Modulation of gene expression by the oxidative stress generated in human skin cells by UVA radiation and the restoration of redox homeostasis†
Received
14th July 2011
, Accepted 15th September 2011
First published on 19th October 2011
Abstract
UVA radiation generates a significant oxidative stress in skin cells which is further enhanced by the release of the pro-oxidant catalysts iron and heme, and exacerbated by UVA-mediated destruction of cellular reducing equivalents and the antioxidant enzyme catalase. An important consequence of this altered redox state is the generation of oxidized membrane components in the form of 4-hydroxynonenal, ceramides and oxidized phospholipids, all of which are potent signalling molecules which lead to modulation of the expression of many genes. Transcription factors (such as nuclear factor kappa-light-chain-enhancer of activated B cells) and several genes (e.g. interleukins, intercellular adhesion molecule and 1, hemeoxygenase 1) involved in the inflammatory response are dramatically modified by UVA. Levels of both antioxidant and pro-oxidant proteins, including manganese-dependent superoxide dismutase, glutathione peroxidase, hemeoxygenase 1, NADPH oxidase, ferritin, and methionine-S-sulfoxidereductase, are increased by UVA treatment and following moderate dose levels these will contribute to either the restoration or a further perturbation of redox homeostasis. Finally, UVA induces a whole set of matrix metalloproteinases and proteases, primarily in cells of dermal origin, which can contribute to the long-term consequences of UVA exposure of skin.
1 Introduction
A key and essential property of all cells, tissues and organisms is their ability to maintain a state of homeostasis. All cellular and multicellular organisms have evolved stress responses to restore homeostasis as rapidly as possible under adverse physical and chemical conditions. Eucaryotic cells were shown to respond to short wavelength UV (UVC) radiation by inducing a plethora of genes in a phenomenon known as the “UV response”, and the genes activated included oncogenes such as c-FOS and c-JUN as well as interstitial collagenase (matrix metalloproteinase 1, MMP-1). Activation of several types of transcription factor were central to the response (e.g., Activator protein 1 (AP-1), nuclear factor kappa-light-chain-enhancer of activated B cells (NFκB) and p53) and key upstream events included the activation of several types of mitogen-activated protein kinases (MAPKs). Numerous publications on this topic have appeared over the years but it should be noted that UV doses were often very high and, while similar results were obtained with UVB radiation and the results have been very informative in terms of understanding the cellular signalling response, the doses were frequently out of any reasonable physiological range.
In contrast to the UVB wavelength range, the UVA component of sunlight generates a major oxidative stress in cells at physiologically relevant doses. One of the early events appears to be generation of singlet oxygen. Events that rapidly follow include oxidation of lipids and phospholipids and products include 4-hydroxynonenal (4-HNE) and ceramides which can act as signalling molecules. The latter are believed to be key intermediates in the stimulation of nicotinamide adenine dinucleotide phosphate oxidase 1 (NOX-1) which, at least in keratinocytes, leads to a rapid further increase in reactive oxygen species (ROS), particularly superoxide anion. UVA-mediated oxidative events are further exacerbated by both the immediate and the delayed release of the pro-catalytic factors, free iron and heme, by UVA. As a consequence of these events, a key property of UVA radiation is the perturbation of redox homeostasis in cells and the consequent increase in lipid signalling intermediates leads to activation of various genes. The purpose of this review is to describe these complex consequences of UVA irradiation and to point out, where relevant, how such induced events contribute to restoration of redox homeostasis. The studies described have been undertaken using human skin fibroblasts unless stated otherwise.
2
UVA as an oxidative stress
a Evidence in vitro and in cells
It was observed a century ago that glass-filtered radiation (i.e. >302 nm) emitted from a mercury source inactivated enzymes such as amylase, catalase, trypsin and tyrosinase and that this damage was oxygen-dependent (H. Agulhon, Ann. Inst. Pasteur Paris, 1912, 26, 38–47). Many years later it was shown that the UVA inactivation of Escherichia coli was oxygen dependent,1 and studies followed in several microorganisms and in human cells where almost complete oxygen-dependence of inactivation of colony forming ability was shown.2,3 Work from several laboratories using components of cellsin vitro demonstrated that UVA radiation at relevant doses had the potential to generate various ROS in cells and this, together with evidence for the oxygen-dependence of most UVA-mediated biological events, provided the basis for concluding that UVA would generate a strong oxidative stress in cells and tissue (see Fig. 1). Experiments with human fibroblasts demonstrated that reduction in the major source of cellular reducing equivalents (reduced glutathione, GSH) dramatically enhanced UVA-mediated events such as cell death4 and gene activation,5 further implicating ROS in these events.
UVA leads to an extremely rapid depletion of cellular GSH, the major source of cellular reducing equivalents, in both human fibroblasts5 as well as human skin.2 This occurs at routine solar exposure levels and will exacerbate oxidative stress levels in cells. GSH depletion also occurs after UVB but the depletion as a function of other biological events (e.g.death) is several times less than UVA. Catalase itself is exquisitely sensitive to UVA radiation because of its heme-containing groups and the solar sensitivity of catalase is well-demonstrated by the seasonal variation of its activity in the stratum corneum of skin where it is much lower in the summer months.6 Additional studies with broad band UVA and UVB lamps showed that the effect was due to the UVA component. In contrast, superoxide dismutase levels were not affected by seasonal variation.
b Evidence in tissue
ROS have been measured in the skin of hairless rats7 and in the stratum corneum of human skin after UVA8,9 with some evidence of ROS generation in deeper layers. These studies of the UVA radiation-induced chemiluminescence of human skin in vivo have been complemented10 by following the decay characteristics of UVA-induced photon emission caused by different radiation doses. This approach provides an extremely sensitive methodology to measure the effectiveness of topically applied antioxidants. There is a considerable literature showing that levels of skin antioxidants and antioxidant enzymes are not only reduced by acute UVA exposures but also decrease with photoaging which again will exacerbate the oxidative stress generated by further exposure to the oxidising components of sunlight11 and should be measurable by these new methodologies.
c Specific ROS generated
Several lines of evidence indicate that the main ROS species generated in cells and tissue by UVA radiation is singlet oxygen and that this species is the main one involved in UVA effects from cell death to gene activation.12,13 Although the latter was first demonstrated with the hemeoxygenase 1 (HO-1) gene, studies with other UVA inducible genes reported a similar dependence.14 Many subsequent studies demonstrated the role of this species in UVA-mediated signalling15 so that UVA-generated singlet oxygen is now generally accepted as a key early intermediate in cellular signalling pathways.
Recently it has been shown that UVA (presumably via singlet oxygen) enhances NADPH oxidase 1 (NOX-1) and that this is a primary source of UVA-induced ROS generation (primarily superoxide anion) in immortalized human skin keratinocytes at sub-lethal doses.16 In contrast, a study17 comparing normal primary murine keratinocytes with cells knocked out for phagocyte oxidase, demonstrated that this oxidase played only a limited role in both the induction of ROS and the increased apoptosis that follows UVA irradiation of these cells.
d Disruption of iron and heme homeostasis
In addition to the production of ROS, UVA radiation can influence the levels of pro-oxidant catalysts, particularly iron and heme.18,19Iron plays a catalytic role in Fenton chemistry which results in peroxide generation in cells and, although iron is not involved in the initial step of lipid peroxidation, it can play a catalytic role in the lipid peroxidation chain reaction. Reflecting these observations, there have been numerous examples where modulating free cellular iron levels by iron chelation has led to modulation of UVA-mediated effects.19 Importantly, it has been shown20 that low dose irradiation of human skin fibroblasts with UVA can increase the levels of labile iron in these cells several-fold. This appears to result from UVA damage to the lysosomal membranes. Subsequent studies have confirmed these findings and shown that free iron is also increased in other skin cell types following UVA21 and further implicated iron in necrotic cell death.22 The role of iron in UVA and other oxidant-mediated effects is explored in depth in the article by Aroun et al. in this issue.151
Free heme can also play a catalytic role in the appropriate cellular environment and is a major UVA absorbing chromophore. UVA can damage heme-containing proteins (e.g.catalase, see above) and, more recently, it has been shown that following UVA damage to cells free heme is released.23 The release of both labile iron and free heme by UVA radiation will further amplify the oxidative stress engendered by direct production of ROS.
3
UVA as an oxidant that modulates gene expression
a Introduction
UVA is well-known to activate expression of a variety of genes at doses relevant to natural sunlight exposure (see Fig. 2).24,25 Of these, the largest and most studied effects are the activation of HO-126,27 and a series of metalloproteinases as first observed for (MMP-1).28 However many other genes have been observed to be activated by UVA including intercellular adhesion molecule 1 (ICAM-1)29 and cyclooxygenase 2 (COX-2).30 Much has been learnt recently about the UVA-induced signalling intermediates and the complex cascades of signalling kinases and phosphatases involved in these effects. The pathways are often cell-type dependent.
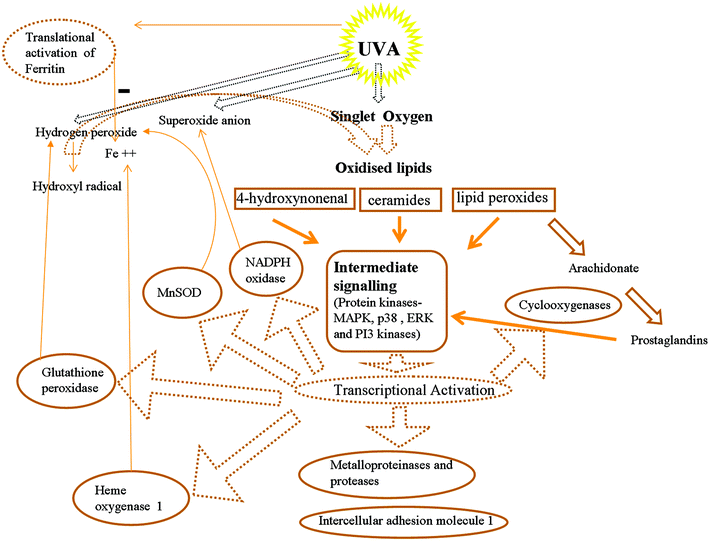 |
| Fig. 2 Generation of oxidised signalling lipids and transcriptional activation as a consequence of UVA irradiation of cells. Reactive oxygen species and free iron may be increased (+) or decreased (−) as a consequence of activation of expression of specific genes with a profound influence on redox homeostasis. | |
b UVA-generated signalling intermediates
i Singlet oxygen.
Pioneering studies31 had shown that ROS, particularly peroxide, were involved in modulation of gene expression and there is now a substantial literature on redox regulation of genesvia a variety of mechanisms. Studies on UVA inactivation of human cells had shown that singlet oxygen was involved in this response, and the demonstration that this intermediate was the primary ROS intermediate in UVA activation of HO-1 gene expression was the first demonstration that singlet oxygen was potentially a key signalling intermediate.13 Studies soon followed that showed, not surprisingly, that activation of gene expression in photodynamic therapy models (that involved photosensitisers that generated singlet oxygen) also arose via this intermediate. Singlet oxygen was also shown to be involved in UVA activation of MMP-132 and ICAM-1,33 as well as most UVA-mediated signalling events subsequently tested. The UVA generation of singlet oxygen is now considered a primary early event in the gene modulation process and is likely to act via the generation of oxidised lipids such as ceramides, 4-HNE and oxidised phospholipid, all of which are powerful signalling molecules (Fig. 2).
ii
Lipid oxidation products, oxidised phospholipids and 4-HNE.
