A simple method for the preparation of 3-hydroxyiminodehydroquinate,† a potent inhibitor of type II dehydroquinase
Received (in Cambridge, UK) 24th June 2002, Accepted 24th July 2002
First published on 16th August 2002
Abstract
A number of routes to 3-hydroxyiminodehydroquinate 4, one of the most potent inhibitors of type II dehydroquinase that is currently known, have been investigated. Methods based on the existing literature synthesis, i.e. oxime formation of a suitably C-4 and C-5 protected methyl 3-dehydroquinate derivative were initially studied. Benzoyl protection as in 11 did give the desired product but in low overall yield. An alternative BBA protection strategy starting with 7 was successful in generating a C-4/C-5 analogue of the desired oxime 4 in high yield. Further investigation revealed that it was unnecessary to protect the dehydroquinate precursor, hence the potassium salt corresponding to oxime 4 was simply synthesised as a single isomer from methyl dehydroquinate 10.
Introduction
Types I and II dehydroquinase catalyse the dehydration of 3-dehydroquinate 1 to 3-dehydroshikimate 3. The type II enzyme operates in the catabolic quinate pathway, thus allowing fungi to use quinic acid as a carbon source (Scheme 1).1 By contrast, both types I and II enzymes are utilised in the shikimate pathway—the biosynthetic pathway by which aromatic amino acids and essential aromatic compounds such as folate and ubiquinone are produced in nature.2 The shikimate pathway is only present in plants, fungi and bacteria, but not in mammals. A very exciting and recent development is the recognition that the shikimate pathway plays an important role in the apicomplexin parasites such as Toxoplasma gondii, Plasmodium falciparum
(malaria), and Cryptosporidium parvum, and that inhibition of the pathway can retard parasitic growth.3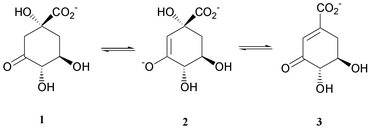 |
| Scheme 1 | |
These facts, together with the recent determination of crystal structures of the dehydroquinases4 highlight the need to develop inhibitors of these enzymes as new and novel antibiotic and herbicide leads. One such compound, oxime 4, has recently been shown to be selective for type II dehydroquinases over type I and to exhibit selectivity amongst the type II enzymes, being more potent against the enzymes from the bacterium M. tuberculosis and the fungi A. nidulans, than the enzyme from the bacterium S. coelicolor.5 It has been suggested that the type selectivity of 4 is a result of positioning of the oxime functionality into the binding pocket of the type II enzyme that normally6 stabilises the developing negative charge of a biosynthetic enolate intermediate 2.5 By contrast, the type I enzyme operates via a mechanism involving the formation of a series of imine–enamine intermediates7,8 rather than via an enolate intermediate. The stabilising effect of the oxime inhibitor is therefore not possible in this case. This potential mechanistic basis for the selectivity and potency of 4 towards the dehydroquinases provides a basis for further inhibitor design. Accordingly, a convenient and reliable method for the synthesis of this deceptively simple molecule, and its analogues, is required.
Oxime 4 has previously been prepared as the sodium salt from quinic acid, in eight steps and 6–9% overall yield.5 This preparation requires a lengthy and tedious series of protections and deprotections of quinic acid to allow selective functionalisation at C-3.9 It also gives rise to mixtures of isomers at two key steps which contributes to the low overall yield. Herein we describe an efficient and simple method for the synthesis of this oxime from 3-dehydroquinic acid 6
(Scheme 3). This synthetic route was made possible by our recently published10 synthesis of 6 from quinic acid 5
(see Scheme 2, 53% overall yield). Analogues with C-4 and C-5 protection have also been prepared (Scheme 3).
