAlis171 .NET).
- O. V. Dolomanov, L. J. Bourhis, R. J. Gildea, J. A. K. Howard and H. Puschmann, J. Appl. Crystallogr., 2009, 42, 339 CrossRef CAS.
- G. M. Sheldrick, Acta Crystallogr., Sect. A: Found. Crystallogr., 2008, 64, 112.
-
S. K. Wolff, D. J. Grimwood, J. J. McKinnon, M. J. Turner, D. Jayatilaka and M. A. Spackman, CrystalExplorer (Version 3.0), University of Western Australia, 2012 Search PubMed.
Footnote |
† Electronic supplementary information (ESI) available: pKa values determined in three independent experiments, UV/vis spectral scans for compounds 1, 3c and phenylboronic acid in solutions at various pH values; normalized spectral scans, spectral difference between different solutions of compounds, pKa determination plots, the apparent binding constant values (K [M−1]) of 1, 2a–c and 3a–c with ARS, fructose, galactose and glucose; fluorescence spectrum of ARS at different concentrations of boronic acid; 1/ΔIF versus 1/Cboronic acid plot; selected geometrical parameters and packing diagram for 5a. Geometry of intra- and intermolecular interactions in 5a. CCDC 893518 (5a). For ESI and crystallographic data in CIF or other electronic format see DOI: 10.1039/c2nj40687j |
|
This journal is © The Royal Society of Chemistry and the Centre National de la Recherche Scientifique 2013 |