Although ceramide can be generated enzymatically, UVA is believed to act photochemically on sphingomyelin to generate a ceramide intermediate,34 although there is some controversy in the literature on this issue.35Ceramide is a key component in cellular stress responses and has been shown to be implicated in the AP-2 dependent activation of ICAM-1 expression in keratinocytes.33 The process is likely to involve a ceramide-mediated autocrine loop since the second phase of AP-2/ICAM-1 activation involves activation of serine palmitoyltransferase,36 which is central to ceramide synthesis. This UVA-mediated signalling response, which appears to involve an early non-enzymatic (photochemical) step, is entirely dependent on the ceramide composition of lipid rafts.37
UVA generates oxidized phospholipids from 1-palmitoyl-2-arachidonoyl-sn-glycero-3-phosphorylcholine (PAPC) in human skin fibroblasts.38 This process depends upon singlet oxygen and these phospholipids are now known to stimulate accumulation of a nuclear factor (NRF-2), a crucial transcription factor which, on migration to the nucleus, leads to up-regulation of a series of antioxidant response genes including aldo-ketoreductases and HO-1.39UVA irradiation of arachidonate-containing phospholipids also generated lipid oxidation products which include epoxyisoprostane-phosphatidylcholine and again induce HO-1 expression in skin cells38via NRF-2.39
A crucial UVA-generated lipid oxidation product known to be important in signalling is 4-HNE This compound is a strong inducer of HO-1 and is almost certainly involved in its activation by UVA radiation.40 Using specific lipid anti-oxidants, this study also indicated that the origin of the lipid signalling intermediates was internal membrane lipids. In subsequent studies, UVA-generated 4-HNE has been shown to underlie sustained activation of a JUN N-terminal kinase (JNK) and apoptosis41 in a human myelogenous leukaemia cell line (K562). Interestingly, lower 4-HNE levels are observed in cells which over-express glutathione S-transferases and these cells display resistance to apoptosis induced by UVA and other oxidants.42
As part of the study on the link between UVA-mediated lipid oxidation products and activation of HO-1 mRNA, the phospholipase metabolites diacylglycerol and arachidonic acid were also shown to be active in modulating gene expression.40
c
UVA
activation of protein kinases and phosphatases
Signalling pathways usually involve a cascade of protein phosphorylation (kinase) reactions. UVA activates a broad spectrum of protein kinases, the specifics of which vary considerably with skin cell type. In studies undertaken in the early days of signalling science, UVA radiation had been shown to activate protein kinase C (PKC, a phospholipid-dependent ser/thr kinase) in murine fibroblasts,43 normal human epidermal keratinocytes,44 and rat myeloid leukemia cells.45Inhibitor studies later linked PKC signalling to the UVA activation of MMP-1.46Activation of p38 MAP kinase and JNK pathways by UVA has been well characterized and will be discussed in depth by Zhang and Bowden elsewhere in this issue.152 While it is still crucial to define the target genes involved, there are strong arguments to support the notion that activation of kinase-dependent signalling pathways is involved in skin tumor promotion and progression.47,48
UVA irradiation is known to compromise gap junctional intercellular communication and this has been associated with hyperphosphorylation and decreased levels of the highly expressed keratinocyte gap junction protein, Connexin 43.49
The epidermal growth factor receptor (EGFR), a much-studied oncogene involved in development of many cancers, is a membrane surface receptor which is activated by various ligands (e.g.epidermal growth factor) to become an important tyrosine kinase which, in turn, activates several signal transduction pathways (e.g.MAPK, AKT kinase and JNK) that lead to DNA synthesis and cell proliferation. Many receptor tyrosine kinases (including EGFR located at the inner side of the plasma membrane) are activated by short wavelength UV50–53 and UVA leads to a similar activation by inhibiting tyrosine phosphatase activity.54 A series of studies have examined the downstream consequences of EGFR activation by UVA on signalling kinase activation.55–59 Unlike EGF, UVA modifies EGFR independently of kinase activity and the receptor down-regulation by UVA may be involved in promoting apoptosis.59Activation of EGFR by UVA irradiation of HaCaT cells potentiates anchorage-independent growth of the keratinocytes and this may well be relevant to malignant transformation by UVA radiation.60UVA activation of EGFR has been linked with both loss of cell-to-cell contact and the nuclear relocalisation of beta catenin to generate a beta-catenin/T-cell factor 4 (TCF4) complex which mediates activation of MMP-1.61 Again these events would be crucial in stimulating keratinocyte invasiveness following UVA.
Another interesting kinase that is stimulated by UVA is the DNA damage-inducible Ataxia Telangiectasia mutated (ATM) kinase which regulates cell survival and cell cycle checkpoints and again ATM-dependent p53 and JNK pathways have been linked to UVA apoptosis.62 Abelson-related gene (Arg) is also strongly up-regulated by UVA in normal human keratinocytes.63 Finally, PKR (double-stranded RNA-dependent protein kinase R) has also been shown to be activated by extracellular stress including UVA64 with potential anti-viral and apoptotic consequences.
UVA radiation is known to increase the expression of DUSP1/MKP-a specific protein-tyrosine phosphatase,65,66 which can down-regulate mitogen-activated protein kinases by dephosphorylation. However UVA (and UVB) radiations can also degrade and irreversibly inactivate protein tyrosine phosphatases by the combined effect of substrate oxidation and activation of calpain, a calcium-dependent non-lysosomal cysteine protease.67
d
UVA modulation of transcription factors
i
UVA modulation of the transcription factors NRF-2 and BTB and CNC homology 1, basic leucine zipper transcription factor 1 (BACH-1).
Hemeoxygenase 1 is controlled at the transcriptional level by the dynamic interaction of the activating NRF-2/MAFK heterodynamic complex and the repressing BACH-1/MAFK type complex.68 Of all the factors involved in cellular stress responses, NRF-2 is the most studied. This factor is involved in the activation of phase 2 proteins which have been strongly implicated in chemoprevention of carcinogenesis. Under normal (non-stress) conditions Kelch-like-ECH-associated protein 1 KEAP-1 binds to NRF-2 in the cytoplasm and it is continuously degraded through the ubiquitination pathway. Stress (including UVA irradiation) stabilizes the protein which migrates to the nucleus where it binds to small MAF proteins (typically MAFK). This powerful heterodimeric transcription factor complex can then bind to the MARE (MAF associated regulatory element) which is present in the promoter of the HO-1 gene and other stress-activated genes. Apparently, the activation of the NRF-2 stabilisation response by UVA correlates with the UVA generation of oxidized phospholipids39 The biological relevance of NRF-2 activation in light/UVA-induced stress has been shown by using rodent retinal pigment epithelial cells knocked out for NRF-2 or with the repressor KEAP1 disrupted and demonstrating the photosensitivity (300–400 nm) of these cells compared to wild type.69Activation of NRF-2 by various agents (e.g.sulforafane and flavonoid antioxidants such as quercetin) has been implicated in photoprotection.70
UVA radiation leads to stabilization and nuclear accumulation of NRF-2 both in rodent71 and human72 in skin fibroblasts. In the latter study, specific inhibitory small interfering RNAs(SiRNAs)were used to show that NRF-2 is involved in protection against UVA-induced membrane damage. The second study72 also showed that the UVA/NRF-2 activation response is dependent on heme levels. The DNA binding activity and therefore functionality of the transcriptional repressor protein BACH-1 is also dependent on heme.73Heme also promotes degradation of BACH-174 and its nuclear transport.75 Like NRF-2, BACH-1 forms a heterodimeric transcription factor by binding to MAFK but the BACH-1/MAFK complex acts as a negative regulatory protein and binds to the MARE site of HO-1 to prevent transcription.68BACH-1 bound to the HO-1 promoter prevents UVA activation of HO-1 under low heme conditions and knock-down of BACH-1 increases resistance of keratinocytes to UVA-induced membrane damage.76
ii
NFκB
.
NFκB has provided a classical example of redox sensitivity of transcription factors.31 This factor, which has a central role in many inflammatory events, would be expected to be responsive to UVA and indeed it was shown that relatively low doses of UVA activate NFκB binding to DNA in human skin fibroblasts,77 whereas activation of NFκB by UVC and UVB radiations was only observed at very high doses or where there was evidence of significant membrane damage. It was later shown78 that the kinetics of appearance of NFκB accumulation in the nucleus was slow and that this was related to labile iron-dependent nuclear membrane damage. Indeed, this work supported the concept that oxidised lipids are a crucial intermediate in UVA-mediated NFκB activation and may underlie the role of UVA in skin inflammation. There is also evidence that UVA can enhance NFκB binding to DNA in HaCaT cells79 and a human epithelial cell line,80 but studies in normal human melanocytes report both negative79 and positive81 results.
iii AP-1.
AP-1 which is crucially involved in metalloproteinase activation has long been known to be redox sensitive and the REF-1 protein has been strongly implicated in redox regulation of transcription factors including the AP-1 component proteins, c-FOS and c-JUN.82,83 Apparently UVA can alter the redox state of and activate AP-1 components.84UVA radiation strongly activates c-FOS gene expression in human dermal fibroblasts at physiologically relevant doses,85 thus providing a mechanism by which genes (e.g. those coding for stromelysin and MMP-1)86 are UVA-activated via the AP-1 binding elements in their respective promoters. However, as detailed in the section on metalloproteinases below, the pathways leading to metalloproteinase activation by UVA are varied and complex.
In a recent study it has been confirmed that low-dose UVA increased nuclear levels of c-JUN but not c-FOS in JB6 C141 epidermal cells and increased reporter activity of AP-1 promoter elements.87 Importantly, these authors showed that PIN-1, a peptidylprolylisomerase that is overexpressed in most types of cancer tissues, is activated by UVA irradiation of both the skin of hairless mice and epidermal cells. PIN-1 inhibition blocked proliferation and cyclin D1 expression of epidermal cells following UVA treatment.
iv STATs.
Signal transducers and activators of transcription (STATs) which regulate genes involved in cell proliferation, survival, differentiation, angiogenesis and invasion are among the most studied classes of transcription factors because they are often inappropriately activated in cancer and have been implicated in the carcinogenesis process. STAT-1 binding to its target promoter was enhanced by low UVA doses in a keratinocyte cell line (NCTC 2544) but inhibited by higher doses.88Inhibitor studies indicated that phosphorylation by both serine/threonine kinases and MEK was involved. Nuclear transport of STAT-1 has been observed after UVA.89Phosphorylation of STAT-3 (at Ser 727) has been observed in a mouse epidermal cell line (JB6) following UVA radiation and this occurs viamitogen and stress-activated protein kinase (MSK-1) and JNKs but not extracellular signal-regulated kinases (ERKs) or p38 kinase.90 The biological relevance of UVA modulation of STAT activity remains to be elucidated.
v Activating transcription factor 3 (ATF-3).