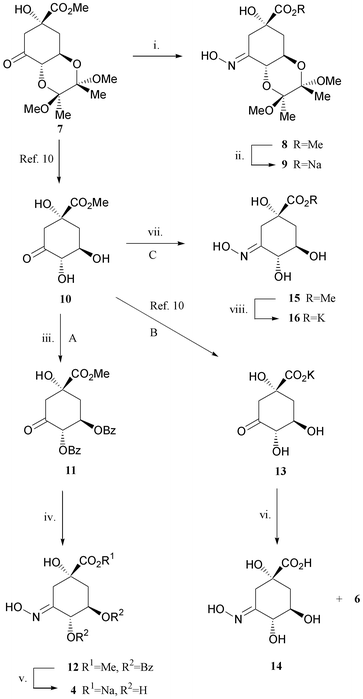 |
| Scheme 2 | |
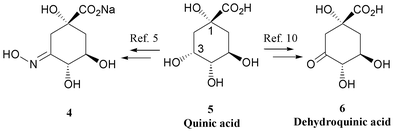 |
| Scheme 3 i. H2NOH·HCl, NaOAc, 92%; ii. NaOH, H2O, 98%; iii. BzCl, pyridine, 8%; iv. H2NOH·HCl, NaOAc; v. NaOH, H2O; vi. H2NOH·HCl; vii. H2NOH·HCl, NaOAc, 96%, viii. KOH, H2O, 97%. | |
Results and discussion
A number of alternative approaches to 4 were investigated (see Scheme 3). Initially, we attempted to simplify the published route by using methyl 3-dehydroquinate bearing a protecting group for the hydroxy groups at C-4 and C-5 as the starting material for the introduction of the oxime functionality at C-3. The butane 2,3-bisacetal (BBA) protected11 dehydroquinate 7, available in our laboratory as a precursor to dehydroquinic acid 6,10 was simply treated with hydroxylamine hydrochloride in the presence of sodium acetate to afford the oxime 8 in 92% yield and as a single isomer (Scheme 3). The methyl ester of 8 was carefully hydrolysed using sodium hydroxide at room temperature to afford the oxime as the sodium salt 9 which gave satisfactory characterisation data. While this sequence provided access to a useful dehydroquinate-based oxime, it did not allow preparation of the desired oxime 4 since the conditions for removing the protecting group are not compatible with the oxime functionality.‡Next we investigated three routes to the oxime 4 starting from methyl 3-dehydroquinate 10, itself derived from 7 by deprotection of the BBA group10
(see A, B and C, Scheme 3). The first of these methods involved protecting the C-4 and C-5 hydroxy groups of 10 with a base-labile protecting group. This alternative to the BBA protection (see 7 above) allows concomitant removal of all protecting groups under conditions that are compatible with the oxime functionality. Consequently, methyl dehydroquinate 10 was treated with pyridine and benzoyl chloride following conditions developed by Mercier et al. for the esterification of methyl quinate.12 The desired dibenzoyl ester 11 was isolated in a low 8% yield after column chromatography and crystallisation.§ A further two fractions were obtained that contained complex mixtures of benzoylated dehydroquinates and dehydroshikimates. Despite the low yield of 11, a sufficient quantity was obtained to investigate oxime formation. Dehydroquinate 11 was reacted with hydroxylamine hydrochloride and sodium hydroxide to give the oxime 12 which was not purified. This sample was then saponified with sodium hydroxide to give the desired oxime salt 4 in a mixture with sodium benzoate and sodium acetate. Further purification was not attempted since parallel studies using the unprotected dehydroquinates proved more successful.