Transcription of ATF-3, a member of the cyclic AMP response element binding (CREB) family of transcription factors is substantially induced by relatively low (non-lethal) doses of UVA radiation.91 Again the biological relevance of this UVA-dependent response is not yet clear but ATF-3 dependent genes are involved in immunity and implicated in cancer development.
e
UVA
activation of genes influencing cellular redox homeostasis and inflammation
i
Hemeoxygenase 1.
HO-1 was the first gene to be shown to be activated by an imbalance in cellular redox state in mammalian cells and the initial reports in human skin fibroblasts26,27 derived from studies which used UVA radiation as a natural oxidising carcinogen at biologically relevant doses. It is by far the most dramatic gene activation response to oxidative stress observed in mammalian cells and, like other UVA-mediated gene activation responses, the first event appears to be the UVA generation of singlet oxygen. HO-1 levels (basal and induced) are exquisitely dependent on cellular redox status and can be manipulated by altering GSH levels in cells.5 As mentioned above, UVA is a powerful lipid oxidant and HO-1 is involved in protection against oxidative membrane damage.92 However it has also been recognized as a major anti-apoptotic and anti-inflammatory protein and has been implicated in numerous pathologies.93,94
The central role of hemeoxygenases is in heme catabolism. This activity is essential to degrade heme in a controlled fashion during erythrocyte turnover but it is also an essential cellular factor to keep free heme levels low. Oxidants including UVA can release free heme in cells23 and this must be removed to maintain heme and redox homeostasis (Fig. 3).
 |
| Fig. 3 Disturbance and restoration of heme and iron homeostasis following UVA irradiation. | |
As described above, the promoters of both the rodent and human HO-1 genes contain crucial response elements (MAREs) and a dynamic equilibrium between activation and repression of transcription of the gene is maintained by the relative activities of the NRF-2 and BACH-1 heterodimeric transcription factors respectively.68 The demonstration of the crucial nature of these factors in HO-1 expression allowed us to further investigate our earlier hypothesis95 that heme was a crucial factor in modulating the activity of this gene. This study showed that NRF-2 is stabilised by UVA radiation and that this stabilisation is mediated by the UVA release of heme.72 The heme molecule is central to the repression and UVA released heme will bind to BACH-1 and release its binding to the HO-1 promoter.76,96 Together, these studies evidence the central role of free heme in HO-1 gene expression.
In contrast to skin fibroblasts and melanocytes,27,97 epidermal keratinocytes appear not to require the inducible HO-1 pathway since they show constitutive expression of HO-2.98 Again, HO-2 was believed to prevent UVA activation of HO-1 by mopping up free heme but the role of HO-2 in suppressing HO-1 activation has only recently been verified.76 Specific silencing of HO-2 by SiRNA knockdown leads to strong basal and UVA-induced expression of HO-1 even in epidermal keratinocytes. Importantly, it has been shown that it is BACH-1 that is central to the suppression of HO-1 in fibroblasts since SiRNA knockdown of expression of this protein leads to a massive constitutive expression of HO-1. The expression level cannot be further increased by inducers such as UVA radiation or by knocking down HO-2. Finally, a central role for heme in mediating the expression level of HO-1 could also explain the strong refractoriness to UVA induction of HO-1 by a second delayed dose of UVA95 since a few hours after UVA irradiation, induced HO-1 levels will be high and free heme levels very low.
Maintaining low levels of free heme is crucial to preventing cellular damage and it would appear that the primary role for the activation of HO-1 following oxidative stress, including that induced by UVA radiation, is the restoration of heme homeostasis (Fig. 3). However, it is important to note that the catabolism of free heme results in the release of iron and that this will add to the level of labile iron released immediately after UVA treatment as a result of lysosomal destruction.20UVA-mediated oxidative stress therefore leads not only to a disruption of heme homeostasis but also to a disruption of iron homeostasis. Enhanced free iron levels will exacerbate oxidative stress by acting as a catalyst in Fenton chemistry and the lipid peroxidation chain reaction. The release of iron following UVA treatment will activate another regulatory protein, the iron responsive protein, which results in the strong induction of the iron storage protein ferritin.99 The link between UVA and iron homeostasis is further described by Aroun et al. in this issue.151 It is evident that the immediate induction of HO-1 and the delayed induction of ferritin following UVA are intimately linked and are crucial to restoring heme and iron homeostasis in cells and thereby maintaining cellular redox homeostasis.
ii
Antioxidant enzymes.
The classical cellular antioxidant enzymes, superoxide dismutases (SODs, which convert superoxide anion to hydrogen peroxide), glutathione peroxidases (GPx, which remove cellular peroxides including hydrogen peroxide), peroxiredoxins (which reduce a broad range of peroxides) and catalase (which converts hydrogen peroxide to water) are available in most cells to provide essential antioxidant defense against UVA irradiation and back up the constitutive defense provided by reduced GSH (which is present in skin tissue at millimolor levels) and other anti-oxidant components including anti-oxidant vitamins. Glutathione is progressively destroyed with increasing doses of UVA. The activity of catalase, which contains a UVA-absorbing heme-containing chromophore, is also reduced by UVA radiation (see above) and so the activity of the other antioxidant enzymes following UVA becomes particularly relevant.
A series of studies have mapped changes in antioxidant enzyme activity in different skin tissue100 and showed gradients of activity.101 Work in human skin fibroblasts102 had indicated that acute exposure to UVA and incubation for several days led to little overall change in SOD and GPx activity in living human fibroblasts but did result in loss of catalase activity. Chronic irradiation of rodent skin103 had shown a similar outcome. However, studies using cultured cells demonstrated that repeated acute doses of UVA (at an appropriate interval, 12 h but not 24 h) led to an approximately 5-fold increase in manganese-dependent superoxide dismutase (MnSOD) in human skin fibroblasts and that this was protective against high dose UVA exposure.104 In complementary work shortly afterwards,105 it was shown that similar repeat dose UVA irradiation conditions led to a substantial upregulation of selenium-dependent GPx and that under selenium-supplemented conditions this activity complemented the protection provided by the enhanced MnSOD levels. Although similar studies have not been carried out in human skin, the prediction is that both induced MnSOD and GPx will add to the antioxidant defense required against solar UVA and compensate, at least in part, for the loss of catalase and GSH activity.
iii
NADPH
oxidase 1.
UVA is now known to activate NOX-1 and this is a primary source of UVA-induced ROS generation (primarily superoxide anion) in cells at sublethal doses.16 A direct consequence of this increase in ROS (as shown by SiRNA knockdown experiments) is rapid initiation of prostaglandin E2 synthesis.
iv
Thioredoxin interacting protein.
Thioredoxin is an important cellular reducing agent which is inhibited by the thioredoxin interacting protein (TXNIP). UVA exposure up-regulates TXNIP gene expression in cutaneous melanoma cells thereby lowering thioreoxin activity and increasing cellular ROS which, in turn, leads to intravasation of melanoma cells.106
v Methionine-S-sulfoxidereductase.
The enzyme methionine-S-sulfoxidereductase which repairs oxidized proteins is expressed in human epidermis and upregulated by low doses of UVA radiation (and hydrogen peroxide), but not by UVB.107 Its expression was also shown to be increased by repetitive exposure of human skin to simulated sunlight. The enzyme reduces methionine sulfoxide to methionine, thus reversing the inactivation of proteins caused by oxidation of critical methionine residues. It is the only enzyme so far identified in human skin that is able to repair oxidative protein damage.
vi Intercellular adhesion molecule 1.
ICAM-1 plays an important role in mediating leucocyte/keratinocyte adhesion and is therefore crucial to the inflammatory response. UVA induces ICAM-1 in human skin keratinocytes29 and the activation of the AP-2 transcription factor on the ICAM-1 promoter of the human keratinocyteICAM-1 gene was shown to be the mechanism underlying this response.33 This model system was also used to show the crucial role of ceramide in UVA signalling (see above). Interestingly, ICAM-1 (and E-Selectin but not VCAM-1) was induced by UVA but not UVB in human dermal microvascular epithelial cells,108 indicating an important role for these cells in skin inflammation. Further studies from the same group109 demonstrated that repeated exposure to UVA actually abrogated leucocyte adhesion to endothelial cells. In contrast, UVA has been shown to lead to a decrease in ICAM-1 expression in both epidermis and epidermal keratinocytes and although some increase in ICAM-1 was observed in dermal fibroblasts, ICAM-staining in the dermis was specific for vascular structures,110 a finding consistent with previous data.108
vii
Cyclooxygenase-2 (COX-2).
Cyclooxygenases generate the inflammatory mediators known as prostaglandins from arachidonate. Although such intermediates have been implicated in UVB induction of erythema, studies using indomethacin (a COX inhibitor) indicated that prostaglandins were not involved in UVA erythema.111 Nevertheless, COX activity was measured in human and murine skin fibroblasts30 following UVA irradiation and activation of COX-2 has been clearly demonstrated in HaCaT cells23 and in artificial human epidermis.112Activation in keratinocytes appears to be mediated by p38 stabilisation of the COX-2 mRNA.113 These authors conclude that MAPKs, JNK and p38 are all involved in COX-2 stimulation following UVA activation and that AP-1 is a major factor.
f
UVA
activation of metalloproteinases and other proteases
i Metalloproteinases.
UVA irradiation activates a series of metalloproteinases in human skin fibroblasts (Table 1) and it is believed that these play a key role in elimination of damaged/oxidised proteins and in remodelling of damaged skin. Chronic activation of these multiple proteases in the dermis together with sustained oxidative protein damage will very likely lead to irreversible damage to the extracellular matrix and contribute to the photoaging process.
Table 1
UVA
activation of a variety of metalloproteinases in human skin cells
|
Fibroblasts
|
Keratinocytes
|
*, **, *** refer to the relative levels of activation (low, medium or high respectively. |
Metalloproteinase 1 (MMP-1) collagenase |
*** |
Lowered (normal cells); enhanced in transformed cells, e.g.HaCaT |
Metalloproteinase 2 (MMP-2) type iv collagenase, gelatinase A |
** |
Lowered |
Metalloproteinase 3 (MMP-3) stromelysin 1 |
** |
* |
Metalloproteinase 9 (MMP-9) gelatinase B |
|
Lowered (acute) in normal human keratinocytes. Increased secretion (chronic, HaCaT) |
Metalloproteinase 10 (MMP-10) stromelysin 2 |
|
Enhanced in transformed cells, e.g.HaCaT |
UVB and UVA radiation both activate MMP-1 in human skin fibroblasts. Studies with UVB radiation led to the proposal several years ago that tissue-degrading metalloproteinases are involved in photoaging114,115 and strong activation by UVA radiation will clearly contribute to this process. The enzymatic capacity for extracellular matrix synthesis and degradation mostly resides in the dermis. MMPs (28 zinc metallo-endopeptidases are currently recognised) break down collagen, gelatine and other matrix proteins and include collagenases (collagen), gelatinases (gelatine), stromelysins and matrilysins (non-secreted membrane type family). It is important to note that MMPs are zymogens, and the signal sequence polypeptides are removed during activation.