In the next method, hydrolysis of 10 gave potassium dehydroquinate 1310 which was then reacted directly with hydroxylamine hydrochloride. It was thought that the carboxylate functionality of 13 would act as an internal base to liberate free hydroxylamine from its hydrochloride salt and hence minimise the likelihood of forming dehydroshikimate derivatives via dehydration at C-1. To this end, 13 was treated with one equivalent of hydroxylamine hydrochloride. The reaction afforded an inseparable mixture of the oxime 14 and dehydroquinic acid 6
(9 ∶ 1 by 1H-NMR). Although it is possible that this reaction could be completed with more careful control of pH, it was thought more expedient to simply perform the saponification after the conversion to the oxime. Hence, in the final and successful method, methyl dehydroquinate 10 was treated with hydroxylamine hydrochloride (1 equiv.) and sodium acetate (1 equiv.). In this case the oxime 15 was obtained as a single isomer (97%) that was fully characterised. The methyl ester of 15 was then carefully hydrolysed using potassium hydroxide to give the desired potassium 3-hydroxyiminodehydroquinate 16
(97%) which gave essentially equivalent data to 4.5
Conclusion
The hydroxyiminodehydroquinate 16 has been synthesised in five steps from quinic acid 5 in 50% yield. A C-4/C-5 analogue 9 has also been prepared in excellent overall yield. The method presented provides a simple and inexpensive route to this important class of dehydroquinase inhibitor.Experimental
General
1H-NMR spectra were obtained using a Varian Unity 300 NMR spectrometer or a Varian Inova 500 spectrometer at 300 and 500 MHz respectively. 13C-NMR spectra were recorded at a frequency of 75 MHz on a Varian Unity 300 spectrometer. 1H–13C-NMR Correlation experiments were carried out on a Varian Inova 500 spectrometer. Chemical shifts (δ) are given in parts per million (ppm). Mass spectra were recorded on a Kratos MS80RFA instrument for electron impact (EI) technique, or a Micromass LCT spectrometer for the time-of-flight (TOF) method. Infrared spectra were obtained on a Shimadzu Hyper FT-IR instrument. Optical rotations were measured using a Perkin 341 spectrometer. Melting points were taken on an Electrothermal® apparatus and are uncorrected.Methyl (1S,3E,4R,5R)-4,5-[(2S,3S)-2,3-dimethoxybutane-2,3-diyldioxy)]-1-hydroxy-3-(hydroxyimino)cyclohexane-1-carboxylate 8
To a solution of protected dehydroquinic acid 710
(100 mg, 0.31 mmol) in methanol (1 mL) were added hydroxylamine hydrochloride (26 mg, 0.37 mmol) and sodium acetate (60 mg, 0.4 mmol) and the mixture was left to stir at room temperature for 15 hours. Methanol was removed under reduced pressure, and the residue was partitioned between ethyl acetate (15 mL) and water (15 mL). The organic layer was separated, and the aqueous layer was extracted with ethyl acetate (3 × 30 mL). The combined organic layer was dried over magnesium sulfate, then concentrated in vacuo to afford the oxime 8 as a glass (95 mg, 92%). Mp 117–120 °C; [α]20D