MMP-1 was shown to be activated in primary fibroblasts but not in keratinocytes where UVA actually slightly lowered MMP-1 levels.116,117 However UVA activation of MMP-1 was later shown in transformed cells that included a squamous cell carcinoma cell line and HaCaT cells.118–120
Crucially very low doses of UVA radiation lead to activation of MMP-1 in human skin fibroblasts,116 an activation that has again been shown to depend on singlet oxygen.32 At least in cultured fibroblasts, the response is biphasic121 with a first peak of induction at approximately 3 h and a second peak a day later. The first peak is believed to be due to the rapid post-translational activation of interleukin 1 (IL-1) which stimulates IL-6 and the second peak also involves IL-1 and IL-6 but is due to transcriptional activation of these cytokines. There are several studies related to protein kinase involvement in the process. For example, it has been shown that serine/threonine kinase is crucial to MMP-1 activation (at least for UVB) but it is at the level of translational control.122 However, a key finding123 is that UVA activation of MMP-1 is abrogated by phospholipid-hydroperoxide glutathione peroxidase clearly demonstrating the involvement of oxidised lipid in this response, e.g. vialipid peroxide-mediated activation of NFκB which itself is essential to IL-6 activation. Interestingly iron is involved in both NFκB activation by UVA78 and the second peak (24 h) of UVA activation of MMP-1.124
Several intermediates have been implicated in MMP-1 activation by UVA in dermal fibroblasts including macrophage migration inhibitory factor (MIF),125 which is controlled by various upstream protein kinase pathways (PKC, PKA, SRC family tyrosine kinases, MAPK and c-JUN but not p38). A more recent study using knock-out mice has confirmed that the IL-1 beta/MIF is involved in UVA activation of MMP-1.126
New findings on redox regulation of MMP-1 have emerged recently. For example, the antioxidant oxidoreductase thioredoxin-1 has been shown to inhibit UVA-induced MMP-1 activation.127 Although these authors concluded that this was an effect on transcription, redox regulation of MMP-1 could occur at the post-translational level. Reduced thiols such as cysteine or glutathione substantially reduced MMP-1 activity128 and this effect was reversed by oxidising radicals (e.g. the trolox radical). The authors concluded that these observations reflected redox control viaderivatization or oxidation of a GSH/MMP-1 complex. As for HO-1, lipid signalling (involving the UVA generation of lipid peroxides) has been implicated in MMP-1 activation in skin fibroblasts.124 Repeated (chronic) exposure of human skin fibroblasts to UVA radiation leads to a sustained overexpression of MMP-1.129 Recently UVA has been shown to down-regulate the forkhead box gene, group O (FOXO) transcription factor (crucial in regulating insulin/insulin-like growthfactor-1 signalling)130 and since knock-down of FOXO-1A expression led to increased expression of MMP-1 and -2 this pathway is also implicated in regulating metalloproteinase expression.
Among agents that have been shown to protect against MMP-1 activation by UVA are flavonoids.21 Beta carotene can also prevent UVA-mediated MMP-1 activation in skin cells120 and dietary beta carotene can suppress MMP-9 activation in mice.131 The authors conclude that this systemic effect is due to the prevention of cholesterol peroxidation which is known to be mediated by UVA and to be a trigger for gene activation.
The proteasome has also been implicated in the UVA stress response involving metalloproteinases132 since overexpression of either the proteasome or methionine sulfoxide reductase (which repairs oxidized proteins) prevents UVA activation of MMP-1. Under conditions where the proteasome is inhibited, MMP-1 signalling pathways are activated (viac-JUN phosphorylation and AP-1 activation).
As well as MMP-1, metalloproteinases 2 (type IV collagenase, gelatinase A) and 3 (stromelysin 1) have also been reported to be induced in human fibroblasts after UVA treatment.28Activation of MMP-2 (and reduction in tissue inhibitor of metalloproteinase 2, TIMP-2, activity) has been linked to increased secretion of thioredoxin following UVA treatment by UVA. Although other studies have independently reported UVA induction of MMP-2 in fibroblasts,133,134 a study in normal human keratinocytes135 showed that as for MMP-1, UVA quite significantly lowered expression of both MMP-2 and MMP-9 (gelatinase B). No change in secretion of these proteins was seen136 but chronic (18 week) re-exposure of HaCaT cells to UVA treatment137 actually led to increased secretion of matrix metalloproteinase 9 (MMP-9). In studies with human microvascular endothelial cells, UVA was shown to up-regulate MMP-1 but down-regulate MMP-2138 and this has been linked to the impaired angiogenic phenotype of the dermal endothelial cells. The induction of MMP-3 in fibroblasts by UVA has been reported and, as for MMP-1, the crucial cis-acting promoter element is an AP-1 site.86 MMP-3 is also induced in HaCaT cells.120 Induction of MMP-10 (stromelysin-2) by UVA has been observed in a human squamous cell carcinoma line118 and in HaCaT cells.119
Recent studies in mice139 demonstrated that the UVA activation of MMP-9 correlated with increased peroxidised cholesterol formation and increased sagging and wrinkling of the skin. Direct injection of cholesterol hydroperoxides also led to MMP-9 activation supporting the concept that lipid peroxides are key to the UVA induced activation of metalloproteinases.131
ii Other proteases.
Recently, there has been considerable interest in the cathepsin family of proteases. The lysosomal protease, cathepsin K (CATK), is a potent mammalian elastase and may play a role (among other elastases) in formation of solar elastosis. UVA activation of this potent elastase has been observed in human fibroblasts from young donors both in vitro and in vivo.140 Importantly the authors observed much reduced inducible elastase activity in fibroblasts derived from the skin of aging donors. They suggested that this age-related decline in CATK activity, which was also evident after UVA exposure, may “promote the formation of actinic elastosis through a decline of orderly intracellular elastin degradation and subsequent accumulation of elastin in the extracellular space.”
An interesting study in human skin fibroblasts from Wondrak's group141 has shown that the enzymatic activity of cathepsin B (CATB), a lysosomal cysteine protease is substantially reduced by repeated UVA irradiation which also compromised CATB maturation. The consequent impairment of lysosomal capacity to remove lipofuscin would be a clear source of dermal damage. A more recent study reported in this issue (Lamore and Wondrak) has indicated that autophagic-lysosomal alterations observed after UVA are the result of impaired autophagy downstream of CATB inactivation.153
Another lysosomal protease, CATL, which degrades matrix proteins was not modified by acute exposure of fibroblasts to UVA but chronic irradiation using repeated exposure led to significant extracellular release of the protein.142 Furthermore, this was associated with a reduction in intracellular processed CATL and an accumulation of unprocessed cathepsin.
UVA may stimulate proteases viaactivation of p38 kinase (see above) in human skin keratinocytes. For example, UVA activation of p38 MAPK in keratinocytes leads to granzyme B (a serine protease) and this will facilitate degradation of extracellular matrix components.143Redox-dependent MIF release appears to be involved.
The serious perturbation of metalloproteinase and other types of protease activity/maturation by UVA radiation will undoubtedly prove to have dramatic long-term consequences in skin. The imbalance in synthesis of MMPs, TIMPs and other proteases following acute and chronic UVA is likely to be a major factor in the dissolution of dermal and basement structures and eventual photoaging.
g Modulation of expression of other genes by UVA
An interesting preliminary report144 indicated a UVA-mediated up-regulation of N-Cadherin and a down-regulation of E-cadherin in a murine melanoma cell line. This was associated with a decrease in self-adhesion of melanoma cells and an increase in melanoma adhesion to epithelial cells with evident implications for metastatic potential. These authors also demonstrated the UVA-induced metastatic potential of these melanoma cells in mice.145 However, in a study of melanocyte proliferation in hairless SKH-2 mice, it was found that while a single (but not fractionated) dose of UVB led to a strong enhancement in proliferation, no change in proliferation rate was observed following any type of UVA dose regimen.146 In a study comparing UVA and UVB induced gene expression in primary human melanocytes,147proteins involved in cell growth (p73 and Nup88) were notably enhanced in UVA-irradiated cells. Induction of expression of these genes has not been noted in other cell types.
UVA irradiation of mouse epidermis or a murine keratinocyte cell line has been shown to result in induction of an oxidant-susceptible calcium-binding protein (S100A8) of significance in human psoriasis.148
Finally, UVA has been shown149 to induce a reduction in neuron-specific enolase levels in acrosclerosis (a type of auto-immune rheumatic disorder). UVA has been used as an adjuvant treatment in moderately active systemic lupus erythematosus.150 Such treatment has been associated with softening of sclerotic lesions but other UVA-mediated events such as T-cell apoptosis, metalloproteinase induction and angiogenesis may all also be involved.
Concluding remarks
While normal low level exposures to the UVA component of sunlight will generate a significant oxidative stress throughout the skin and this is further exacerbated by the UVA-mediated release of pro-oxidant catalysts (iron and heme), a consequence of this altered redox state is the activation of several enzymes which act to restore the redox balance (Fig. 2). The activation of MnSOD and GPx will reduce superoxide and peroxide levels respectively thus directly contributing to the restoration of redox homeostasis. Enhanced hemeoxygenase activity results in the mopping up of free heme and a consequence of this appears to be the partial reversal of oxidative membrane damage. However a by-product of heme catabolism is free iron which, together with the labile iron directly released in cells by UVA radiation, will fuel Fenton chemistry, enhance hydroxyl radical generation and catalyse the lipid peroxidation chain reaction. A further contribution to the oxidative stress, at least in epidermal cells, will be the activation of membrane-associated NOX-1 oxidase which will generate enhanced superoxide anion levels. Clearly while cells and tissue have developed ways to counter redox stress, including that generated by UVA radiation, the potential exists for these systems to be overwhelmed and result in various types of oxidative damage. Damage to skin itself may be further exacerbated by the activation of many proteases, including matrix metalloproteinases, which will progressively erode the structure of skin on repeated and chronic exposure to the UVA component of sunlight.
List of abbreviations
4-HNE
|
4-hydroxynonenal
|
AP-1 | Activator protein 1 |
ATM
| Ataxia Telangiectasia mutated |
BACH-1
|
BTB and CNC homology 1, basic leucine zipper transcription factor 1 |
CATK
| cathepsin K |
EGFR
|
epidermal growth factor receptor
|
GPx
|
glutathione peroxidases |
GSH
|
glutathione
|
HO-1
|
hemeoxygenase 1 |
ICAM-1
| intercellular adhesion molecule |
IL-1
| interleukin 1 |
JNK
| JUN N-terminal kinase |
MARE
|
MAF associated regulatory element |
MIF
| migration inhibitory factor |
MMP-1 | matrix metalloproteinase 1 |
MnSOD
|
manganese-dependent superoxide dismutase |
NF-κB
| nuclear factor kappa-light-chain-enhancer of activated B cells |
NOX-1
|
NADPH
oxidase 1 |
NRF-2
| nuclear factor (erythroid-derived 2)–like 2 |
PKC
|
protein kinase C
|
ROS |
reactive oxygen species
|
SiRNA
|
small interfering RNAs
|
STATs | signal transducers and activators of transcription |
References
- R. B. Webb and J. R. Lorenz, Oxygen dependence and repair of lethal effects of near ultraviolet and visible light, Photochem. Photobiol., 1970, 12, 283–289 CrossRef CAS.
-
R. M. Tyrrell, UVA (320–380 nm) radiation as an oxidative stress in Oxidative Stress: Oxidants and antioxidants, ed. H. Sies, Academic Press, London, 1991, pp. 57–83 Search PubMed.
- H. J. Danpure and R. M. Tyrrell, Oxygen-dependence of near UV (365 nm) lethality and the interaction of near UV and X-rays in two mammalian cell lines, Photochem. Photobiol., 1976, 23, 171–177 CrossRef CAS.
- R. M. Tyrrell and M. Pidoux, Endogenous glutathione protects human skin fibroblasts against the cytotoxic action of UVB, UVA and near-visible radiations, Photochem. Photobiol., 1986, 44, 561–564 CrossRef CAS.
- D. Lautier, P. Luscher and R. M. Tyrrell, Endogenous glutathione levels modulate both constitutive and UVA radiation/hydrogen peroxide inducible expression of the human heme oxygenase gene, Carcinogenesis, 1992, 13, 227–232 CrossRef CAS.
- L. Hellemans, H. Corstjens, A. Neven, L. Declercq and D. Maes, Antioxidant enzyme activity in human stratum corneum shows seasonal variation with an age-dependent recovery, J. Invest. Dermatol., 2003, 120, 434–439 CrossRef CAS.
- H. Yasui and H. Sakurai, Age-dependent generation of reactive oxygen species in the skin of live hairless rats exposed to UVA light, Exp. Dermatol., 2003, 12, 655–661 CrossRef CAS.
- H. Ou-Yang, G. Stamatas, C. Saliou and N. Kollias, A chemiluminescence study of UVA-induced oxidative stress in human skin in vivo, J. Invest. Dermatol., 2004, 122, 1020–1029 CrossRef.
- T. Herrling, J. Fuchs, J. Rehberg and N. Groth, UV-induced free radicals in the skin detected by ESR spectroscopy and imaging using nitroxides, Free Radical Biol. Med., 2003, 35, 59–67 CrossRef CAS.
- A. Jain, I. Rieger, M. Rohr and A. Schrader, Antioxidant efficacy on human skin in vivo investigated by UVA-induced chemiluminescence decay analysis via induced chemiluminescence of human skin, Skin Pharmacol. Physiol., 2010, 23, 266–272 CrossRef CAS.
- G. Rhie, M. H. Shin, J. Y. Seo, W. W. Choi, K. H. Cho, K. H. Kim, K. C. Park, H. C. Eun and J. H. Chung, Aging- and photoaging-dependent changes of enzymic and nonenzymic antioxidants in the epidermis and dermis of human skin in vivo, J. Invest. Dermatol., 2001, 117, 1212–1217 CrossRef CAS.
- R. M. Tyrrell and M. Pidoux, Singlet oxygen involvement in the inactivation of cultured human fibroblasts by UVA (334 nm, 365 nm) and near-visible (405 nm) radiations, Photochem. Photobiol., 1989, 49, 407–412 CrossRef CAS.
- S. Basu-Modak and R. M. Tyrrell, Singlet oxygen: a primary effector in the ultraviolet A/near-visible light induction of the human heme oxygenase gene, Cancer Res., 1993, 53, 4505–4510 CAS.
- R. M. Tyrrell, Oxidant, antioxidant status and photocarcinogenesis: the role of gene activation, Photochem. Photobiol., 1996, 63, 380–383 CrossRef CAS.
- S. W. Ryter and R. M. Tyrrell, Singlet molecular oxygen ((1)O2): a possible effector of eukaryotic gene expression, Free Radical Biol. Med., 1998, 24, 1520–1534 CrossRef CAS.
- A. Valencia and I. E. Kochevar, Nox1-based NADPH oxidase is the major source of UVA-induced reactive oxygen species in human keratinocytes, J. Invest. Dermatol., 2007, 128, 214–222 CrossRef.
- Y. Y. He, J. L. Huang, M. L. Block, J. S. Hong and C. F. Chignell, Role of phagocyte oxidase in UVA-induced oxidative stress and apoptosis in keratinocytes, J. Invest. Dermatol., 2005, 125, 560–566 CrossRef CAS.
- S. W. Ryter and R. M. Tyrrell, The heme synthesis and degradation pathways: role in oxidant sensitivity. Heme oxygenase has both pro- and antioxidant properties, Free Radical Biol. Med., 2000, 28, 289–309 CrossRef CAS.
-
R. M. Tyrrell, Heme oxygenase – a crucial enzyme in iron and heme homeostasis in Heme oxygenase: The elegant orchestration of its products in medicine, ed. L. Otterbein and B. S. Zuckerbraun, Nova Science Publishers, New York, 2005, pp. 333–349 Search PubMed.
- C. Pourzand, R. D. Watkin, J. E. Brown and R. M. Tyrrell, Ultraviolet A radiation induces immediate release of iron in human primary skin fibroblasts:
the role of ferritin, Proc. Natl. Acad. Sci. U. S. A., 1999, 96, 6751–6756 CrossRef CAS.
- S. Basu-Modak, D. Ali, M. Gordon, T. Polte, A. Yiakouvaki, C. Pourzand, C. Rice-Evans and R. M. Tyrrell, Suppression of UVA-mediated release of labile iron by epicatechin–a link to lysosomal protection, Free Radical Biol. Med., 2006, 41, 1197–1204 CrossRef CAS.
- J. L. Zhong, A. Yiakouvaki, P. Holley, R. M. Tyrrell and C. Pourzand, Susceptibility of skin cells to UVA-induced necrotic cell death reflects the intracellular level of labile iron, J. Invest. Dermatol., 2004, 123, 771–780 CrossRef CAS.
- E. Kvam, A. Noel, S. Basu-Modak and R. M. Tyrrell, Cyclooxygenase dependent release of heme from microsomal hemeproteins correlates with induction of heme oxygenase 1 transcription in human fibroblasts, Free Radical Biol. Med., 1999, 26, 511–517 CrossRef CAS.
- R. M. Tyrrell, Activation of mammalian gene expression by the UV component of sunlight - from models to reality, BioEssays, 1996, 18, 139–148 CrossRef CAS.
-
R. M. Tyrrell, UV activation of mammalian stress proteins, in Stress-inducible cellular responses ed. U. Feige, R. I. Morimoto, I. Yahara and B. S. Polla, Birkhäuser Verlag, Basel, 1996b, pp. 255–272 Search PubMed.
- S. M. Keyse and R. M. Tyrrell, Both near ultraviolet radiation and the oxidizing agent hydrogen peroxide induce a 32-kDa stress protein in normal human skin fibroblasts, J. Biol. Chem., 1987, 262, 14821–14825 CAS.
- S. M. Keyse and R. M. Tyrrell, Heme oxygenase is the major 32-kDa stress protein induced in human skin fibroblasts by UVA radiation, hydrogen peroxide, and sodium arsenite, Proc. Natl. Acad. Sci. U. S. A., 1989, 86, 99–103 CrossRef CAS.
- G. Herrmann, M. Wlaschek, T. S. Lange, K. Prenzel, G. Goerz and K. Scharffetter-Kochanek, UVA irradiation stimulates the synthesis of various matrix-metalloproteinases (MMPs) in cultured human fibroblasts, Exp. Dermatol., 1993, 2, 92–97 CrossRef CAS.
- J. Krutmann and M. Grewe, Involvement of cytokines, DNA damage, and reactive oxygen intermediates in ultraviolet radiation-induced modulation of intercellular adhesion molecule-1 expression, J. Invest. Dermatol., 1995, 105, 67S–70S CAS.
- D. L. Hanson and V. A. DeLeo, Long wave ultraviolet radiation stimulates arachidonic acid release and cyclooxygenase activity in mammalian cells in culture, Photochem. Photobiol., 1989, 49, 423–430 CrossRef CAS.
- R. Schreck, K. Albermann and P. A. Baeuerle, Nuclear factor kappa B: an oxidative stress-responsive transcription factor of eukaryotic cells (a review), Free Radical Res., 1992, 17, 221–237 CrossRef CAS.
- M. Wlaschek, K. Briviba, G. P. Stricklin, H. Sies and K. Scharffetter-Kochanek, Singlet oxygen may mediate the ultraviolet A-induced synthesis of interstitial collagenase, J. Invest. Dermatol., 1995, 104, 194–198 CAS.
- S. Grether-Beck, S. Olaizola-Horn, H. Schmitt, M. Grewe, A. Jahnke, J. P. Johnson, K. Briviba, H. Sies and J. Krutmann, Activation of transcription factor AP-2 mediates UVA radiation- and singlet oxygen-induced expression of the human intercellular adhesion molecule 1 gene, Proc. Natl. Acad. Sci. U. S. A., 1996, 93, 14586–14591 CrossRef CAS.
- S. Grether-Beck, G. Bonizzi, H. Schmitt-Brenden, I. Felsner, A. Timmer, H. Sies, J. P. Johnson, J. Piette and J. Krutmann, Non-enzymatic triggering of the ceramide signalling cascade by solar UVA radiation, EMBO J., 2000, 19, 5793–5800 CrossRef CAS.
- C. Maziere, M. A. Conte, L. Leborgne, T. Levade, W. Hornebeck, R. Santus and J. C. Maziere, UVA radiation stimulates ceramide production: relationship to oxidative stress and potential role in ERK, JNK, and p38 activation, Biochem. Biophys. Res. Commun., 2001, 281, 289–294 CrossRef CAS.
- S. Grether-Beck, A. Timmer, I. Felsner, H. Brenden, D. Brammertz and J. Krutmann, Ultraviolet A-induced signaling involves a
ceramide-mediated autocrine loop leading to ceramide de novo synthesis, J. Invest. Dermatol., 2005, 125, 545–553 CrossRef CAS.
- S. Grether-Beck, M. Salahshour-Fard, A. Timmer, H. Brenden, I. Felsner, R. Walli, J. Fullekrug and J. Krutmann, Ceramide and raft signaling are linked with each other in UVA radiation-induced gene expression, Oncogene, 2008, 27, 4768–4778 CrossRef CAS.
- F. Gruber, O. Oskolkova, A. Leitner, M. Mildner, V. Mlitz, B. Lengauer, A. Kadl, P. Mrass, G. Kronke, B. R. Binder, V. N. Bochkov, N. Leitinger and E. Tschachler, Photooxidation generates biologically active phospholipids that induce heme oxygenase-1 in skin cells, J. Biol. Chem., 2007, 282, 16934–16941 CrossRef CAS.
- F. Gruber, H. Mayer, B. Lengauer, V. Mlitz, J. M. Sanders, A. Kadl, M. Bilban, R. de Martin, O. Wagner, T. W. Kensler, M. Yamamoto, N. Leitinger and E. Tschachler, NF-E2-related factor 2 regulates the stress response to UVA-1-oxidized phospholipids in skin cells, FASEB J., 2009, 24, 39–48 CrossRef CAS.
- S. Basu-Modak, P. Luscher and R. M. Tyrrell, Lipid metabolite involvement in the activation of the human heme oxygenase-1 gene, Free Radical Biol. Med., 1996, 20, 887–897 CrossRef CAS.