+88.5 (c 0.47 in CHCl3)
(Found C, 49.06, H, 7.08, N, 3.90, C14H23O8N·½H2O requires C, 49.10, H, 7.08, N, 4.08%); νmax(KBr)/cm−1 3340, 2954, 1738, 1450, 1379; δH
(CDCl3, 300 MHz) 1.16 (3H, s, 2′-CH3 or 3′-CH3), 1.19 (3H, s, 2′-CH3 or 3′-CH3), 1.96 (2H, m, 6-H2), 2.11 (1H, d, J 15, 2-HH), 3.16 (3H, s, OCH3), 3.41 (3H, s, OCH3), 3.45 (1H, dd, J 15, 2, 2-HH), 3.72 (3H, s, CO2CH3), 4.02 (1H, ddd, J 10, 10, 5.5, 5-H), 4.18 (1H, d, J 10, 4-H); δC
(CDCl3, 75 MHz) 17.1 (2′-CH3 or 3′-CH3), 17.3 (2′-CH3 or 3′-CH3), 32.8 (C-2), 36.8 (C-6), 47.4 (2′-OCH3 or 3′-OCH3), 47.7 (2′-OCH3 or 3′-OCH3), 52.8 (CO2CH3), 67.0 (C-5), 71.3 (C-4), 73.6 (C-1), 99.2 (C-2′ or C-3′), 99.7 (C-2′ or C-3′), 149.5 (C-3), 174.3 (CO2CH3); m/z
(EI) 302 (M+
− OCH3, 7%), 242 (18), 167 (93), 151 (41), 101 (100), 75 (50).Sodium (1S,3E,4R,5R)-4,5-[(2S,3S)-2,3-dimethoxybutane-2,3-diyldioxy)]-1-hydroxy-3-(hydroxyimino)cyclohexane-1-carboxylate 9
A solution of oxime 8
(300 mg, 0.90 mmol) in diethyl ether (20 mL) was treated with an aqueous solution of sodium hydroxide (2.5 M, 0.65 mL). The reaction mixture was stirred vigorously at room temperature for 5 min. The water was evaporated in vacuo to afford the crude product that was recrystallised from ethanol to give oxime 9 as white plates (302 mg, 98%). Mp 122–124 °C; [α]20D
+51.4 (c 1.03 in H2O)
(Found C, 39.50, N, 3.25, C13H23O8NNa·3H2O requires C, 39.50, N, 3.54%); νmax(KBr)/cm−1 3327, 2949, 1596, 1386, 1130, 1031; δH
(D2O, 300 MHz) 1.22 (3H, s, 2′-CH3 or 3′-CH3), 1.27 (3H, s, 2′-CH3 or 3′-CH3), 1.80 (1H, m, 6-HH), 1.90 (1H, dd, J 13, 11.5, 6-HH), 2.19 (1H, d, J 15, 2-HH), 3.15 (3H, s, 2′-OCH3 or 3′-OCH3), 3.18 (3H, s, 2′-OCH3 or 3′-OCH3), 3.24 (1H, dd, J 15, 2.5, 2-HH), 3.59 (1H, m, 5-H), 4.28 (1H, d, J 10.5, 4-H); δC
(D2O, 75 MHz) 17.1 (2′-CH3 or 3′-CH3), 17.3 (2′-CH3 or 3′-CH3), 32.8 (C-2), 36.8 (C-6), 47.4 (2′-OCH3 or 3′-OCH3), 47.7 (2′-OCH3 or 3′-OCH3), 52.8 (CO2CH3), 67.0 (C-5), 71.3 (C-4), 73.6 (C-1), 99.2 (C-2′ or C-3′), 99.7 (C-2′ or C-3′), 149.5 (C-3), 174.3 (CO2CH3); m/z
(TOF) found 318.1197, C13H20O8N requires 318.1189; 318 (M − Na+, 100%), 305 (34).Methyl (1R,4S,5R)-4,5-bis(benzoyloxy)-1-hydroxy-3-oxocyclohexane-1-carboxylate 11
A solution of methyl dehydroquinate 1010