- Y. Yang, A. Sharma, R. Sharma, B. Patrick, S. S. Singhal, P. Zimniak, S. Awasthi and Y. C. Awasthi, Cells preconditioned with mild, transient UVA irradiation acquire resistance to oxidative stress and UVA-induced apoptosis: role of 4-hydroxynonenal in UVA-mediated signaling for apoptosis, J. Biol. Chem., 2003, 278, 41380–41388 CrossRef CAS.
- Y. C. Awasthi, Y. Yang, N. K. Tiwari, B. Patrick, A. Sharma, J. Li and S. Awasthi, Regulation of 4-hydroxynonenal-mediated signaling by glutathione S-transferases, Free Radical Biol. Med., 2004, 37, 607–619 CrossRef CAS.
- M. S. Matsui and V. A. DeLeo, Induction of protein kinase C activity by ultraviolet radiation, Carcinogenesis, 1990, 11, 229–234 CrossRef CAS.
- M. S. Matsui, N. Wang, D. MacFarlane and V. A. DeLeo, Long-wave ultraviolet radiation induces protein kinase C in normal human keratinocytes, Photochem. Photobiol., 1994, 59, 53–57 CrossRef CAS.
- D. Leszczynski, K. Leszczynski and K. Servomaa, Long-wave ultraviolet radiation causes increase of membrane-bound fraction of protein kinase C in rat myeloid leukemia cells, Photodermatol., Photoimmunol. Photomed., 1995, 11, 124–130 CrossRef CAS.
- M. Petersen, T. Hamilton and H. L. Li, Regulation and inhibition of collagenase expression by long-wavelength ultraviolet radiation in cultured human skin fibroblasts, Photochem. Photobiol., 1995, 62, 444–448 CrossRef CAS.
- M. A. Bachelor and G. T. Bowden, UVA-mediated activation of signaling pathways involved in skin tumor promotion and progression, Semin. Cancer Biol., 2004, 14, 131–138 CrossRef CAS.
- A. L. Silvers, J. S. Finch and G. T. Bowden, Inhibition of UVA-induced c-Jun N-terminal kinase activity results in caspase-dependent apoptosis in human keratinocytes, Photochem. Photobiol., 2006, 82, 423–431 CrossRef CAS.
- B. Bellei, A. Mastrofrancesco, S. Briganti, N. Aspite, N. Ale-Agha, H. Sies and M. Picardo, Ultraviolet A induced modulation of gap junctional intercellular communication by P38 MAPK activation in human keratinocytes, Exp. Dermatol., 2008, 17, 115–124 CrossRef CAS.
- Y. Devary, R. A. Gottlieb, T. Smeal and M. Karin, The mammalian ultraviolet response is triggered by activation of Src tyrosine kinases, Cell, 1992, 71, 1081–1091 CrossRef CAS.
- C. Sachsenmaier, A. Radler-Pohl, R. Zinck, A. Nordheim, P. Herrlich and H. J. Rahmsdorf, Involvement of growth
factor receptors in the mammalian UVC response, Cell, 1994, 78, 963–972 CrossRef CAS.
- G. L. Schieven, R. S. Mittler, S. G. Nadler, J. M. Kirihara, J. B. Bolen, S. B. Kanner and J. A. Ledbetter, ZAP-70 tyrosine kinase, CD45, and T cell receptor involvement in UV- and H2O2-induced T cell signal transduction, J. Biol. Chem., 1994, 269, 20718–20726 CAS.
- P. J. Coffer, B. M. Burgering, M. P. Peppelenbosch, J. L. Bos and W. Kruijer, UV activation of receptor tyrosine kinase activity, Oncogene, 1995, 11, 561–569 CAS.
- A. Knebel, H. J. Rahmsdorf, A. Ullrich and P. Herrlich, Dephosphorylation of receptor tyrosine kinases as target of regulation by radiation, oxidants or alkylating agents, EMBO J., 1996, 15, 5314–5325 CAS.
- Y. Zhang, Z. Dong, A. M. Bode, W. Y. Ma and N. Chen, Induction of EGFR-dependent and EGFR-independent signaling pathways by ultraviolet A irradiation, DNA Cell Biol., 2001, 20, 769–779 CrossRef CAS.
- C. Maziere, S. Floret, R. Santus, P. Morliere, V. Marcheux and J. C. Maziere, Impairment of the EGF signaling pathway by the oxidative stress generated with UVA, Free Radical Biol. Med., 2003, 34, 629–636 CrossRef CAS.
- C. Jean, H. Hernandez-Pigeon, A. Blanc, M. Charveron and G. Laurent, Epidermal growth factor receptor pathway mitigates UVA-induced G2/M arrest in keratinocyte cells, J. Invest. Dermatol., 2007, 127, 2418–2424 CrossRef CAS.
- Y. Y. He, S. E. Council, L. Feng and C. F. Chignell, UVA-induced cell cycle progression is mediated by a disintegrin and metalloprotease/epidermal growth factor receptor/AKT/Cyclin D1 pathways in keratinocytes, Cancer Res., 2008, 68, 3752–3758 CrossRef CAS.
- Y. Y. He, J. L. Huang, J. B. Gentry and C. F. Chignell, Epidermal growth factor receptor down-regulation induced by UVA in human keratinocytes does not require the receptor kinase activity, J. Biol. Chem., 2003, 278, 42457–42465 CrossRef CAS.
- C. Y. Han, S. C. Lim, H. S. Choi and K. W. Kang, Induction of ErbB2 by ultraviolet A irradiation: potential role in malignant transformation of keratinocytes, Cancer Sci., 2008, 99, 502–509 CrossRef CAS.
- C. Jean, A. Blanc, N. Prade-Houdellier, L. Ysebaert, H. Hernandez-Pigeon, T. Al Saati, M. J. Haure, A. M. Coluccia, M. Charveron, E. Delabesse and G. Laurent, Epidermal growth factor receptor/beta-catenin/T-cell factor 4/matrix metalloproteinase 1: a new pathway for regulating keratinocyte invasiveness after UVA irradiation, Cancer Res., 2009, 69, 3291–3299 CrossRef CAS.
- Y. Zhang, W. Y. Ma, A. Kaji, A. M. Bode and Z. Dong, Requirement of ATM in UVA-induced signaling and apoptosis, J. Biol. Chem., 2001, 277, 3124–3131 CrossRef.
- G. Klosner, R. Varecka, R. Knobler and F. Trautinger, Ultraviolet-A and -B differentially modify the tyrosine-kinase profile of human keratinocytes and induce the expression of Arg+, Photochem. Photobiol., 2008, 84, 261–265 CrossRef CAS.
- T. A. Zykova, F. Zhu, Y. Zhang, A. M. Bode and Z. Dong, Involvement of ERKs, RSK2 and PKR in UVA-induced signal transduction toward phosphorylation of eIF2alpha (Ser(51)), Carcinogenesis, 2007, 28, 1543–1551 CrossRef CAS.
- S. M. Keyse and E. A. Emslie, Oxidative stress and heat shock induce a human gene encoding a protein-tyrosine phosphatase, Nature, 1992, 359, 644–647 CrossRef CAS.
- D. N. Slack, O. M. Seternes, M. Gabrielsen and S. M. Keyse, Distinct binding determinants for ERK2/p38alpha and JNK map kinases mediate catalytic activation and substrate selectivity of map kinase phosphatase-1, J. Biol. Chem., 2001, 276, 16491–16500 CrossRef CAS.
- P. Gulati, B. Markova, M. Gottlicher, F. D. Bohmer and P. A. Herrlich, UVA inactivates protein tyrosine phosphatases by calpain-mediated degradation, EMBO Rep., 2004, 5, 812–817 CrossRef CAS.
- J. Sun, M. Brand, Y. Zenke, S. Tashiro, M. Groudine and K. Igarashi, Heme regulates the dynamic exchange of Bach1 and NF-E2-related factors in the Maf transcription factor network, Proc. Natl. Acad. Sci. U. S. A., 2004, 101, 1461–1466 CrossRef CAS.
- X. Gao and P. Talalay, Induction of phase 2 genes by sulforaphane protects retinal pigment epithelial cells against photooxidative damage, Proc. Natl. Acad. Sci. U. S. A., 2004, 101, 10446–10451 CrossRef CAS.
- S. Kimura, E. Warabi, T. Yanagawa, D. Ma, K. Itoh, Y. Ishii, Y. Kawachi and T. Ishii, Essential role of Nrf2 in keratinocyte protection from UVA by quercetin, Biochem. Biophys. Res. Commun., 2009, 387, 109–114 CrossRef CAS.
- A. Hirota, Y. Kawachi, K. Itoh, Y. Nakamura, X. Xu, T. Banno, T. Takahashi, M. Yamamoto and F. Otsuka, Ultraviolet A irradiation induces NF-E2-related factor 2 activation in dermal fibroblasts: protective role in UVA-induced apoptosis, J. Invest. Dermatol., 2005, 124, 825–832 CrossRef CAS.
- J. L. Zhong, G. P. Edwards, C. Raval, H. Li and R. M. Tyrrell, The role of Nrf2 in ultraviolet A mediated heme oxygenase 1 induction in human skin fibroblasts, Photochem. Photobiol. Sci., 2010, 9, 18–24 CAS.
- K. Ogawa, J. Sun, S. Taketani, O. Nakajima, C. Nishitani, S. Sassa, N. Hayashi, M. Yamamoto, S. Shibahara, H. Fujita and K. Igarashi, Heme mediates derepression of Maf recognition element through direct binding to transcription repressor Bach1, EMBO J., 2001, 20, 2835–2843 CrossRef CAS.
- Y. Zenke-Kawasaki, Y. Dohi, Y. Katoh, T. Ikura, M. Ikura, T. Asahara, F. Tokunaga, K. Iwai and K. Igarashi, Heme induces ubiquitination and degradation of the transcription factor Bach1, Mol. Cell. Biol., 2007, 27, 6962–6971 CrossRef CAS.
- H. Suzuki, S. Tashiro, S. Hira, J. Sun, C. Yamazaki, Y. Zenke, M. Ikeda-Saito, M. Yoshida and K. Igarashi, Heme regulates gene expression by triggering Crm1-dependent nuclear export of Bach1, EMBO J., 2004, 23, 2544–2553 CrossRef CAS.
- J. L. Zhong, C. Raval, G. P. Edwards and R. M. Tyrrell, A role for Bach1 and HO-2 in suppression of basal and UVA-induced HO-1 expression in human keratinocytes, Free Radical Biol. Med., 2010, 48, 196–206 CrossRef CAS.
- G. F. Vile, A. Tanew-Ilitschew and R. M. Tyrrell, Activation of NF-kappa B in human skin fibroblasts by the oxidative stress generated by UVA radiation, Photochem. Photobiol., 1995, 62, 463–468 CrossRef CAS.
- O. Reelfs, R. M. Tyrrell and C. Pourzand, Ultraviolet a radiation-induced immediate iron release is a key modulator of the activation of NF-kappaB in human skin fibroblasts, J. Invest. Dermatol., 2004, 122, 1440–1447 CrossRef CAS.