(122 mg, 0.60 mmol) in acetone (1 mL) was cooled to −10 °C and then treated with triethylamine (2 mL). After 5 min, benzoyl chloride (0.28 mL, 330 mg, 2.4 mmol) was added dropwise and the mixture was left to stir for 2 h at which time the reaction was quenched by addition of aqueous hydrochloric acid (0.1 M, 15 mL). The resulting crude mixture was extracted with ethyl acetate (3 × 30 mL). The combined organic phases were washed with brine (50 mL), dried over magnesium sulfate, and concentrated under reduced pressure. The crude mixture was purified by column chromatography using 40% ethyl acetate in petroleum ether to give the dibenzoylated compound 11 as a colourless solid which was recrystallised from diethyl ether (20 mg, 8%). Mp 58–60 °C; [α]20D
−94.8 (c 1.8 in CH2Cl2) (Found C,64.07, H, 4.89, C22H20O8.H2O requires C, 64.07, H, 4.89%); νmax(KBr)/cm−1 3479, 1728, 1600, 1452, 1282, 1253, 1070; δH
(CDCl3, 300 MHz) 2.55 (1H, m, 6-HH), 2.68–2.81 (2H, m, 6-HH and 2-HH), 3.13 (1H, d, J 14, 2-HH), 3.85 (3H, s, CO2CH3), 5.86–5.97 (2H, m, 4-H and 5-H), 7.35–7.58 (6H, m, ar H × 6), 7.95–8.22 (4H, m, ar H × 4); δC
(CDCl3, 75 MHz) 38.3 (C-6), 48.6 (C-2), 53.7 (OCH3), 70.6 (C-5), 73.4 (C-1), 78.9 (C-4), 128.4–133.4 (ar C × 12), 165.3 (4-CO2Ph or 5-CO2Ph), 165.7 (4-CO2Ph or 5-CO2Ph), 196.6 (C-3).Sodium (1S,3E,4R,5R)-1,4,5-trihydroxy-3-(hydroxyimino)cyclohexane-1-carboxylate 4
To a solution of triester 11
(120 mg, 0.30 mmol) in water and methanol (1 ∶ 2, 4 mL) were added sodium acetate trihydrate (180 mg, 1.32 mmol) and hydroxylamine hydrochloride (85 mg, 1.22 mmol). The mixture was left to stir overnight at room temperature. The methanol was evaporated under reduced pressure, and the aqueous layer was extracted with ethyl acetate (3 × 15 mL). The combined organic phases were dried over magnesium sulfate, then evaporated in vacuo to give the oxime 12
(124 mg) which was not purified further. δH
(CDCl3, 300 MHz) 2.35 (1H, dd, J 13, 11, 6-HH), 2.52 (1H, d, J 15, 2-HH), 2.51–2.58 (1H, m, 6-HH), 3.63 (1H, dd, J 15, 3, 2-HH), 3.82 (3H, s, CO2CH3), 5.80 (1H, dt, J 10.5, 9.5, 5-H), 5.93 (1H, d, J 9.5, 4-H); δC
(CDCl3, 75 MHz) 33.0 (C-2), 38.1 (C-6), 53.4 (CO2CH3), 71.1 (C-5), 72.9 (C-4), 73.3 (C-1), 128.1-133.3 (ar C × 12), 149.9 (C-3), 165.4 (4-CO2Ph or 5-CO2Ph), 165.7 (4-CO2Ph or 5-CO2Ph), 174.1 (CO2CH3); m/z
(TOF) found 428.1345 (C22H21O8N requires 428.1345), 428 (MH+, 100%), 413 (25).A solution of oxime 12
(100 mg, 0.23 mmol) in diethyl ether (2 mL) was treated with an aqueous solution of sodium hydroxide (2.5 M, 0.3 mL, 0.75 mmol) and stirred vigorously for 5 min. The reaction mixture was evaporated to dryness in vacuo to give the desired oxime 4 in a mixture with sodium benzoate and sodium acetate as an off-white solid (110 mg, 4.5 ∶ 4.5 ∶ 1 by 1H-NMR). Selected data for oxime 4:5δH
(D2O, 300 MHz) 1.90 (2H, m, 6-H2), 2.12 (1H, d, J 15, 2-HH), 3.12 (1H, J 15, 2, 2-HH), 3.66 (1H, ddd, J 10, 9, 5.5, 5-H), 3.97 (1H, d, J 9, 4-H).