- J. Shang, C. Schwarz, H. Sanchez Ruderisch, T. Hertting, C. E. Orfanos and B. Tebbe, Effects of UVA and L-ascorbic acid on nuclear factor-kappa B in melanocytes and in HaCaT keratinocytes, Skin Pharmacol. Appl. Skin Physiol., 2002, 15, 353–359 CrossRef CAS.
- C. E. Hellweg and C. Baumstark-Khan, Detection of UV-induced activation of NF-kappaB in a recombinant human cell line by means of Enhanced Green Fluorescent Protein (EGFP), Radiat. Environ. Biophys., 2007, 46, 269–279 CrossRef CAS.
- P. Larsson, K. Ollinger and I. Rosdahl, Ultraviolet (UV)A- and UVB-induced redox alterations and activation of nuclear factor-kappaB in human melanocytes-protective effects of alpha-tocopherol, Br. J. Dermatol., 2006, 155, 292–300 CrossRef CAS.
- C. Abate, L. Patel, F. J. Rauscher, 3rd and T. Curran, Redox regulation of fos and jun DNA-binding activity in vitro, Science, 1990, 249, 1157–1161 CAS.
- J. M. Ordway, D. Eberhart and T. Curran, Cysteine 64 of Ref-1 is not essential for redox regulation of AP-1 DNA binding, Mol. Cell. Biol., 2003, 23, 4257–4266 CrossRef CAS.
- M. Djavaheri-Mergny, J. L. Mergny, F. Bertrand, R. Santus, C. Maziere, L. Dubertret and J. C. Maziere, Ultraviolet-A induces activation of AP-1 in cultured human keratinocytes, FEBS Lett., 1996, 384, 92–96 CrossRef CAS.
- B. Bose, M. Soriani and R. M. Tyrrell, Activation of expression of the c-fos oncogene by UVA irradiation in cultured human skin fibroblasts, Photochem. Photobiol., 1999, 69, 489–493 CrossRef CAS.
- D. Sawamura, T. Ohta, K. Hanada, H. Ishikawa, K. Tamai, H. Yazima, X. Meng, K. Nomura, I. Hashimoto, A. Mauviel and J. Uitto, Involvement of the AP-1 site within the 5′-flanking region of the stromelysin-1 gene in induction of the gene expression by UVA irradiation, Arch. Dermatol. Res., 1996, 288, 628–632 CrossRef CAS.
- C. Y. Han, T. T. Hien, S. C. Lim and K. W. Kang, Role of Pin1 in UVA-induced cell proliferation and malignant transformation in epidermal cells, Biochem. Biophys. Res. Commun., 2011, 410, 68–74 CrossRef CAS.
- C. Maziere, F. Dantin, F. Dubois, R. Santus and J. Maziere, Biphasic effect of UVA radiation on STAT1 activity and tyrosine phosphorylation in cultured human keratinocytes, Free Radical Biol. Med., 2000, 28, 1430–1437 CrossRef CAS.
- J. V. Alvarez and D. A. Frank, Genome-wide analysis of STAT target genes: elucidating the mechanism of STAT-mediated oncogenesis, Cancer Biol. Ther., 2004, 3, 1045–1050 CrossRef CAS.
- Y. Zhang, G. Liu and Z. Dong, MSK1 and JNKs mediate phosphorylation of STAT3 in UVA-irradiated mouse epidermal JB6 cells, J. Biol. Chem., 2001, 276, 42534–42542 CrossRef CAS.
- T. Abe, N. Oue, W. Yasui and M. Ryoji, Rapid and preferential induction of ATF3 transcription in response to low doses of UVA light, Biochem. Biophys. Res. Commun., 2003, 310, 1168–1174 CrossRef CAS.
- G. F. Vile, S. Basu-Modak, C. Waltner and R. M. Tyrrell, Heme oxygenase 1 mediates an adaptive response to oxidative stress in human skin fibroblasts, Proc. Natl. Acad. Sci. U. S. A., 1994, 91, 2607–2610 CrossRef CAS.
-
L. Otterbein, B. S. Zuckerbraun, ed., Heme oxygenase: The elegant orchestration of its products in medicine, Nova Science Publishers, New York, 2005 Search PubMed.
- M. P. Soares and F. H. Bach, Heme oxygenase-1: from biology to therapeutic potential, Trends Mol. Med., 2009, 15, 50–58 CrossRef CAS.
- A. Noel and R. M. Tyrrell, Development of refractoriness of induced human heme oxygenase-1 gene expression to reinduction by UVA irradiation and hemin, Photochem. Photobiol., 1997, 66, 456–463 CrossRef CAS.
-
C. Raval, The role of Bach 1 in ultraviolet-A mediated human heme oxygenase-1 gene regulation, PhD, University of Bath, Bath, 2008.
- L. Marrot, C. Jones, P. Perez and J. R. Meunier, The significance of Nrf2 pathway in (photo)-oxidative stress response in melanocytes and keratinocytes of the human epidermis, Pigm. Cell Melanoma Res., 2007, 21, 79–88 CrossRef.
- L. A. Applegate, A. Noel, G. Vile, E. Frenk and R. M. Tyrrell, Two genes contribute to different extents to the heme oxygenase enzyme activity measured in cultured human skin fibroblasts and keratinocytes: implications for protection against oxidant stress, Photochem. Photobiol., 1995, 61, 285–291 CrossRef CAS.
- G. F. Vile and R. M. Tyrrell, Oxidative stress resulting from ultraviolet A irradiation of human skin fibroblasts leads to a heme oxygenase-dependent increase in ferritin, J. Biol. Chem., 1993, 268, 14678–14681 CAS.
- Y. Shindo, E. Witt, D. Han, W. Epstein and L. Packer, Enzymic and non-enzymic antioxidants in epidermis and dermis of human skin, J. Invest. Dermatol., 1994, 102, 122–124 CAS.
- J. J. Thiele, C. Schroeter, S. N. Hsieh, M. Podda and L. Packer, The antioxidant network of the stratum corneum, Curr. Prob. Dermatol., 2001, 29, 26–42 CrossRef CAS.
- Y. Shindo and T. Hashimoto, Time course of changes in antioxidant enzymes in human skin fibroblasts after UVA irradiation, J. Dermatol. Sci., 1997, 14, 225–232 CrossRef CAS.
- K. Okada, Y. Takahashi, K. Ohnishi, O. Ishikawa and Y. Miyachi, Time-dependent effect of chronic UV irradiation on superoxide dismutase and catalase activity in hairless mice skin, J. Dermatol. Sci., 1994, 8, 183–186 CrossRef CAS.
- A. Poswig, J. Wenk, P. Brenneisen, M. Wlaschek, C. Hommel, G. Quel, K. Faisst, J. Dissemond, K. Briviba, T. Krieg and K. Scharffetter-Kochanek, Adaptive antioxidant response of manganese-superoxide dismutase following repetitive UVA irradiation, J. Invest. Dermatol., 1999, 112, 13–18 CrossRef CAS.
- C. Meewes, P. Brenneisen, J. Wenk, L. Kuhr, W. Ma, J. Alikoski, A. Poswig, T. Krieg and K. Scharffetter-Kochanek, Adaptive antioxidant response protects dermal fibroblasts from UVA-induced phototoxicity, Free Radical Biol. Med., 2001, 30, 238–247 CrossRef CAS.
- G. C. Cheng, P. C. Schulze, R. T. Lee, J. Sylvan, B. R. Zetter and H. Huang, Oxidative stress and thioredoxin-interacting protein promote intravasation of melanoma cells, Exp. Cell Res., 2004, 300, 297–307 CrossRef CAS.
- F. Ogawa, C. S. Sander, A. Hansel, W. Oehrl, H. Kasperczyk, P. Elsner, K. Shimizu, S. H. Heinemann and J. J. Thiele, The repair enzyme peptide methionine-S-sulfoxide reductase is expressed in human epidermis and upregulated by UVA radiation, J. Invest. Dermatol., 2006, 126, 1128–1134 CrossRef CAS.
- M. Heckmann, B. Eberlein-Konig, A. Wollenberg, B. Przybilla and G. Plewig, Ultraviolet-A radiation induces adhesion molecule expression on human dermal microvascular endothelial cells, Br. J. Dermatol., 1994, 131, 311–318 CrossRef CAS.
- M. Heckmann, M. Pirthauer and G. Plewig, Adhesion of leukocytes to dermal endothelial cells is induced after single-dose, but reduced after repeated doses of UVA, J. Invest. Dermatol., 1997, 109, 710–715 CAS.
- G. Treina, C. Scaletta, A. Fourtanier, S. Seite, E. Frenk and L. A. Applegate, Expression of intercellular adhesion molecule-1 in UVA-irradiated human skin cells in vitro and in vivo, Br. J. Dermatol., 1996, 135, 241–247 CrossRef CAS.
- J. Sondergaard, H. Bisgaard and S. Thorsen, Eicosanoids in skin UV inflammation, Photodermatology, 1985, 2, 359–366 CAS.
- A. Mahns, R. Wolber, F. Stab, L. O. Klotz and H. Sies, Contribution of UVB and UVA to UV-dependent stimulation of cyclooxygenase-2 expression in artificial epidermis, Photochem. Photobiol. Sci., 2004, 3, 257–262 CAS.
- M. A. Bachelor, A. L. Silvers and G. T. Bowden, The role of p38 in UVA-induced cyclooxygenase-2 expression in the human keratinocyte cell line, HaCaT, Oncogene, 2002, 21, 7092–7099 CrossRef CAS.
- G. J. Fisher, S. C. Datta, H. S. Talwar, Z. Q. Wang, J. Varani, S. Kang and J. J. Voorhees, Molecular basis of sun-induced premature skin ageing and retinoid antagonism, Nature, 1996, 379, 335–339 CrossRef CAS.
- G. J. Fisher, Z. Q. Wang, S. C. Datta, J. Varani, S. Kang and J. J. Voorhees, Pathophysiology of premature skin aging induced by ultraviolet light, N. Engl. J. Med., 1997, 337, 1419–1428 CrossRef CAS.
- K. Scharffetter, M. Wlaschek, A. Hogg, K. Bolsen, A. Schothorst, G. Goerz, T. Krieg and G. Plewig, UVA irradiation induces collagenase in human dermal fibroblasts in vitro and in vivo, Arch. Dermatol. Res., 1991, 283, 506–511 CrossRef CAS.
- M. J. Petersen, C. Hansen and S. Craig, Ultraviolet A irradiation stimulates collagenase production in cultured human fibroblasts, J. Invest. Dermatol., 1992, 99, 440–444 CAS.
- M. C. Ramos, H. Steinbrenner, D. Stuhlmann, H. Sies and P. Brenneisen, Induction of MMP-10 and MMP-1 in a squamous cell carcinoma cell line by ultraviolet radiation, Biol. Chem., 2004, 385, 75–86 CrossRef CAS.
- K. Wertz, N. Seifert, P. B. Hunziker, G. Riss, A. Wyss, C. Lankin and R. Goralczyk, Beta-carotene inhibits UVA-induced matrix metalloprotease 1 and 10 expression in keratinocytes by a singlet oxygen-dependent mechanism, Free Radical Biol. Med., 2004, 37, 654–670 CrossRef CAS.