(1S,3E,4R)-1,4,5-Trihydroxy-3-(hydroxyimino)cyclohexane-1-carboxylic acid 14
To a solution of potassium dehydroquinate 1310
(122 mg, 0.53 mmol) in water (1 mL) was added hydroxylamine hydrochloride (37 mg, 0.53 mmol) and the mixture was left to stir for 3 h at room temperature. The solvent was removed in vacuo to afford an inseparable mixture of the oxime 14 and dehydroquinic acid 6 and potassium chloride as a white hygroscopic solid (155 mg, 9 ∶ 1 by 1H-NMR). Selected data for oxime 14: νmax(KBr)/cm−1 3400, 1716, 1651, 1226; δH
(D2O, 300 MHz) 1.93 (1H, dd, J 13.0, 11.5, 6-HH), 2.07 (1H, br m, 6-HH), 2.24 (1H, d, J 14.5, 2-HH), 3.30 (1H, dd, J 14.5, 1.5, 2-HH), 3.69 (1H, br m, 5-H), 4.04 (1H, d, J 9.5, 4-H); δC
(D2O, 75 MHz) 31.9 (C-2), 39.4 (C-6), 71.1 (C-5), 74.0 (C-1), 74.6 (C-4), 156.4 (C-3), 177.4 (CO2H). Dehydroquinic acid 6:10δH
(D2O, 300 MHz) 2.20 (1H, m, 6-H2), 2.46 (1H, br d, J 14, 2-HH), 3.02 (1H, d, J 14, 2-HH), 3.79 (1H, m, 5-H), 4.18 (1H, d, J 9.5, 4-H); δC
(D2O, 75 MHz) 39.4 (C-6), 47.6 (C-2), 71.4 (C-5), 74.0 (C-1), 80.6 (C-4), 177.4 (CO2H), (C-3 not observed).Methyl (1S,3E,4R,5R)-3-(hydroxyimino)-1,4,5-trihydroxycyclohexane-1-carboxylate 15
A solution of methyl dehydroquinate 1010
(230 mg, 1.13 mmol) in water (2 mL) was added to sodium acetate trihydrate (153 mg, 1.13 mmol) and hydroxylamine hydrochloride (83 mg, 1.13 mmol). The mixture was left stirring at room temperature for 1.5 h, before it was concentrated under reduced pressure. Methanol (3 mL) was added to the residue, and the insoluble residue of sodium chloride was filtered off over Celite. The filtrate was evaporated under reduced pressure to give a thick oil which was dried under high vacuum to afford the oxime 15 as a very hygroscopic white solid (250 mg, 96%). Mp 99–100 °C; [α]20D
−25.5 (c 1.06 in MeOH)
(Found C, 43.08, H, 6.01, N, 6.02, C8H13O6N·¼H2O requires C, 42.94, H, 6.08, N, 6.25%); νmax(KBr)/cm−1 3415, 2856, 2341, 1732, 1633, 1373, 1087; δH
(acetone-d6, 500 MHz) 1.88 (1H, m, 6-HH), 2.10 (1H, ddd, J 13.5, 5, 2.5, 6-HH), 2.24 (1H, d, J 15, 2-HH), 3.32 (1H, dd, J 15, 2.5, 2-HH), 3.70 (3H, s, CO2CH3), 3.76 (1H, ddd, J 11, 9, 5, 5-H), 4.08 (1H, d, J 9, 4-H); δC
(D2O, 75 MHz) 32.1 (C-2), 40.1 (C-6), 52.1 (CO2CH3), 71.9 (C-5), 74.1 (C-4), 78.5 (C-1), 154.0 (C-3), 174.8 (CO2CH3); m/z
(TOF) found 220.0821, C8H14NO requires 220.0821; 220 (MH+, 100).Potassium (1S,3E,4R,5R)-1,4,5-trihydroxy-3-(hydroxyimino)cyclohexane-1-carboxylate 16
A solution of the methyl ester 15
(115 mg, 0.52 mmol) in water (2 mL) was cooled to 0 °C and then treated with a solution of potassium hydroxide (29.2 mg, 0.52 mmol) in water (1.2 mL). The ice bath was removed and the mixture was left stirring and warming to room temperature for 5 min. The mixture was then concentrated under reduced pressure to give the desired potassium salt of the oxime 16 as a pale yellow hygroscopic glass (122 mg, 97%). [α]20D
−40.3 (c 1.0, H2O); δH
(D2O, 500 MHz) 1.93 (1H, dd, J 13.5, 10.5, 6-HH), 2.0 (1H, ddd, J 13.5, 5, 2.5, 6-HH), 2.23 (1H, d, J 14.5, 2-HH), 3.22 (1H, dd, J 14.5, 2.5, 2-HH), 3.72 (1H, ddd, J 10.5, 9.5, 5, 5-H), 4.07 (1H, d, J 9.5, 4-H); δC
(D2O, 75 MHz) 32.8 (C-2), 40.2 (C-6), 71.8 (C-5), 74.9 (C-4), 75.3 (C-1), 157.3 (C-3), 180.5 (CO2K); m/z
(TOF) found 204.0508, C7H10O6N requires 204.0508; 204 (M − K+, 100%), 186 (22), 126.9 (59).Acknowledgements
We are pleased to acknowledge financial support from a Royal Society of New Zealand Marsden Grant.References
- N. H. Giles, M. E. Case, J. A. Baum, R. F. Geever, L. Huiet, V. B. Patel and B. M. Tyler, Microbiol. Rev., 1985, 49, 338 Search PubMed.
- R. Bentley, CRC Crit. Rev. Biochem., 1990, 25, 307 Search PubMed.
- F. Roberts, C. W. Roberts, J. J. Johnson, D. E. Kyle, T. Krell, J. R. Coggins, G. H. Coombs, W. K. Milhous, S. Tzipori, D. J. P. Ferguson, D. Chakrabarti and R. McLeod, Nature, 1998, 393, 801 CrossRef CAS.
- D. G. Gourley, A. K. Shrive, I. Polikarpov, T. Krell, J. R. Coggins, A. R. Hawkins, N. W. Isaacs and L. Sawyer, Nat. Struct. Biol., 1999, 6, 521 CrossRef CAS.
- M. Frederickson, E. J. Parker, A. R. Hawkins, J. R. Coggins and C. Abell, J. Org. Chem., 1999, 64, 2612 CrossRef CAS.
- J. M. Harris, C. Gonzáles-Bello, C. Kleanthous, A. R. Hawkins, J. R. Coggins and C. Abell, Biochem. J., 1996, 319, 333 CAS.
- A. Shneier, C. Kleanthous, R. Deka, J. R. Coggins and C. Abell, J. Am. Chem. Soc., 1991, 113, 9416 CrossRef.
- S. Chaudhuri, K. Duncan, L. D. Graham and J. R. Coggins, Biochem. J., 1991, 275, 1 CAS.
- M. K. Manthey, C. Gonzáles-Bello and C. Abell, J. Chem. Soc., Perkin Trans. 1, 1997, 625 RSC.
- C. Le Sann, C. Abell and A. D. Abell, Synth. Commun., in the press Search PubMed.
- J.-L. Montchamp, F. Tian, M. E. Hart and J. W. Frost, J. Org. Chem., 1996, 61, 3897 CrossRef CAS.
- D. Mercier, J. Cléophax, J. Hildesheim, A. M. Sépulchre and S. D. Géro, Tetrahedron Lett., 1969, 2497 CrossRef CAS.
- E. Delfourne, P. Despeyroux, L. Gorrichon and J. Véronique, J. Chem. Res. (S), 1991, 56 Search PubMed.
Footnotes |
† The IUPAC name for 3-hydroxyiminodehydroquinate is (1S,4R,5R)-1,4,5-trihydroxy-3-(hydroxyimino)cyclohexane-1-carboxylate. |
‡ Oxime 9 might prove useful for determining the importance of the hydroxy groups at C-4 and C-5 to inhibitory activity. |
§ Delfourne et al.
(see ref. 13) have reported a low 10% yield for the acetylation of methyl dehydroquinate. |
|
This journal is © The Royal Society of Chemistry 2002 |