- K. Wertz, P. B. Hunziker, N. Seifert, G. Riss, M. Neeb, G. Steiner, W. Hunziker and R. Goralczyk, beta-Carotene interferes with ultraviolet light A-induced gene expression by multiple pathways, J. Invest. Dermatol., 2005, 124, 428–434 CrossRef CAS.
- M. Wlaschek, G. Heinen, A. Poswig, A. Schwarz, T. Krieg and K. Scharffetter-Kochanek, UVA-induced autocrine stimulation of fibroblast-derived collagenase/MMP-1 by interrelated loops of interleukin-1 and interleukin-6, Photochem. Photobiol., 1994, 59, 550–556 CrossRef CAS.
- P. Brenneisen, M. Wlaschek, E. Schwamborn, L. A. Schneider, W. Ma, H. Sies and K. Scharffetter-Kochanek, Activation of protein kinase CK2 is an early step in the ultraviolet B-mediated increase in interstitial collagenase (matrix metalloproteinase-1; MMP-1) and stromelysin-1 (MMP-3) protein levels in human dermal fibroblasts, Biochem. J., 2002, 365, 31–40 CrossRef CAS.
- J. Wenk, J. Schuller, C. Hinrichs, T. Syrovets, N. Azoitei, M. Podda, M. Wlaschek, P. Brenneisen, L. A. Schneider, A. Sabiwalsky, T. Peters, S. Sulyok, J. Dissemond, M. Schauen, T. Krieg, T. Wirth, T. Simmet and K. Scharffetter-Kochanek, Overexpression of phospholipid-hydroperoxide glutathione peroxidase in human dermal fibroblasts abrogates UVA irradiation-induced expression of interstitial collagenase/matrix metalloproteinase-1 by suppression of phosphatidylcholine hydroperoxide-mediated NFkappaB activation and interleukin-6 release, J. Biol. Chem., 2004, 279, 45634–45642 CrossRef CAS.
- T. Polte and R. M. Tyrrell, Involvement of lipid peroxidation and organic peroxides in UVA-induced matrix metalloproteinase-1 expression, Free Radical Biol. Med., 2004, 36, 1566–1574 CrossRef CAS.
- H. Watanabe, T. Shimizu, J. Nishihira, R. Abe, T. Nakayama, M. Taniguchi, H. Sabe, T. Ishibashi and H. Shimizu, Ultraviolet A-induced production of matrix metalloproteinase-1 is mediated by macrophage migration inhibitory factor (MIF) in human dermal fibroblasts, J. Biol. Chem., 2003, 279, 1676–1683 CrossRef.
- A. Honda, R. Abe, T. Makino, O. Norisugi, Y. Fujita, H. Watanabe, J. Nishihira, Y. Iwakura, S. Yamagishi, H. Shimizu and T. Shimizu, Interleukin-1beta and macrophage migration inhibitory factor (MIF) in dermal fibroblasts mediate UVA-induced matrix metalloproteinase-1 expression, J. Dermatol. Sci., 2008, 49, 63–72 CrossRef CAS.
- N. Buechner, P. Schroeder, S. Jakob, K. Kunze, T. Maresch, C. Calles, J. Krutmann and J. Haendeler, Changes of MMP-1 and collagen type Ialpha1 by UVA, UVB and IRA are differentially regulated by Trx-1, Exp. Gerontol., 2008, 43, 633–637 CrossRef CAS.
- S. Koch, C. M. Volkmar, V. Kolb-Bachofen, H. G. Korth, M. Kirsch, A. H. Horn, H. Sticht, N. Pallua and C. V. Suschek, A new redox-dependent mechanism of MMP-1 activity
control comprising reduced low-molecular-weight thiols and oxidizing radicals, J. Mol. Med., 2008, 87, 261–272 CrossRef.
- E. Naru, T. Suzuki, M. Moriyama, K. Inomata, A. Hayashi, K. Arakane and K. Kaji, Functional changes induced by chronic UVA irradiation to cultured human dermal fibroblasts, Br. J. Dermatol., 2005, 153(s2), 6–12 CrossRef CAS.
- H. Tanaka, Y. Murakami, I. Ishii and S. Nakata, Involvement of a forkhead transcription factor, FOXO1A, in UV-induced changes of collagen metabolism, J. Invest. Dermatol. Symp. Proc., 2009, 14, 60–62 CrossRef CAS.
- Y. Minami, K. Kawabata, Y. Kubo, S. Arase, K. Hirasaka, T. Nikawa, N. Bando, Y. Kawai and J. Terao, Peroxidized cholesterol-induced matrix metalloproteinase-9 activation and its suppression by dietary beta-carotene in photoaging of hairless mouse skin, J. Nutr. Biochem., 2009, 20, 389–398 CrossRef CAS.
- B. Catalgol, I. Ziaja, N. Breusing, T. Jung, A. Hohn, B. Alpertunga, P. Schroeder, N. Chondrogianni, E. S. Gonos, I. Petropoulos, B. Friguet, L. O. Klotz, J. Krutmann and T. Grune, The proteasome is an integral part of solar ultraviolet a radiation-induced gene expression, J. Biol. Chem., 2009, 284, 30076–30086 CrossRef CAS.
- C. Kut, W. Hornebeck, N. Groult, G. Redziniack, G. Godeau and B. Pellat, Influence of successive and combined ultraviolet A and B irradiations on matrix metalloelastases produced by human dermal fibroblasts in culture, Cell Biol. Int., 1997, 21, 347–352 CrossRef CAS.
- J. H. Oh, A. S. Chung, H. Steinbrenner, H. Sies and P. Brenneisen, Thioredoxin secreted upon ultraviolet A irradiation modulates activities of matrix metalloproteinase-2 and tissue inhibitor of metalloproteinase-2 in human dermal fibroblasts, Arch. Biochem. Biophys., 2004, 423, 218–226 CrossRef CAS.
- H. Steinbrenner, M. C. Ramos, D. Stuhlmann, H. Sies and P. Brenneisen, UVA-mediated downregulation of MMP-2 and MMP-9 in human epidermal keratinocytes, Biochem. Biophys. Res. Commun., 2003, 308, 486–491 CrossRef CAS.
- S. Onoue, T. Kobayashi, Y. Takemoto, I. Sasaki and H. Shinkai, Induction of matrix metalloproteinase-9 secretion from human keratinocytes in culture by ultraviolet B irradiation, J. Dermatol. Sci., 2003, 33, 105–111 CrossRef CAS.
- Y. Y. He, J. Pi, J. L. Huang, B. A. Diwan, M. P. Waalkes and C. F. Chignell, Chronic UVA irradiation of human HaCaT keratinocytes induces malignant transformation associated with acquired apoptotic resistance, Oncogene, 2006, 25, 3680–3688 CrossRef CAS.
- J. H. Cauchard, A. Robinet, S. Poitevin, H. Bobichon, J. C. Maziere, G. Bellon and W. Hornebeck, UVA-mediated down-regulation of MMP-2 and MT1-MMP coincides with impaired angiogenic phenotype of human dermal endothelial cells, Biochem. Biophys. Res. Commun., 2006, 345, 681–687 CrossRef CAS.
- I. Sjerobabski Masnec and S. Poduje, Photoaging, Coll. Antropol., 2008, 32(Suppl 2), 177–180 Search PubMed.
- K. A. Codriansky, M. J. Quintanilla-Dieck, S. Gan, M. Keady, J. Bhawan and T. M. Runger, Intracellular degradation of elastin by cathepsin K in skin fibroblasts–a possible role in photoaging, Photochem. Photobiol., 2009, 85, 1356–1363 CrossRef CAS.
- S. D. Lamore, S. Qiao, D. Horn and G. T. Wondrak, Proteomic identification of cathepsin B and nucleophosmin as novel UVA-targets in human skin fibroblasts, Photochem. Photobiol., 2010, 86, 1307–1317 CrossRef CAS.
- A. Klose, A. Wilbrand-Hennes, J. Brinckmann and N. Hunzelmann, Alternate trafficking of cathepsin L in dermal fibroblasts induced by UVA radiation, Exp. Dermatol., 2009, 19, e117–123 CrossRef.
- H. Hernandez-Pigeon, C. Jean, A. Charruyer, M. J. Haure, C. Baudouin, M. Charveron, A. Quillet-Mary and G. Laurent, UVA induces granzyme B in human keratinocytes through MIF: implication in extracellular matrix remodeling, J. Biol. Chem., 2007, 282, 8157–8164 CrossRef CAS.
- R. Pastila and D. Leszczynski, Ultraviolet A exposure alters adhesive properties of mouse melanoma cells, Photodermatol., Photoimmunol. Photomed., 2005, 21, 234–241 CrossRef CAS.
- R. Pastila and D. Leszczynski, Ultraviolet A exposure might increase metastasis of mouse melanoma: a pilot study, Photodermatol., Photoimmunol. Photomed., 2005, 21, 183–190 CrossRef.
- A. van Schanke, M. J. Jongsma, R. Bisschop, G. M. van Venrooij, H. Rebel and F. R. de Gruijl, Single UVB overexposure stimulates melanocyte proliferation in murine skin, in contrast to fractionated or UVA-1 exposure, J. Invest. Dermatol., 2005, 124, 241–247 CrossRef CAS.
- H. Zhang and I. Rosdahl, Ultraviolet A and B differently induce intracellular protein expression in human skin melanocytes–a speculation of separate pathways in initiation of melanoma, Carcinogenesis, 2003, 24, 1929–1934 CrossRef CAS.
- M. A. Grimbaldeston, C. L. Geczy, N. Tedla, J. J. Finlay-Jones and P. H. Hart, S100A8 induction in keratinocytes by ultraviolet A irradiation is dependent on reactive oxygen intermediates, J. Invest. Dermatol., 2003, 121, 1168–1174 CrossRef CAS.
- F. Breuckmann, C. Appelhans, A. Bastian, M. Stuecker, P. Altmeyer and A. Kreuter, UVA1-induced decrease in dermal neuron-specific enolase (NSE) in acrosclerosis, Arch. Dermatol. Res., 2004, 296, 182–184 CrossRef CAS.
- M. C. Polderman, S. le Cessie, T. W. Huizinga and S. Pavel, Efficacy of UVA-1 cold light as an adjuvant therapy for systemic lupus erythematosus, Rheumatology, 2004, 43, 1402–1404 CrossRef CAS.
- A. Aroun, J. L. Zhong, R. M. Tyrrell and C. Pourzand, Iron, oxidative stress and the example of solar ultraviolet A radiation, Photochem. Photobiol. Sci. 10.1039/c1pp05204g.
- J. Zhang and G. T. Bowden, Activation of p38 MAP kinase and JNK pathways by UVA irradiation, Photochem. Photobiol. Sci. 10.1039/c1pp05133d.
- S. D. Lamore and G. T. Wondrak, Autophagic-lysosomal dysregulation downstream of cathepsin B inactivation in human skin fibroblasts exposed to UVA, Photochem. Photobiol. Sci, 10.1039/c1pp05131h.
Footnote |
† Contribution to the themed issue on the biology of UVA. |
|
This journal is © The Royal Society of Chemistry and Owner Societies 2012 |
Click here to see how this site uses Cookies. View our privacy policy here.